- Search by keyword
- Search by citation
Page 1 of 127
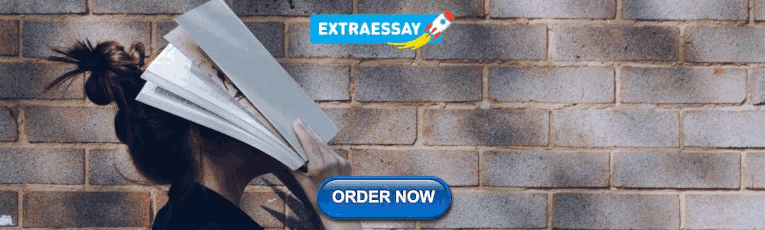
Upregulated synthesis and production of bioactive compounds in Lotus arabicus L. by in vitro feeding with dried powder of date palm seeds
Plants are considered the primary source of many principal bioactive compounds that have been utilized in a wide range of applications including the pharmaceutical and biotechnological industries. Therefore, t...
- View Full Text
Comparative transcriptome and coexpression network analysis reveals key pathways and hub candidate genes associated with sunflower ( Helianthus annuus L. ) drought tolerance
Drought severely limits sunflower production especially at the seedling stage. To investigate the response mechanism of sunflowers to drought stress, we utilized two genotypes of sunflower materials with diffe...
Genome-wide association study for in vitro digestibility and related traits in triticale forage
Triticale is making its way on dairy farms as an alternative forage crop. This requires the availability of high-yielding triticale varieties with good digestibility. Triticale forage breeding mainly focussed ...
Prediction accuracy of genomic estimated breeding values for fruit traits in cultivated tomato ( Solanum lycopersicum L.)
Genomic selection (GS) is an efficient breeding strategy to improve quantitative traits. It is necessary to calculate genomic estimated breeding values (GEBVs) for GS. This study investigated the prediction ac...
Inoculation of heavy metal resistant bacteria alleviated heavy metal-induced oxidative stress biomarkers in spinach ( Spinacia oleracea L.)
Most vegetable crops are severely affected by the uptake of heavy metals from the soil. Heavy metals in vegetable bodies generate reactive oxygen species (ROS) that unbalance the antioxidant defense system. Th...
Mathematical kinetic modelling followed by in vitro and in vivo assays reveal the bifunctional rice GTPCHII/DHBPS enzymes and demonstrate the key roles of OsRibA proteins in the vitamin B2 pathway
Riboflavin is the precursor of several cofactors essential for normal physical and cognitive development, but only plants and some microorganisms can produce it. Humans thus rely on their dietary intake, which...
Integrative physiological, metabolomic, and transcriptomic analysis reveals the drought responses of two apple rootstock cultivars
Drought is considered the main environmental factor restricting apple production and thus the development of the apple industry. Rootstocks play an important role in enhancing the drought tolerance of apple pl...
The activation of iron deficiency responses of grapevine rootstocks is dependent to the availability of the nitrogen forms
In viticulture, iron (Fe) chlorosis is a common abiotic stress that impairs plant development and leads to yield and quality losses. Under low availability of the metal, the applied N form (nitrate and ammoniu...
Effects of nitrate- and ammonium- nitrogen on anatomical and physiological responses of Catalpa bungei under full and partial root-zone drought
Catalpa bungei is a precious timber species distributed in North China where drought often occurs. To clarify adaptive responses of C. bungei to partial- and full- root-zone drought under the influence of nitroge...
Overexpression of OsDUF6 increases salt stress tolerance in rice
Soil salinity is one of the primary environmental stresses faced in rice production. When plants are exposed to salt stress, a series of cellular balances will be disrupted. Dufulin is an immune-induced antivi...
Analysis of the global transcriptome and miRNAome associated with seed dormancy during seed maturation in rice ( Oryza sativa L. cv. Nipponbare)
Seed dormancy is a biological mechanism that prevents germination until favorable conditions for the subsequent generation of plants are encountered. Therefore, this mechanism must be effectively established d...
Investigation of the morphological, physiological, biochemical, and catabolic characteristics and gene expression under drought stress in tolerant and sensitive genotypes of wild barley [ Hordeum vulgare subsp. spontaneum (K. Koch) Asch. & Graebn.]
Barley ( H. vulgare L .) is an important cereal crop cultivated across various climates globally. Barley and its ancestor ( H. vulgare subsp. spontaneum ) are an economically valuable model for genetic research and i...
Genome-wide analysis of the KNOX gene family in Moso bamboo: insights into their role in promoting the rapid shoot growth
KNOTTED1 -like homeobox ( KNOX ) genes, plant-specific homologous box transcription factors (TFs), play a central role in regulating plant growth, development, organ formation, and response to biotic and abiotic str...
Long-term organogenic callus cultivation of Ranunculus illyricus L.: a blueprint for sustainable ex situ conservation of the species in urban greenery
The growing trend of introducing wild plant species into urban environments necessitates the identification of novel species adapted to prevailing conditions. A promising reservoir of such species may be xerot...
Genome-wide identification analysis of the 4-Coumarate: CoA ligase ( 4CL ) gene family expression profiles in Juglans regia and its wild relatives J. Mandshurica resistance and salt stress
Persian walnut ( Juglans regia ) and Manchurian walnut ( Juglans mandshurica ) belong to Juglandaceae, which are vulnerable, temperate deciduous perennial trees with high economical, ecological, and industrial values...
Integrated transcriptome and metabolome analysis reveals anthocyanin biosynthesis mechanisms in pepper ( Capsicum annuum L.) leaves under continuous blue light irradiation
Different metabolic compounds give pepper leaves and fruits their diverse colors. Anthocyanin accumulation is the main cause of the purple color of pepper leaves. The light environment is a critical factor aff...
The role of strigolactone in alleviating salinity stress in chili pepper
Salinity stress can significantly delay plant growth. It can disrupt water and nutrient uptake, reducing crop yields and poor plant health. The use of strigolactone can be an effective technique to overcome th...
Integrating genes and metabolites: unraveling mango's drought resilience mechanisms
Mango ( Mangifera indica L.) faces escalating challenges from increasing drought stress due to erratic climate patterns, threatening yields, and quality. Understanding mango's drought response mechanisms is pivota...
Transcriptome sequencing and expression analysis in peanut reveal the potential mechanism response to Ralstonia solanacearum infection
Bacterial wilt caused by Ralstonia solanacearum severely affects peanut ( Arachis hypogaea L.) yields. The breeding of resistant cultivars is an efficient means of controlling plant diseases. Therefore, identifica...
Deciphering the possible role of RNA-helicase genes mechanism in response to abiotic stresses in rapeseed ( Brassica napus L.)
Plants mediate several defense mechanisms to withstand abiotic stresses. Several gene families respond to stress as well as multiple transcription factors to minimize abiotic stresses without minimizing their ...
Genome-wide characterization of the bHLH gene family in Gynostemma pentaphyllum reveals its potential role in the regulation of gypenoside biosynthesis
Gynostemma pentaphyllum , an ancient Chinese herbal medicine, serves as a natural source of gypenosides with significant medicinal properties. Basic helix-loop-helix (bHLH) transcription factors play pivotal roles...
Evolutionary history of an Irano-Turanian cushion-forming legume ( Onobrychis cornuta )
The Irano-Turanian region is one of the largest floristic regions in the world and harbors a high percentage of endemics, including cushion-like and dwarf-shrubby taxa. Onobrychis cornuta is an important cushion-...
Integrated transcriptomic and metabolomic analyses reveals anthocyanin biosynthesis in leaf coloration of quinoa ( Chenopodium quinoa Willd.)
Quinoa leaves demonstrate a diverse array of colors, offering a potential enhancement to landscape aesthetics and the development of leisure-oriented sightseeing agriculture in semi-arid regions. This study ut...
Comparative plastome analysis of the sister genera Ceratocephala and Myosurus (Ranunculaceae) reveals signals of adaptive evolution to arid and aquatic environments
Expansion and contraction of inverted repeats can cause considerable variation of plastid genomes (plastomes) in angiosperms. However, little is known about whether structural variations of plastomes are assoc...
Identification and expression analyses of B3 genes reveal lineage-specific evolution and potential roles of REM genes in pepper
The B3 gene family, one of the largest plant-specific transcription factors, plays important roles in plant growth, seed development, and hormones. However, the B3 gene family, especially the REM subfamily, has n...
Transcriptome data analysis provides insights into the conservation of Michelia lacei , a plant species with extremely small populations distributed in Yunnan province, China
Michelia lacei W.W.Smith (Magnoliaceae), was classified as a Plant Species with Extremely Small Populations (PSESP) by the Yunnan Provincial Government in both action plans of 2012 and 2021. This evergreen tree i...
Whole genome identification, molecular docking and expression analysis of enzymes involved in the selenomethionine cycle in Cardamine hupingshanensis
The selenomethionine cycle (SeMTC) is a crucial pathway for the metabolism of selenium. The basic bioinformatics and functions of four enzymes involved in the cycle including S-adenosyl-methionine synthase (MA...
Biomass and energy potential of Erianthus arundinaceus and Saccharum spontaneum- derived novel sugarcane hybrids in rainfed environments
Energy canes are viable feedstocks for biomass industries due to their high biomass production potential, lower susceptibility to insects and diseases, better ability to adapt to extreme conditions and clean b...
Comprehensive genomic analysis of Bacillus subtilis and Bacillus paralicheniformis associated with the pearl millet panicle reveals their antimicrobial potential against important plant pathogens
Plant microbiome confers versatile functional roles to enhance survival fitness as well as productivity. In the present study two pearl millet panicle microbiome member species Bacillus subtilis PBs 12 and Bacill...
Chalkiness and premature controlled by energy homeostasis in OsNAC02 Ko-mutant during vegetative endosperm development
Chalkiness is a common phenotype induced by various reasons, such as abiotic stress or the imbalance of starch synthesis and metabolism during the development period. However, the reason mainly for one gene lo...
Genetic diversities in wild and cultivated populations of the two closely-related medical plants species, Tripterygium Wilfordii and T. Hypoglaucum (Celastraceae)
The sustainable supply of medicinal plants is important, and cultivating and domesticating them has been suggested as an optimal strategy. However, this can lead to a loss of genetic diversity. Tripterygium wilfo...
Variation in shoot architecture traits and their relationship to canopy coverage and light interception in soybean ( Glycine max )
In soybeans, faster canopy coverage (CC) is a highly desirable trait but a fully covered canopy is unfavorable to light interception at lower levels in the canopy with most of the incident radiation intercepte...
Genome-wide systematic survey and analysis of the RNA helicase gene family and their response to abiotic stress in sweetpotato
Sweetpotato ( Ipomoea batatas (L.) Lam.) holds a crucial position as one of the staple foods globally, however, its yields are frequently impacted by environmental stresses. In the realm of plant evolution and the...
Mitigation of cadmium-induced stress in maize via synergistic application of biochar and gibberellic acid to enhance morpho-physiological and biochemical traits
Cadmium (Cd), being a heavy metal, tends to accumulate in soils primarily through industrial activities, agricultural practices, and atmospheric deposition. Maize, being a staple crop for many regions, is part...
Applications of humic and fulvic acid under saline soil conditions to improve growth and yield in barley
Enriching the soil with organic matter such as humic and fulvic acid to increase its content available nutrients, improves the chemical properties of the soil and increases plant growth as well as grain yield....
Cyanobacterial elicitor enhances the biomass of Mentha piperita L. and improves the production of high-value rosmarinic acid under in vitro culture of apical meristem
Rosmarinic acid (RA), like other phenolic compounds, is sources of antioxidants and anti-inflammatory agents in medicinal plants. In vitro culture of plants can improve the medicinal plants’ metabolite profile...
Far-red light modulates grapevine growth by increasing leaf photosynthesis efficiency and triggering organ-specific transcriptome remodelling
Growing evidence demonstrates that the synergistic interaction of far-red light with shorter wavelength lights could evidently improve the photosynthesis efficiency of multiple species. However, whether/how fa...
SISTER OF FCA physically associates with SKB1 to regulate flowering time in Arabidopsis thaliana
Proper flowering time is important for the growth and development of plants, and both too early and too late flowering impose strong negative influences on plant adaptation and seed yield. Thus, it is vitally ...
Comparative study of the quality indices, antioxidant substances, and mineral elements in different forms of cabbage
As the second largest leafy vegetable, cabbage ( Brassica oleracea L. var. capitata ) is grown globally, and the characteristics of the different varieties, forms, and colors of cabbage may differ. In this study, f...
Genetic resources of common ash ( Fraxinus excelsior L.) in Poland
Knowledge of genetic structure and the factors that shape it has an impact on forest management practices. European ash ( Fraxinus excelsior L.) has declined dramatically throughout its range as a result of a dise...
Role of gamma-irradiated sodium alginate on growth, physiological and active components of iceberg lettuce ( Lactuca sativa ) plant
One of the most widely recognized biostimulators of plant development; is oligoalginate, which regulates the biological processes of plants and was used in horticultural fields as a plant growth regulator. The...
Cytological characteristics of blueberry fruit development
Using the blueberry cultivar "Powderblue" after pollination, fruits at different developmental stages were collected for study. The transverse and longitudinal diameters, individual fruit weight, and fruit wat...
Genetic mapping of deoxynivalenol and fusarium damaged kernel resistance in an adapted durum wheat population
Fusarium head blight (FHB) infection results in Fusarium damaged kernels (FDK) and deoxynivalenol (DON) contamination that are downgrading factors at the Canadian elevators. Durum wheat ( Triticum turgidum L. var....
Integrated analysis of the transcriptome and metabolome reveals the molecular mechanism regulating cotton boll abscission under low light intensity
Cotton boll shedding is one of the main factors adversely affecting the cotton yield. During the cotton plant growth period, low light conditions can cause cotton bolls to fall off prematurely. In this study, ...
Species delimitation of tea plants ( Camellia sect. Thea ) based on super-barcodes
The era of high throughput sequencing offers new paths to identifying species boundaries that are complementary to traditional morphology-based delimitations. De novo species delimitation using traditional or ...
Modulation of early gene expression responses to water deprivation stress by the E3 ubiquitin ligase ATL80: implications for retrograde signaling interplay
Primary response genes play a pivotal role in translating short-lived stress signals into sustained adaptive responses. In this study, we investigated the involvement of ATL80, an E3 ubiquitin ligase, in the d...
The growth, nutrient uptake and fruit quality in four strawberry cultivars under different Spectra of LED supplemental light
An experiment was conducted in a greenhouse to determine the effects of different supplemental light spectra on the growth, nutrient uptake, and fruit quality of four strawberry cultivars. The plants were grow...
‘ Fertile island’ effects on the soil microbial community beneath the canopy of Tetraena mongolica , an endangered and dominant shrub in the West Ordos Desert, North China
The fertile islands formed by shrubs are major drivers of the structure and function of desert ecosystems, affecting seedling establishment, plant–plant interactions, the diversity and productivity of plant co...
KAKU4 regulates leaf senescence through modulation of H3K27me3 deposition in the Arabidopsis genome
Lamins are the major components of the nuclear lamina, which regulate chromatin structure and gene expression. KAKU4 is a unique nuclear lamina component in the nuclear periphery, modulates nuclear shape and s...
Nutrient availability and plant phenological stage influence the substrate microbiome in container-grown Impatiens walleriana ‘Xtreme Red’
The microbiome plays a fundamental role in plant health and performance. Soil serves as a reservoir of microbial diversity where plants attract microorganisms via root exudates. The soil has an important impac...
Important information
Editorial board
For authors
For editorial board members
For reviewers
- Manuscript editing services
Annual Journal Metrics
2022 Citation Impact 5.3 - 2-year Impact Factor 5.9 - 5-year Impact Factor 1.415 - SNIP (Source Normalized Impact per Paper) 1.159 - SJR (SCImago Journal Rank)
2023 Speed 14 days submission to first editorial decision for all manuscripts (Median) 134 days submission to accept (Median)
2023 Usage 3,148,425 downloads 1,056 Altmetric mentions
- More about our metrics
- Follow us on Twitter
BMC Plant Biology
ISSN: 1471-2229
- General enquiries: [email protected]
What keeps the style under tension? Experimental tests to understand the biomechanics of the explosive style movement in Marantaceae
- Marcus Jerominek
- Regine Claßen-Bockhoff
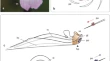
Morphological, genetic and ecological divergence in near-cryptic bryophyte species widespread in the Holarctic: the Dicranum acutifolium complex (Dicranales) revisited in the Alps
- Thomas Kiebacher
- Péter Szövényi
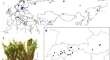
Phosphate environment and phosphate uptake studies: past and future
- Tetsuro Mimura
- Robert Reid
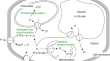
Reviewing impacts of biotic and abiotic stresses on the regulation of phosphate homeostasis in plants
- Laurent Nussaume
- Satomi Kanno
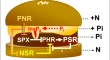
Tolerance to mild shading levels in cattail as related to increased photosynthesis and changes in its leaf area and anatomy
- Carlos Henrique Goulart dos Reis
- Poliana Noemia da Silva
- Fabricio José Pereira
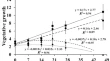
Effect of robbing intensity on reproductive success of Symphytum officinale (Boraginaceae)
- Nurbiye Ehmet
- Tai-Hong Wang
- Qin-Zheng Hou
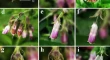
Synergistic regulation of hydrogen sulfide and nitric oxide on biochemical components, exopolysaccharides, and nitrogen metabolism in nickel stressed rice field cyanobacteria
- Garima Singh
- Sheo Mohan Prasad
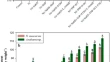
Involvement of GLR-mediated nitric oxide effects on ROS metabolism in Arabidopsis plants under salt stress
- Azime Gokce
- Askim Hediye Sekmen Cetinel
- Ismail Turkan
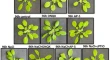
Floral pigments and their perception by avian pollinators in three Chilean Puya species
- Takayuki Mizuno
- Shinnosuke Mori
- Tsukasa Iwashina
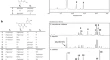
Potassium transporter OsHAK17 may contribute to saline-alkaline tolerant mechanisms in rice ( Oryza sativa )
- Mami Nampei
- Akihiro Ueda
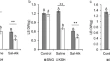
Relictithismia kimotsukiensis, a new genus and species of Thismiaceae from southern Japan with discussions on its phylogenetic relationship
- Kenji Suetsugu
- Yasunori Nakamura
- Shuichiro Tagane
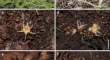
The interaction between heterochrony and mechanical forces as main driver of floral evolution
- Louis P. Ronse De Craene
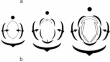
From forest to savanna and back to forest: Evolutionary history of the genus Dimorphandra (Fabaceae)
- Vinicius Delgado da Rocha
- Thaís Carolina da Silva Dal’Sasso
- Luiz Orlando de Oliveira
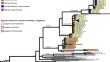
Pyrrolizidine alkaloids are synthesized and accumulated in flower of Myosotis scorpioides
- Kyohei Takano
- Hajime Ikeda
- Kojiro Takanashi
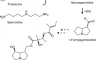
Overexpression of thioredoxin-like protein ACHT2 leads to negative feedback control of photosynthesis in Arabidopsis thaliana
- Yuka Fukushi
- Yuichi Yokochi
- Keisuke Yoshida
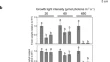
Bud development, flower phenology and life history of holoparasitic Rafflesia cantleyi
- Suk Ling Wee
- Shwu Bing Tan
- Bernard Kok Bang Lee
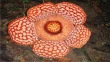
Two lineages of Lemna aequinoctialis (Araceae, Lemnoideae) based on physiology, morphology, and phylogeny
- Takashi Shiga
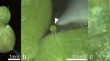
Floral scents, specialized metabolites and stress-response activities in Heritiera fomes and Bruguiera gymnorrhiza from Sundarban mangrove ecosystem
- Ishita Paul
- Sourav Manna
- Mousumi Poddar Sarkar
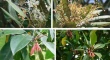
Newly found leaf arrangement to reduce self-shading within a crown in Japanese monoaxial tree species
- Hitoshi Aoyagi
- Miyabi Nakabayashi
- Toshihiro Yamada
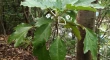
Morphological and physiological response of amphibious Rotala rotundifolia from emergent to submerged form
- Wangai Zhao
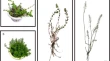
Seasonal and diurnal variations in soil respiration rates at a treeline ecotone and a lower distribution limit of subalpine forests
- Soichiro Takeda
- Naoki Makita
- Koichi Takahashi
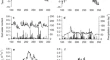
Dominance of non-wetland-dependent pollinators in a plant community in a small natural wetland in Shimane, Japan
- Tomohiro Watazu
- Masayoshi K. Hiraiwa
- Tetsuro Hosaka
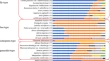
The PpMYB75- PpDFR module reveals the difference between ‘SR’ and its bud variant ‘RMHC’ in peach red flesh
- Xiaomin Xue
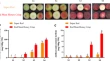
Unreduced spore formation in a spontaneous chimeric pinnule in an artificially produced haploid Anisocampium niponicum (Athyriaceae, Polypodiales)
- Suzue M. Kawakami
- Shogo Kawakami
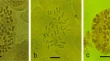
Role of GARP family transcription factors in the regulatory network for nitrogen and phosphorus acquisition
- Naohiko Ohama
- Shuichi Yanagisawa
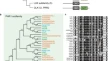
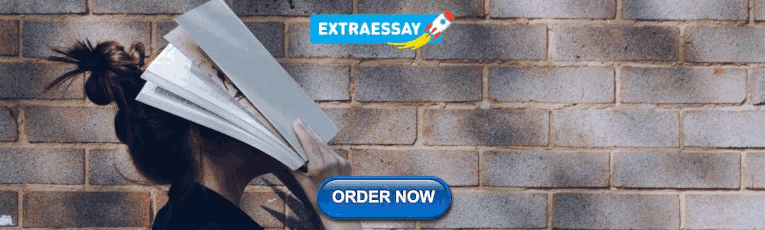
Evidence of an active role of resveratrol derivatives in the tolerance of wild grapevines ( Vitis vinifera ssp. sylvestris ) to salinity
- Faouzia Hanzouli
- Hassène Zemni
- Samia Daldoul
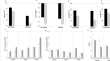
Equisetum praealtum and E. hyemale have abundant Rubisco with a high catalytic turnover rate and low CO 2 affinity
- Sakiko Sugawara
- Yuji Suzuki
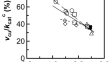
Reproductive interference between alien species in Veronica
- Sachiko Nishida
- Naoko Tamakoshi
- Masahiro M. Kanaoka
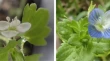
New Year’s greetings 2024 from the Journal of Plant Research
- Maki Katsuhara
Comparative floral development in Mimosa (Fabaceae: Caesalpinioideae) brings new insights into merism lability in the mimosoid clade
- Bruno Cesar Ferreira Gonçalves
- Vidal de Freitas Mansano
- Juliana Villela Paulino
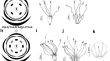
Reproductive isolation between two sympatric bat-pollinated Bauhinia (Leguminosae)
- Sinzinando Albuquerque-Lima
- Ariadna Valentina Lopes
- Isabel Cristina Machado
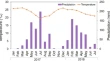
Genome-wide identification and characterization of wall-associated kinases, molecular docking and polysaccharide elicitation of monoterpenoid indole alkaloids in micro-propagated Catharanthus roseus
- Jawad Ahmed
- Yasar Sajjad
- Amjad Hassan
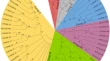
Drought-adapted leaves are produced even when more water is available in dry tropical forest
- Tamires Soares Yule
- Rosani do Carmo de Oliveira Arruda
- Mauro Guida Santos
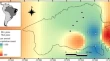
Integrative transcriptomic and metabolomic analyses reveal the phenylpropanoid and flavonoid biosynthesis of Prunus mume
- Chengcheng Qian
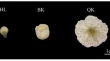
Correction: Impact of mycoheterotrophy on the growth of Gentiana zollingeri (Gentianaceae), as suggested by size variation, morphology, and 13 C abundance of flowering shoots
- Masahide Yamato
Comparative complete chloroplast genome of Geum japonicum : evolution and phylogenetic analysis
- Yujing Miao
- Linfang Huang
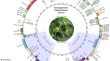
Molecular phylogeny of Vincetoxicum (Apocynaceae, Asclepiadoideae) from Thailand and integrative taxonomy corroborating a new cryptic species within Vincetoxicum kerrii
- Aroonrat Kidyoo
- Manit Kidyoo
- Rumsaïs Blatrix
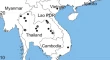
A new mathematical model of phyllotaxis to solve the genuine puzzle spiromonostichy
- Takaaki Yonekura
- Munetaka Sugiyama
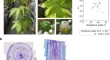
Simultaneous analysis of shape and internal structure of a curved Hibiscus cannabinus pulvinus: X-ray microtomography and semi-automated quantification
- Miyuki T. Nakata
- Masahiro Takahara
- Taku Demura
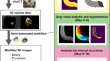
Acknowledgement
Correction to: cyclic guanosine monophosphate improves salt tolerance in solanum lycopersicum.
- Gulnaz Bibi
- Iqra Shafique
- Jamshaid Hussain
Leaf morphometric analysis and potential distribution modelling contribute to taxonomic differentiation in the Quercus microphylla complex
- Oscar Ángel De Luna-Bonilla
- Susana Valencia-Á
- Antonio González-Rodríguez
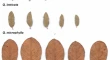
Impact of mycoheterotrophy on the growth of Gentiana zollingeri (Gentianaceae), as suggested by size variation, morphology, and 13 C abundance of flowering shoots
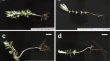
Plasmodesmata callose binding protein 2 contributes to the regulation of cambium/phloem formation and auxin response during the tissue reunion process in incised Arabidopsis stem
- Yusuke Ohba
- Sakura Yoshihara
- Hiroaki Iwai
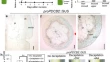
Morpho-physiological profiling of rice ( Oryza sativa ) genotypes at germination stage with contrasting tolerance to salinity stress
- N. Kruthika
- M. N. Jithesh
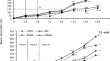
Metal tolerance and Cd phytoremoval ability in Pisum sativum grown in spiked nutrient solution
- Edith Cruzado-Tafur
- Aleksandra Orzoł
- Katarzyna Głowacka
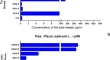
Long-and short-billed hummingbirds as pollinators of Palicourea demissa , a distylous treelet of Neotropical cloud forests
- Zamira Betancourt
- Pascual J. Soriano
- Hamleth Valois-Cuesta
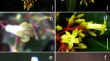
Correction to: Metabolome expression in Eucryphia cordifolia populations: role of seasonality and ecological niche centrality hypothesis
- Camila Fuica-Carrasco
- Óscar Toro-Núñez
- Víctor Hernández
Hidden history of the tobacco BY-2 cell line
- Toshiyuki Nagata
Cyclic guanosine monophosphate improves salt tolerance in Solanum lycopersicum
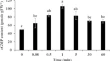
- Find a journal
- Publish with us
- Track your research

An official website of the United States government
The .gov means it’s official. Federal government websites often end in .gov or .mil. Before sharing sensitive information, make sure you’re on a federal government site.
The site is secure. The https:// ensures that you are connecting to the official website and that any information you provide is encrypted and transmitted securely.
- Publications
- Account settings
Preview improvements coming to the PMC website in October 2024. Learn More or Try it out now .
- Advanced Search
- Journal List
- Curr Genomics
- v.17(4); 2016 Aug
Bioinformatics Approach in Plant Genomic Research
a Plant Abiotic Stress Research Group, Ton Duc Thang University, Ho Chi Minh City, Vietnam;
b Faculty of Applied Sciences, Ton Duc Thang University, Ho Chi Minh City, Vietnam;
Phuc Nguyen
c School of Biotechnology, International University, Vietnam National University, Ho Chi Minh City, Vietnam
Nguyen Phuong Thao
The advance in genomics technology leads to the dramatic change in plant biology research. Plant biologists now easily access to enormous genomic data to deeply study plant high-density genetic variation at molecular level. Therefore, fully understanding and well manipulating bioinformatics tools to manage and analyze these data are essential in current plant genome research. Many plant genome databases have been established and continued expanding recently. Meanwhile, analytical methods based on bioinformatics are also well developed in many aspects of plant genomic research including comparative genomic analysis, phylogenomics and evolutionary analysis, and genome-wide association study. However, constantly upgrading in computational infrastructures, such as high capacity data storage and high performing analysis software, is the real challenge for plant genome research. This review paper focuses on challenges and opportunities which knowledge and skills in bioinformatics can bring to plant scientists in present plant genomics era as well as future aspects in critical need for effective tools to facilitate the translation of knowledge from new sequencing data to enhancement of plant productivity.
1. INTRODUCTION
The Plant kingdom is very important not only for human but also for other living organisms. One of the crucial role of plants is to provide a huge amount of food [ 1 ]. Plants are also used in making many human medicines [ 2 ] and have been selected as model organisms to study transposable elements in heterochromatin and epigenetic control [ 3 ]. Study of plant biology has, therefore, been conducted broadly since the early stage of human life because of its vital role.
Modern technologies have pushed the study of plant biology to a higher level than before [ 4 ]. The innovation of high-throughput sequencing methods gives scientists the ability to exploit the structure of the genetic material at the molecular level which is known as “genomics”. Plant genomics study has exploded recently and becomes the main theme in plant research due to the rapid increase of sequenced genomes of many plant species [ 5 ]. It is easy to see the huge impact of plant genome research on the improvement of economically important plants and the knowledge of plant biology [ 6 ]. Open-access and constant updates to this plant genomic information create a fertile environment for plant research to grow. This requires strong connection and cooperation among global biological community [ 7 ].
In this paper, we review firstly the development of genomic sequencing technologies and their applications in plant genomic research. Then, we introduce recent approaches of bioinformatics in managing and analyzing plant genomic databases. Particularly, we summarize most popular plant genomic resources. In addition, we also provide fundamental knowledge of key methods for integration and analysis of these genomic data such as comparative genomic analysis, phylogenomics, evolutionary analysis and genome-wide association study (GWAS) in plant.
2. Next generation sequencing technology in plant genomic research
The development of DNA sequencing technology has been a great and memorial journey filled with many historical events. In the last decade, nearly all of DNA sequence production has restrictively been executed with capillary-based, semi-automated applications of the Sanger biochemistry and its variations [ 8 - 10 ]. Over the years, the field of DNA sequencing has been revived and prospered due to various scientific breakthroughs. These technological advancements eventually lead to the encouragement for developing novel experimental designs for this field due to various reasons [ 11 ]. Ultimately, next-generation sequencing (NGS) technologies were released in 2005 [ 12 ]. They are known as “high throughput sequencing technologies that parallelize the sequencing process, producing millions of sequences at once at a much lower per-base cost than conventional Sanger sequencing” [ 13 ].
Based on NGS technologies, big companies like Roche, Illumina, Applied Biosystems and so forth have recently developed many autonomous and ultrahigh-throughput platforms. All of them are all well-fitted for the current and even future large sequence needs. Generally, Sanger’s dideoxy chain termination sequencing technology is no longer utilized in these NGS platforms. Instead, more advanced methods are applied such as pyrosequencing, sequencing-by-synthesis, sequencing-by-ligation, ion semiconductor-based non-optical sequencing, single molecule sequencing and nanopore sequencing [ 14 ].
Sequencing-by-synthesis platform utilizes DNA polymerase to extend many DNA strands in parallel [ 15 ]. This method uses modified deoxynucleoside triphosphates (dNTPs) containing a terminator which prevents further polymerization, thus, only one single base can be added by DNA polymerase to each growing DNA copy strand. Therefore, the newly incorporated nucleotide or oligonucleotide can be determined as extension proceeds. The pyrosequencing platform is based on the principle of sequencing-by-synthesis (SBS) [ 16 ]. It relies on the detection of pyrophosphate released on nucleotide incorporation by DNA polymerase to facilitate a following series of enzymatic reactions that finally produces light signal from the cleavage of oxyluciferin by luciferase. Sequencing-by-ligation platform uses DNA ligase to create sequential ligation of dye-labeled oligonucleotides. This process enables massively parallel sequencing of clonally amplified DNA fragments [ 17 ]. The discrepancy sensitivity of these clonally amplified DNA fragments is then used to determine the hidden sequence of the target DNA molecule. Ion semiconductor-based non-optical sequencing platform detects the hydrogen ions which are released during DNA polymerization. Single molecule sequencing is based on “the successive enzymatic degradation of fluorescently labeled single DNA molecules, and the detection and identification of the released monomer molecules according to their sequential order in a micro-structured channel” [ 18 ]. Single molecule sequencer does not require any amplification of DNA fragments prior to sequencing [ 19 ]. Nanopore sequencing identifies individual nucleotide sequences as the DNA strand is passed through a membrane-inserted protein nanopore, one base at a time, by alterations in the ion current [ 20 ].
Some examples for well-known NGS platforms commercially available are Genome Sequencer from Roche/454 (Pyrosequencing); Genome Analyzer from Illumina/Solexa (Sequencing-by-synthesis); SOLiD from Applied Biosystems (Sequencing-by-ligation) and Polonator from Dover SystemsP (Sequencing-by-ligation), Ion Torrent from Life Science, Inc. (Ion semiconductor-based non-optical sequencing); Heliscope sequencers from Helicos Bioscience Corporation (True single molecule sequencing); PacBio RS sequencers from Pacific Biosciences (Single molecule, real-time sequencing); GridION and miniaturized MinION sequencers from Oxford Nanopore Technologies (Nanopore sequencing) [ 4 , 14 ].
The main differences among these systems are the length of a sequence read, the unique error model that they applied and the operation cost [ 21 - 24 ]. These differences may affect how the reads are utilized in bioinformatics analyzes, depending upon the application [ 19 ]. However, most of the results finally showed that the data produced are similar among these methods [ 21 - 24 ]. Therefore, it mainly depends on the ultimate goal of a particular research that one may choose the appropriate sequencing methods.
With its rapid innovation, NGSs have been well applied to many aspects in plant genomic research, such as exome sequencing and studying genetic transmission of alleles/quantitative trait loci (QTLs) through whole genome sequencing [ 14 ]. Exome sequencing can effectively help in exploring biodiversity, studying host–pathogen interactions, investigating the natural evolution of crops, testing for the inheritance of genetic markers, providing large-scale genetic resources for the crop improvement, identifying the genes and establishing the presence of functional gene sets that are involved in symbiotic or other co-existential systems [ 14 ]. In addition, NGS methods with single-base resolution can provide epigenomic information. For instance, a study in A. thaliana epigenome revealed that the location and abundance of small RNA targets were significantly related to cytosine methylation [ 25 ]. Another application of plant genome sequencing is genotyping by sequencing (GBS), which is emerging as high through-put and inexpensive method for optimizing genotype populations. GBS has many approaches for enhancing genomic map construction, especially single nucleotide polymorphisms (SNPs) identification [ 26 ]. 681,257 SNP markers of 2,815 maize inbred accessions were found to be positively associated with trait related genes by performing GBS [ 27 ].
The successful application of NGSs in plant genomic research is undoubtable. However, there are challenges in developing computational tools for analyzing genome sequences. Galaxy ( http://galaxyproject.org ) is one of the software systems in which researchers can easily use analysis tools through web-based interfaces comprised of enormous free-accessed biological data [ 28 ]. Another software is Artemis, which is freely available from Sanger institute ( http://www.sanger.ac.uk/ ). It provides genome browser and annotation tool [ 29 ]. There are several other genome sequence analysis tools given by The Broad's Genome Sequencing and Analysis Program (GSAP) ( http://www.broadinstitute.org/ ). Additionally, the rapid decrease in cost of genome sequencing leads to the urgent requirement of a development of huge database storage and management. In fact, there are more and more plant genomic databases have been generated to confront with that demand.
3. PLANT GENOMIC RESOURCES
The history of plant genomics has been changed dramatically by the creation of expressed sequence tag (EST) sequencing, a high-throughput gene discovery method [ 30 ], and the release of the complete Arabidopsis thaliana genomic sequence in 2000 [ 31 ]. Following that success, the complete genomic sequence of rice became available only 2 years later [ 32 ]. These events have created powerful waves on both plant biotechnology and crop bioinformatics. For the advancement of learning, more sequencing projects on vital plant species have been carried out by combining novel in silico technologies from genomic research with traditional breeding schemes for further enhancing the quality of crops.
With the advent of NGS technology in 2005 [ 33 ], the number of plant genomes sequenced have dramatically increased to more than 100 species in 2014 according to CoGepedia, a platform that aims to record all plant genomes with published or in-processed sequences [ 12 ]. Throughout the years, these genomes have contributed many valuable materials for plant research in modern molecular genomics era. Based on that foundations, genetical/biological activities of many critical genes and pathways have been revealed [ 34 ]. For instance, plant species such as Arabidopsis [ 31 ], Brachypodium distachyon (grass) [ 35 ], Physcomitrella patens (moss) [ 36 ] and Setaria italic (millet) [ 37 , 38 ] can be used as scientific model for genomic studies in drought tolerance [ 39 ]. Others like Oryza sativa (rice) [ 40 , 41 ], Populus trichocarpa (poplar) [ 42 ], Zea mays (maize) [ 43 ], Glycine max (soybean) [ 44 ], Solanum lycopersicum (tomato) [ 45 ], and Pinus taeda (loblolly pine) [ 46 ] can serve as both crops and functional models [ 34 ].
Non-model and non-crop plant genomes can also tell a story about genome construction and flowering plant evolution [ 34 ]. For examples, Utricularia gibba (bladderwort) and Genlisea aurea (corkscrew) genomes can provide significant understanding about genome size variation [ 47 , 48 ]. Furthermore, Spirodela polyrhiza (greater duckweed) genome which share the similarity in size with that of Arabidopsis but only needs 28% fewer genes to function normally [ 49 ]. In another case, the genomes of Selaginella moellendorffii (spikemoss) and Amborella trichopoda present the bridge between the evolution of vascular plants and angiosperms respectively, revealing fundamental understandings about the trajectory of plant specific gene families and the radiance of flowering plants, thus, shedding more light in the evolution of flowering plant [ 34 ].
The gene knowledge drawn from genomics can be utilized to recognize, classify, exploit and tag individual alleles as well as to promote and manipulate molecular markers to track the desired alleles in breeding programs [ 50 ]. For those reasons, many genome sequencing projects in the field of horticultural crops were carried out such as Tomato genome sequencing project ( www.sgn.cornell.edu/about/tomato ) [ 45 ], Potato genome sequencing consortium, (www.potato genome.net) [ 51 ], Papaya genome sequencing project ( www.asgpb.mhpcc.hawaii.edu/papaya/ ) [ 52 ], Grape genome sequencing project ( www.vitaceae.org ) [ 53 ], Floral genome sequencing project ( www.fgp.bio.psu.edu/ ) [ 54 ] and hopefully many more will be available in public domain for scientific usages in near future. Combining with traditional methods, these projects were armed with advanced sequencing technologies, to fully certify generation of high-quality sequences and budget-efficient design [ 55 ]. Therefore, these whole-genome sequencing projects may have great significant impact in global food insurance and bio-energy advancement by providing invaluable resources for comparative and functional genomic studies [ 55 ]. If current research keeps moving forward, noticeable impact on global human well-being may be seen through applications of genomic science resources to horticulture plant species.
The availability of complete genome sequences, as well as the explosion of sequence data, is leading to an urgent need for well-catalogued and annotated DNA sequence databases. The largest and most well-known of these sequence databases are GenBank, EMBL and DNA Data Bank of Japan [ 32 ]. These databases are acknowledged as the standard figure for public annotated DNA sequence collection worldwide and contain millions of plant DNA sequences. Take NCBI as an example, up to 2015, NCBI Genome database have been increased to a total of 5,132,285 plant accession entries according to RefSeq Growth Statistics ( http://www.ncbi.nlm.nih.gov/refseq/statistics/ ). Back to 2004, there were only 88,972 entries, thus, the growth rate is approximately 458,483 entries per year over ten years, which means more than 38,000 sequences are updated monthly.
There are other public databases which may provide extra information on plant genome such as Phytozome [ 56 ], PlantGDB [ 57 ], EnsembPlants, ChloroplastDB [ 58 ], KEGG [ 59 ], Genomes On-Line Database (GOLD) [ 12 ] and the wiki of CoGepedia web page (Table 1 1 ). Recently, in addition to these general sequence data banks, other databases that focus on specific plant species have been available. Some examples for species-specific sequence databases are The Arabidopsis Initiative Resource (TAIR) [ 60 ], The Salk Institute Genomics Analysis Laboratory (SIGnAL), The RIKEN Arabidopsi s Genome Encyclopedia (RARGE) [ 61 ], The Rice Genome Annotation Project (RGAP) [ 62 ],The Rice Annotation Project (RAP-DB) [ 63 ], The Solanaceae (SOL) Genomics Network (SGN) [ 64 ], Gramene [ 65 ], GrainGenes [ 66 ], SoyBase [ 67 ], MaizeGDB [ 68 ], CyanoBase [ 69 ], the Genome Database for Rosaceae (GDR) [ 70 ], Brassica Genome Gateway and Cucurbit Genomics Database (Table 1 ) [ 71 ]. Commonly, these databases and associated web portals incorporate a set of analytical, visualization and interrogation tools to study the genomic sequences they process such as BLAST for identifying sequence similarity in large datasets.
List of plant genomic databases.
4. Plant cOMPARATIVE GENOMIC ANALYSIS
Once whole genomes have been sequenced, defining and describing the gene and non-coding content in these sequences is an important process [ 72 ]. For that reason, plant comparative genomic analysis has arisen as a new field of modern biotechnology since its main function is to predict functions for many unknown genes by studying the significant differences and similarities among species. These genes, however, are required to appear in the available datasets of orthologs evolved from the same ancestor [ 73 ]. As can be seen, developing new tools, strategies to manage and analyze these tremendous data has been urgently needed. Recent approaches in bioinformatics and systematic biology have reached those demands but still faced further challenges.
4.1. Tools and Databases for Plant Comparative Genomic Analysis
Using comparative genomic approach, more and more genes in plant species have been annotated. For instance, several known stress-responsive transcription factors (TFs) in Arabidopsis and rice were used to correctly predict stress-responsive TFs in many other plant species, such as soybean, maize, sorghum, barley, and wheat [ 74 - 76 ]. Moreover, not only comparing within plant species, comparative genomics between plants and distantly related prokaryotes can be greatly presumed the genes functionally associated. The function of NiaP protein family in plants was determined from knowing the role of those proteins in bacteria [ 77 ]. Similar strategies to identify functional genes among different plants using comparative analysis also help researchers study genes annotation in newly sequenced plant species [ 78 ].
In addition, comparative genomics can discover missing biosynthetic genes by co-expression analysis [ 79 ]. This
method performs by considering an unknown gene that is co-expressed with various genes from a metabolic pathway which is expected to have a function in that particular pathway [ 80 , 81 ]. GolmTranscriptome DB [ 82 ] and ATTED-II [ 83 ] are two popular tools for such type of analysis in plants. One case for this analysis is the discovery of trans-prenyldiphosphate synthase responsible for making the solanesyl moiety of ubiquinone-9. Arabidopsis gene At2g34630 was identified as an alternative candidate using the co-expression and under-expression analysis in Arabidopsis and by functional complementation in yeast [ 84 ].
Besides tools and strategies for analysis, powerful computational resources are essential to store and manage massive genomic data. Many online platforms have been developed, published and available to perform comparative genomic study among different plant species. For instance, several plant genomic data platforms described below have been the most representative and widely used recently.
Phytozome. One of the largest comparative databases for plant species ( http://phytozome.jgi.doe.gov/pz/portal.html ). It contains plant genome, gene family data, and evolutionary history information. From the beginning, only 25 plant genomes were sequenced and annotated. This number has increased up to more than 50 species at the current state. Phytozome also provides impressive tools for comparative analysis in level of sequence, gene structure, gene family, and genome organization. With those tools and comprehensive web portal, Phytozome makes it accessible for scientist worldwide conducting plant research intensively [ 56 ].
PLAZA . Being known as the most comprehensible plant comparative genomics online platform, PLAZA integrates functional and structure annotation of all currently published crop plant genomes ( http://plaza.psb.ugent.be/ ). Together with that huge set of data, PLAZA provides many interactive tools to study gene, genome evolution, and gene function. Those tools include pre-computed datasets cover, intraspecies dot plots, whole-genome multiple sequence alignments, homologous gene families, phylogenetic trees, and genomic colinearity between species [ 85 ].
GreenPhylDB . A web resource belongs to South Green Bioinformatics Platform ( http://southgreen.cirad.fr/ ) and is open to public access. GreenPhylDB is designed for comparative and functional genomics in plants. This database contains 37 full genomes of members of the Plant kingdom at the current release version 4. Catalogue of gene families from GreenPhylDB is provided by gene predictions of genomes, covering a broad taxonomy of green plants. Its web interfaces have been continually developed to improve the navigation through information related to each gene or gene family, such as gene composition, protein domains, publications, orthologous gene predictions, and also external links. The latest version of this database is now possible to browse the full Gene Oncology, which supports gene discovery [ 86 ].
PlantsDB. This is one of the most commonly used plant database resources for integrative and comparative plant genome research ( http://mips.helmholtz-muenchen.de/plant/genomes.jsp ). PlantsDB comprises database instances for tomato, Medicago , Arabidopsis , Brachypodium , Sorghum , maize, rice, barley and wheat. This platform stores and provides individual plant genomes. Moreover, it is also equipped with up-to-date bioinformatics tools to visualize synteny, transfer data from model systems to crops and explore similarities and peculiarities of different plant species. Further important analysis strategies developed from PlantsDB are repeat catalogs and classification systems for all plant species [ 87 ].
4.2. Remaining Challenges
The enormous amount of genomic data for plants rapidly increases. Thousands of Gb of plant sequences are deposited in NCBI and other public databases monthly. However, reference genome sequence with basic annotation provided by current comparative genomic databases is simply a foundation. It still needs to be integrated with specific biological data such as plant epigenetic decorations and gene expression under vary conditions of environment, development stages and tissue types in order to get better detailed genome maps [ 34 ].
Moreover, since plant genomes have been constantly sequenced and re-sequenced, there is rising problem in updating databases. The update process should occur in all comparative genomic databases, not just solely in that individual genome database. This technical problem requires efforts to synchronize update data resources among different plant genomic platforms. Developing a strong community network of plant researchers might be one solution for this issue [ 88 ].
Several databases have been developed, published and available to compare plant genomes and tentatively identify orthologs (Table 1 ). Having powerful application in gene prediction, comparative genomics recently has played an important role in contributing the functional annotation infrastructure on which future plant biotechnology researchers rely on.
5. PHYLOGENOMICS AND EVOLUTIONARY ANA- LYSIS IN PLANT
Phylogenomics is known as molecular phylogenetic analysis, in which using sets of genomic database for gene function prediction and exploration of the evolutionary relationships among species. This definition of phylogenomics was formed from the early studies in the late 1990s when a scientific hypothesis about protein function via evolutionary analysis of a gene and its homologs was published [ 89 ]. Phylogenomics was also defined as the new era of phylogenetic analysis when there are more complete genomes sequenced [ 90 ]. Plant phylogenomics has an advantage over other species, which is the ability to identify hundreds of low copy number nuclear genes, hence easily to study the molecular systematic and evolutionary biology [ 91 ]. Current approaches of NGS also provide plant phylogenomics research useful information about plant genome diversity, such as the nature and frequency of genome duplication among a diversity of plant lineages [ 92 - 94 ].
There are two important goals in phylogenomic research aims to accomplish. First is to discover the evolutionary patterns among plant species using nuclear genomic information. Second is to derive new hypothesis for the unknown function of plant genes associated to major divergence events in the evolution of plant species [ 95 ]. Genomic data give more advantages in the evolutionary study than morphological data which are easily misleading or fossil data which are usually fragmented. Phylogenomics also uses a set of orthologs from genomic sequence via a phylogenetic context to detect hypotheses for the genes and biological processes [ 96 ]. The main difference between functional phylogenomics compare to classical phylogenetic analysis methods and current functional genomic methods is that in phylogenomics research, genomic information is mined without incorporating a phylogenetic context during the search for orthologs or candidate genes of functional importance [ 97 ]. However, it remains a debating issue in constructing the tree of life (phylogeny of all organisms), which inferred evolutionary relationship using phylogenomics as the advance method. Some studies continuously revalidated the positions of certain plant species in biological taxonomy [ 98 - 100 ] to get the most accurate tree as possible. Therefore, how to draw a scientifically significant topology is still problematic due to some limitations, such as the confliction among methodologies and character sets [ 101 ] and systematic errors from merely adding more sequences [ 102 ].
As shown above, the main problem of phylogenomics comes from how to handle the large scale of genomic data in a proper way to avoid systematic misleading (bias) assumptions. Statistical confidence ( P value ) which is normally used in such phylogenetic issue manner, however, was reported as unreliable. The authors then suggested that the magnitudes of differences (effect sizes) and biological relevance are those should be more focus on to get trustworthy results [ 103 ]. Another solution is the improvement of existing phylogenetic algorithms so that phylogenomic relationships can be inferred with minimal technical biases and greater computer efficiency [ 104 ].
New methods and tools have been developed to gradually overcome these limitations of plant phylogenomics. For instance, de novo assembly of short read RNA-seq data dramatically improves gene coverage by non-redundant and non-chimeric transcripts that are optimized for downstream phylogenomic analysis [ 105 ]. Another protocol is called Hyb-Seq, which combines target enrichment of low-copy nuclear exons and flanking regions, as well as genome skimming of high-copy repeats and organelle genomes, to efficiently produce genome-scale data sets for plant phylogenomics [ 106 ]. More recently, ExaML (Exascale Maximum Likelihood), which is usually known as new code for large-scale phylogenetic analyzes on Intel MIC (Many Integrated Core) hardware platform, has been updated its version 3. This coding program represents the achievement of developing better phylogenetic analysis algorithms, it is now possible to analyze datasets with 10-20 genes and up to 55,000 taxa [ 107 ]. However, even though it is just released few months ago, ExaML still has its limit since it can only run on supercomputer with Linux/Mac system. Obviously, new plant phylogenomic tools similar to ExaML is desperately needed with high quality performance and easy to operate in any computational system in the future.
6. GENOME-WIDE ASSOCIATION STUDIES IN PLANT
Basic knowledge of phenotypic variation, such as those agronomically important traits used for plant breeding resources has been the main trend of plant genetic studies. In classical crop breeding, biparental cross-mapping is still a major method for genetics dissections of the traits although its limitation is giving the QTLs mapping with low resolution (typically with several megabases in distance) [ 108 ]. To overcome that disadvantage, GWAS is currently a favorable tool to explore the allelic variation in a broader scope for extensive phenotypic diversity and higher resolution of QTL mapping thanks to the advent of NGS. Using GWAS, many research projects have been done to investigate the association between genetic variation and valuable plant traits. GWAS has been successfully applied to study Arabidopsis thaliana , a typical model plant organism, in which more than 1,300 distinct accessions have been genotyped for 250,000 SNPs [ 109 ] and 107 phenotypes have been studies [ 110 ]. Following this initial foundation, there were numerous achievements in conducting GWAS on other traits of interests in Arabidopsis , such as glucosinolate levels [ 111 ], shade avoidance [ 112 ], heavy metal [ 113 ], salt tolerance [ 114 ] and flowering time [ 115 ], etc. Beside Arabidopsis , rice, one of the most important crop species in the world, also has been focus of intense efforts to map the ancestral genetic variation that underlines agronomic traits such as heading date, grain size, and starch quality [ 116 ]. A few rice genes having large effects in controlling traits are involved in determining yield, morphology, stress tolerance, and nutritional quality were also identified [ 117 ]. GWAS has been widely used to dissect complex traits in some other major crops, e.g., maize and soybean [ 118 - 122 ].
It is undeniable that GWAS has the powerful application to plant species for identifying phenotypic diversity in trait-associated loci, as well as allelic variation in candidate genes addressing quantitative and complex traits [ 123 , 124 ]. However, to accelerate genetic mapping and gene discovery in plant using GWAS, besides massive DNA variation data from NGS, it requires having a high-through put phenotyping facility that is capable to capture in details specific traits to enhance GWAS results and gain more significant gene identification information [ 125 ]. It is a challenging and promising road for future plant genomic mapping research. Hence, there are efforts on making high quality phenotyping data [ 126 - 129 ]. Furthermore, having computational tools to assist GWAS is also concerning issue. There are three main factors required for a GWAS tool to well perform including computing speed, memory requirements, and statistical power [ 130 ]. At the current stage, several bioinformatics approaches have been introduced as GWAS acceleration tools. Following are some examples:
Heap. Heap is a SNPs detection tool for NGS data with special reference to GWAS and genomic. Heap detects larger number of variants taking advantage of the information whether the samples are inbred (homozygosity assumption) or not. For data portability to GWAS/GP, Heap outputs variant information in vcf, beagle and PED/MAP format files that are compatible with existing GWAS/GP tools [ 131 ].
GnpIS-Asso . GnpIS-Asso is a generic database for managing and exploiting plant genetic association studies. This database provides tools that allow plant scientists or breeders to get associations values between traits and markers obtained in several association studies. It is also easy to view graphically the results with dedicated plots (QQPlot, Manhattan Plot), generated dynamically and to extract data in files to continue the analysis with external tools. After selecting the best markers associated to trait of interest, one specific tool automatically jumps on the genome to find where those markers are located on chromosomes and to identify which genes or other markers or features of interest are nearby. This database is already currently used for dealing GWAS for two species: tomato and maize [ 132 ].
BioGPU . As a high performance computing tool for GWAS, BioGPU effectively controls false positives caused by population structure and unequal relatedness among individuals and improves statistical power when compared to mixed linear model methods. The BioGPU method requires much less complex computing time. BioGPU was developed with parallel computational capacity to increase computing speed, so that computing time decreases linearly with the number of central processing units. To solve the memory footprint bottleneck, BioGPU allows users to directly control memory usage when big data are analyzed on computers with limited memory, which means users have the option to trade computing time for less memory usage. Based on these features, BioGPU makes analyzes of large and complex datasets feasible without supercomputers [ 130 ].
BHIT . Bayesian high-order interaction toolkit (BHIT) first builds a Bayesian model on both continuous data and discrete data, which is capable of detecting high-order interactions in SNPs related to case-control or quantitative phenotypes. Using both simulation data and soybean nutritional seed composition studies on oil content and protein content, BHIT effectively detects the high-order interactions associated with phenotypes, and it outperformed a number of other currently available tools. BHIT are also used on Soybean 50K SNP array analysis by diversity computational strategies. Then a series of SNP interactions in multiple-orders are detected associated with oil and protein phenotypes. BHIT is freely available at http://digbio.missouri.edu/BHIT/ for academic users [ 133 ].
While it was time-consuming in the past to perform QTL analysis a small data, recent bioinformatics approach helps running GWAS with a simple marker scan of few hundred thousand SNPs on PC or web-based software within few minutes [ 123 ]. However, future GWAS assisted tools still need to be improved in speed and increased memory capacity in order to integrate with rapidly growing plant genomic data. Moreover, to ensure the accuracy of GWAS results, statistical test is very important factor and must be applied intensively, in which mixed models are set as the error-making factor of genetic background [ 134 , 135 ]. One example for this is a GWAS online tool is the one for Arabidopsis , which was developed based on R and Python programming languages [ 136 ]. This web-based server comprises of common accessions with their genotyping information and several statistical options as well as integrates correlation analysis among published traits [ 136 ].
In combination with high resolution phenotyping technologies, performing GWAS is a novel strategy for conducting research on plant genetics, genomics, gene characterization and breeding [ 137 ]. Nevertheless, GWAS analysis still has another limitation, which is failure in detecting epistatic and gene-environment interactions in most studies [ 138 ]. Due to the fact that living organisms express their phenotypes as the result of not only one but several factors including epistatic effects and their interactions with environment; hence it is important to estimate those gene-gene and gene-environment interactions for better breeding system [ 139 , 140 ]. Focusing on one main SNP that correlates with a specific phenotype as normal GWAS output may miss the key genetic variants with particular environment response in the context of complex traits [ 141 ]. For this issue, bioinformatics approach is again a current solution. Generalize multifactor dimensionality reduction (GMDR) algorithm on a computing system with graphics processing units (GPUs) is one in some available methods at the moment that can screen potential candidate variants and then use the mixed liner model to detect the epistatic and gene-environment interactions [ 142 ]. This new GWAS strategy was applied and showed its success in identifying four significant SNPs associated with additive, epistatic, and gene-environment interaction effects in rice [ 138 ]. Similar GWAS method using epistatic association mapping (EAM) also successfully detected three epistatic QTLs in soybean [ 143 ]. Those presented methods are just the groundwork, future bioinformatics tools have to be more powerful in statistical methodology and overcome the heavy burden of current computation [ 144 - 146 ].
7. BIOINFORMATIC ADVANCES BEYOND PLANT GENOMIC RESEARCH
The world is now at the post genomic era since DNA sequencing technology continues reaching unprecedented innovations in sequencing scale and throughput. In particular, the term “genomics” by itself is only just a small part in the whole picture named “Omics”. With the development of modern technology, several new omics layers have been emerged to deepen the knowledge of plant molecular system [ 147 ]. The most recent added omics layers include interactomics, epigenomics, hormonomics, and metabolomics. While NGS provides feature for whole-genome sequencing/re-sequencing for various genomic analysis, such as those are discussed across this paper, RNA sequencing (RNA-seq) is established for transcriptome and non-coding RNAome analysis, quantitative detection of epigenomic dynamics, and Chip-seq analysis for DNA–protein interactions [ 148 ]. In addition, approaches in transcriptional regulatory networks research based on omics data have been published such as interactome analysis for networks formed by protein–protein interactions [ 149 ], hormonome analysis for phytohormone-mediated cellular signaling [ 150 ], and metabolome analysis for metabolic systems [ 151 ]. Apparently, these rapidly growing omics databases widen the large-scale of genomic resources. Therefore, bioinformatics has become more essential than ever for every aspect of omic-based research to be well managed and effectively analyzed.
8. CONCLUSIONS
Recent advances in bioinformatics application for plant genomes not only provide huge potential for large-scale genomic research among plant species but also many technical challenges. NGS technologies and platforms will make plant genetic data become abundant in the next few years. With these accessible genomic data, development of effective tools for these data management and analysis become increasingly important. Indeed, there are more and more genome databases of plant species continuously established merging with different analysis methods. Comparative genomic analysis gives a specific insight of functional genes within the same and among plant species. Phylogenomic results show more accurate evidences for evolution studies and hypothesized function of genes in plant. GWAS, which has been currently used in plant research, successfully point out loci and allelic variation related to valuable traits. On the contrary, one of the main challenges facing plant genomic researchers is the high demand of knowledge and skills in bioinformatics as well as computer sciences in order to well manage and intensively manipulate the results from the increasing of large-scale plant genomic data. Moreover, since high density genotype information rapidly exploited, high-throughput phenotyping is urgently needed to provide plant genomic analysis results at high resolution.
In brief, the recent wealth of plant genomic resources, along with advances in bioinformatics, have enabled plant researchers to achieve fundamental and systematic understanding of economically important plants and plant processes, critical for advancing crop improvement. Despite these exciting achievements, there remains a critical need for effective tools and methodologies to advance plant biotechnology, to tackle questions that are hardly solved using current approaches, and to facilitate the translation of this newly discovered knowledge to improve plant productivity.
ACKNOWLEDGEMENTS
“This work was supported by Vietnam National University, HCM under grant number C2014-28-07 to N.P.T. This work was also funded by the Asian Office of Aerospace Research & Development of The United Statesunder grant number FA2386-15-1-419 to L.L.”
CONFLICT OF INTEREST
The author(s) confirm that this article content has no conflict of interest.
Academia.edu no longer supports Internet Explorer.
To browse Academia.edu and the wider internet faster and more securely, please take a few seconds to upgrade your browser .
- We're Hiring!
- Help Center
Plant Biology
- Most Cited Papers
- Most Downloaded Papers
- Newest Papers
- Save to Library
- Last »
- Plant Molecular Biology Follow Following
- Plant biotechnology Follow Following
- Plant Pathology Follow Following
- Plant breeding and genetics Follow Following
- Botany Follow Following
- Plant Physiology Follow Following
- Plant Ecology Follow Following
- Molecular Biology Follow Following
- Biology Follow Following
- Genetics Follow Following
Enter the email address you signed up with and we'll email you a reset link.
- Academia.edu Publishing
- We're Hiring!
- Help Center
- Find new research papers in:
- Health Sciences
- Earth Sciences
- Cognitive Science
- Mathematics
- Computer Science
- Academia ©2024

Sap beetles vs wind: What pollinates screw pines?
Research finds fragrant screw pines are pollinated by sap beetles rather than by wind, overturning previous assumptions.
Researchers Toru Miyamoto, Ko Mochizuki, and Atsushi Kawakita of the University of Tokyo have discovered the first species pollinated by sap beetles in the genus Pandanus , a group of palm-like plants native to the tropics and subtropics of Africa and Eurasia. The discovery overturned the long-held belief that these plants were pollinated by wind. The researchers also found that fragrant screw pines' male and female flowers produced heat at night stably, making them the first such species in the family Pandanaceae . The findings were published in the Botanical Journal of the Linnean Society .
The genus Pandanus , a member of the family Pandanaceae, boasts around 450 species spread around the paleotropical region. The species of this genus have male inflorescences, clusters of flowers on branches, that are pendent and nectarless, which has made scientists assume they are pollinated by wind. However, some of their other properties, such as floral scent and the fact that female inflorescences mature while bracts (specialized leaves) are still present, suggest otherwise. To clarify the pollination mechanisms, the researchers decided to investigate a species native to subtropical Japan called Pandanus odorifer , commonly known as fragrant screw pines.
The researchers took a multistep approach. They conducted fieldwork during three flowering seasons between 2020 and 2022 on five islands of the Ryukyu Archipelago. They observed visitors at various flowering stages. Although many small insects visited the flowers, Amystrops sap beetles were the most numerous. So, the team examined the amount of pollen attached to the beetles' bodies to ascertain whether the amount was enough for pollination. The team also needed to verify whether the beetles were visiting the flowers when they were receptive to pollination. Measuring the receptivity of stigmas, a necessary element for pollination, overlapped with the highest number of beetles found visiting the flowers. These findings together suggest that Amystrops sap beetles are pollinators of fragrant screw pines. However, the team did not stop there. There had been reports of genus Pandanus in the past that claimed their inflorescences were warm. However, thermogenesis had not been formally investigated before.
"We were very surprised," Miyamoto recalls, "when we found that the floral organs of the P. odorifer actually generated heat. Although it was relatively easy to predict that beetles would be found in the inflorescences, we did not expect to discover floral thermogenesis, both in male and female reproductive organs."
Although Pandanus species are one of the iconic species in the paleotropical region, surprisingly little is known about them. So, in addition to the current findings, many more surprising facts must be out there, waiting to be discovered. Thus, Miyamoto lays out the next steps for further research.
"Pollination research in the family Pandanaceae is a hot topic, and there are high hopes for developments in the future. As such, we would like to elucidate the pollination ecology of the family Pandanaceae in regions where its species are diverse, particularly in Madagascar and South-East Asia."
- New Species
- Mating and Breeding
- Insects (including Butterflies)
- Endangered Animals
- Wild Animals
- Willow tree
- Flowering plant
- Water hyacinth
Story Source:
Materials provided by School of Science, The University of Tokyo . Note: Content may be edited for style and length.
Journal Reference :
- Toru Miyamoto, Ko Mochizuki, Atsushi Kawakita. Pollination of thermogenic inflorescence of Pandanus odorifer by a specialist Amystrops sap beetle that reproduces on the male inflorescence . Botanical Journal of the Linnean Society (accepted) , 2024 DOI: 10.1093/botlinnean/boae012
Cite This Page :
Explore More
- Want to Feel Young? Protect Your Sleep
- Century-Old Powdered Milk in Antarctica
- New Artificial Reef Stands Up to Storms
- Persistent Hiccups in a Far-Off Galaxy
- Lighting Up the Future
- Sugarcane's Complex Genetic Code Cracked
- Microbiome of 4,000-Year-Old Teeth
- Magnetic Fields of Milky Way's Black Hole
- Neural Networks Think Alike
- Old Immune Systems Revitalized
Trending Topics
Strange & offbeat.
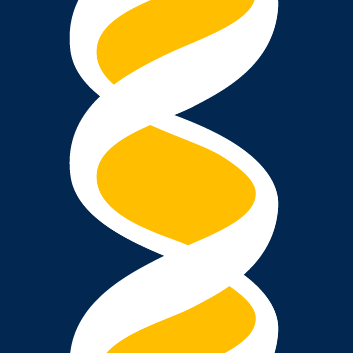
College of Biological Sciences
Researchers identify microbes that help plants thwart parasite.
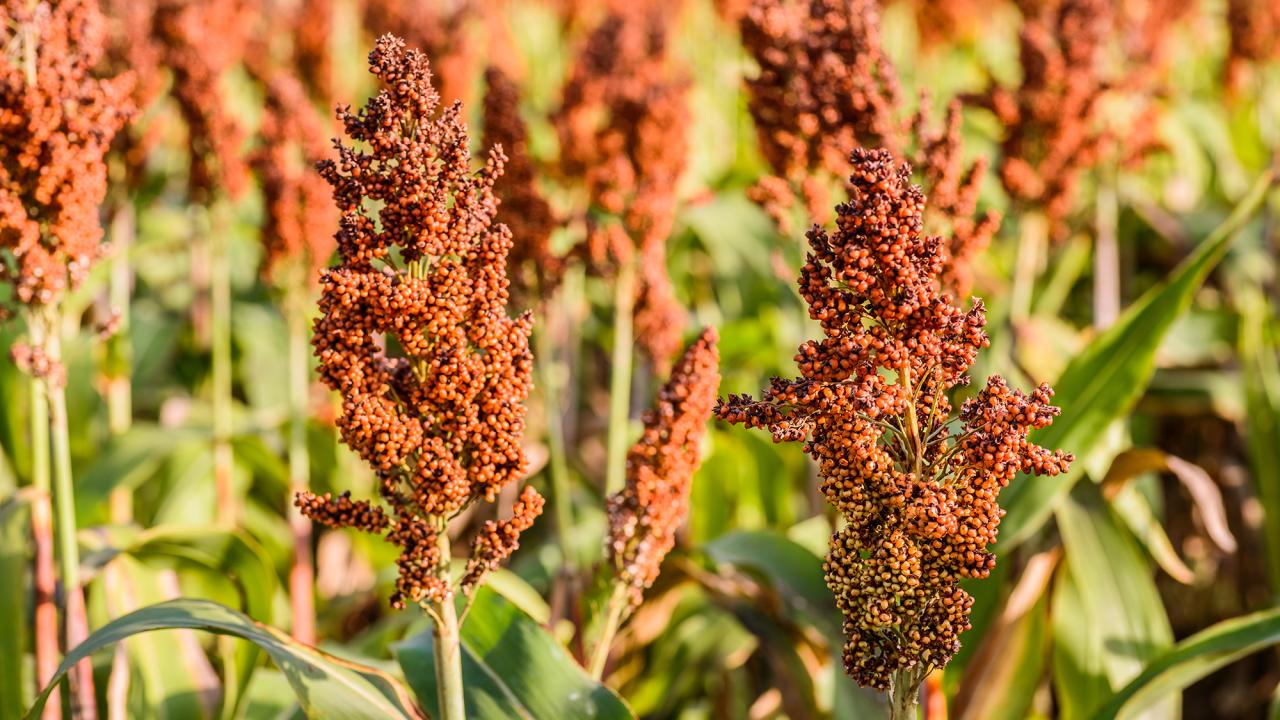
Plant biologists have identified bacteria that help sorghum plants resist infections and could be used as a soil “probiotic”
- by Liana Wait
- March 26, 2024
Sorghum, or broomcorn, is a staple crop in sub-Saharan Africa, but approximately 20% of annual yields are lost due to infections with witchweed ( Striga hermonthica ), a parasitic plant that steals nutrients and water by latching onto the plant’s roots.
In a new study in Cell Reports published on March 26, University of California, Davis researchers show that soil microbes can suppress witchweed infections by inducing changes in sorghum root structure that make it more resistant to infection. The researchers identified specific strains of bacteria that trigger these resistance traits and could be applied as a soil “probiotic” to improve sorghum yields in future.
“These microbes have great promise as soil additives that can help farmers grow sorghum successfully in sub-Saharan Africa,” said Siobhan Brady, a professor in the Department of Plant Biology and Genome Center, and a senior author on the paper.
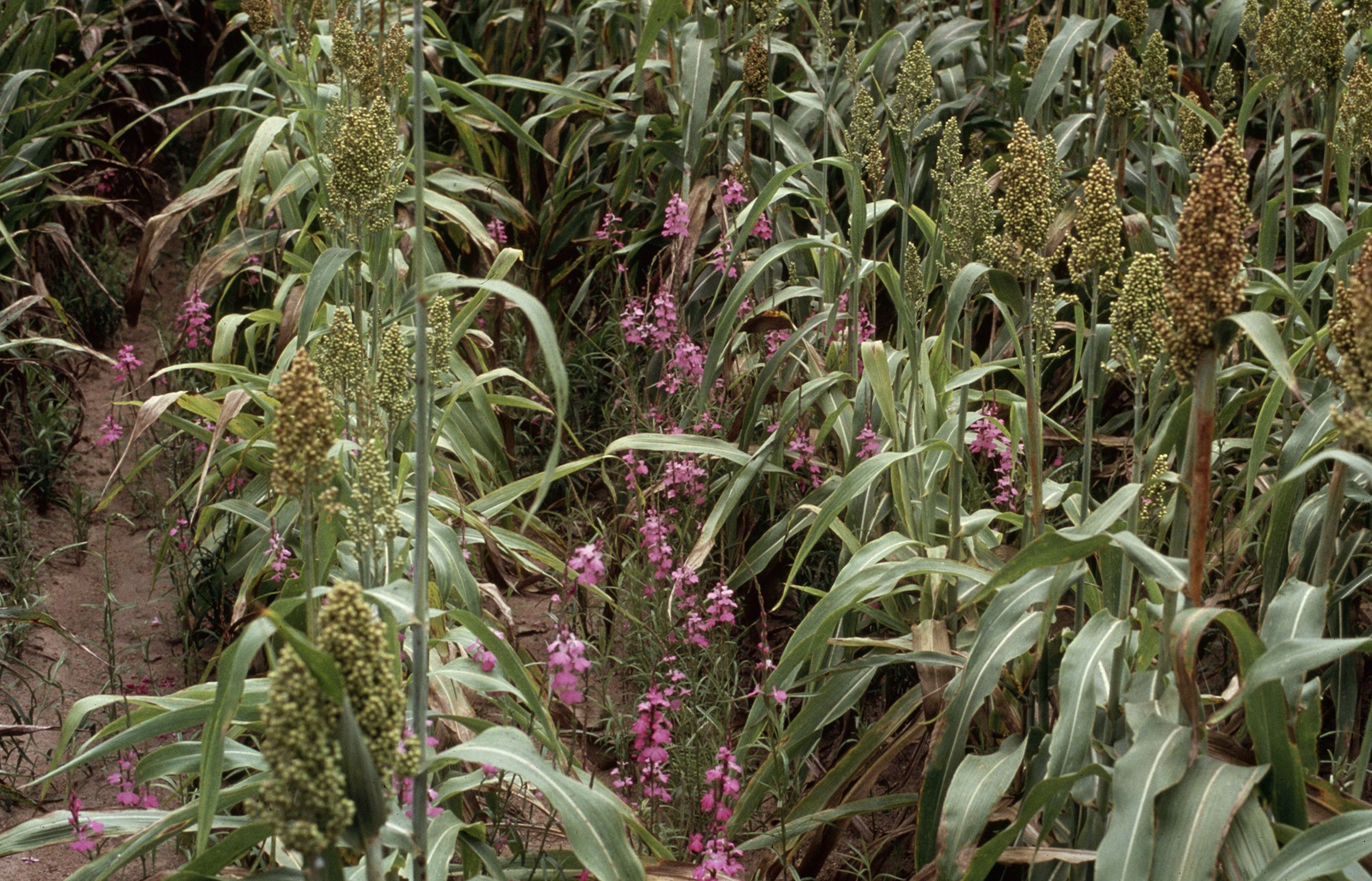
A witchy weed
Witchweed is a major issue for smallholder farmers in sub-Saharan Africa. The parasitic plants produce thousands of tiny seeds that can remain dormant in the soil for up to 20 years, making them extremely difficult to eradicate. Current control methods—which include applying chemical agents, crop rotation and breeding resistant sorghum—have only achieved partial control, and many are inaccessible to the farmers who need them most.
In the new study, postdoctoral scholar Dorota Kawa worked alongside Brady and collaborators in the Netherlands and Ethiopia to show that soil microbes can mitigate witchweed infections in sorghum.
“This is the first example showing how microbes can induce changes in host root cells that are associated with the suppression of witchweed,” said Brady.
Hijacking a plant signal
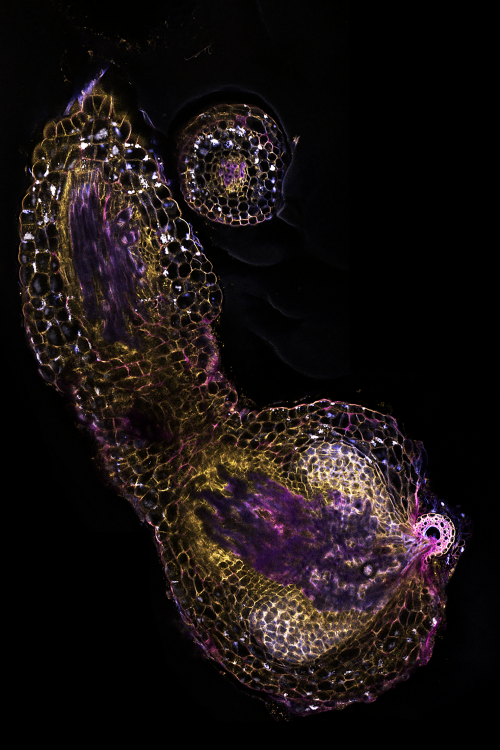
When sorghum plants find themselves in low-phosphate soil, they release chemicals from their roots to attract fungi to join them to help acquire phosphate. Unfortunately for sorghum, witchweed has evolved to respond to this same signal.
“This parasitic plant has hijacked the signaling so that its seeds germinate when they perceive that same signal from the root,” said Brady.
After germinating, witchweed responds to additional chemical cues from sorghum that trigger the parasite to grow appendages called “haustoria” that enable it to latch on and penetrate the sorghum roots.
“Once it has made this connection with the sorghum vasculature, it's like a superhighway of nutrients to the parasitic plant,” said Brady.
Brady and Kawa wanted to know whether soil microbes could interrupt this hijacking. Previous studies have shown that a species of soil fungus, Fusarium , suppresses witchweed germination, and thus infection of sorghum, but little is known about whether soil bacteria or fungi impact witchweed infections by changing the sorghum root.
As a first test, the researchers compared the susceptibility of sorghum seedlings that had been sprouted in “natural” soil to seedlings grown in sterilized, bacterially-depleted soil. When the researchers examined the sorghum plants several weeks later, they found that plants grown in natural soil had fewer witchweed hangers-on than those grown in sterilized soil, suggesting that bacteria play an important role in the plants’ ability to resist infection.
Microbial mechanisms
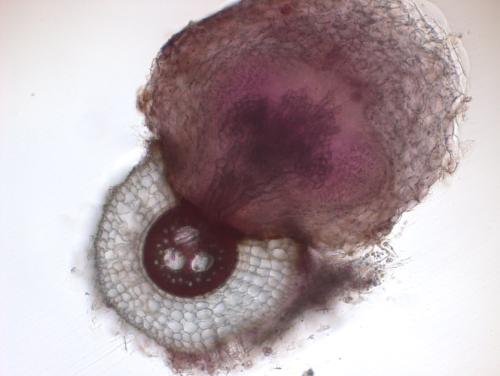
Next, the team wanted to investigate the mechanisms behind this resistance. Using a combination of genetics, microscopy and in vitro experiments, they showed that microbes degrade the chemical cues that help witchweed attach to its host and also alter the sorghum’s root anatomy to make it harder for witchweed to latch on. They observed that when sorghum plants are grown in natural, microbe-laden soil, the bacteria induce genes that result in a thicker layer of suberin, a waxy substance that may act as a barrier to witchweed, and more air-filled gaps or “aerenchymas” that may also impede witchweed’s attachment to sorghum.
Using genetic sequencing, Brady and her collaborators identified over 100 bacteria taxa that were associated with witchweed resistance. When they tested the functions of eight of these bacterial strains in vitro , they identified a strain of Pseudomonas bacteria that degrades chemical cues in the soil and a strain of Arthrobacter that increased root suberization in sorghum.
“It's exciting that we were able to identify individual microbes because typically you have a whole suite of microbes within the soil and it’s possible that they're acting together,” said Brady.
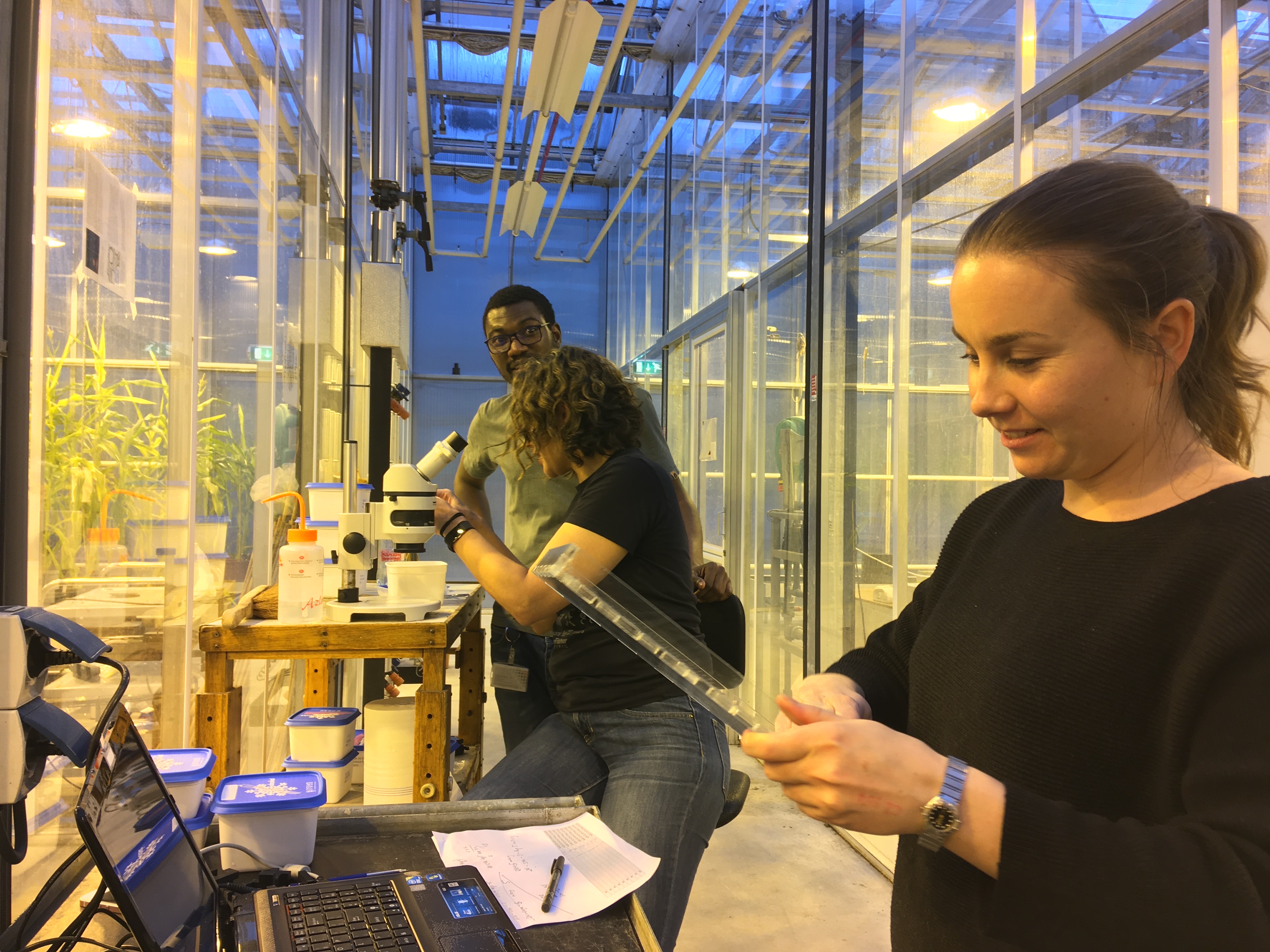
Another tool in the toolkit
“The ultimate goal is to identify microbial solutions that farmers can treat the soil or seeds with to help prevent Striga infection,” Brady said. “The intention is that this should be part of an integrated package of solutions to farmers—another tool in the toolkit.”
Now, the researchers are searching for microbes responsible for conferring the other resistance traits, such as aerenchyma formation. They’re also characterizing soil microbes from other regions, beginning with Ethiopia, and investigating whether these same microbes can confer witchweed resistance to other crop species that are also impacted by the parasite.
“We need to make sure that we're using microbes that come from the country in which those microbes will be applied so that we maintain biodiversity,” said Brady. “We also want to prioritize microbes that are able to work well in other crop species, like pearl millet and rice.”
Kawa is now an assistant professor at Utrecht University.
Additional authors on the study are: Tamera Taylor, Hannah Vahldick, Zayan Musa, Alexander Chen, and Jiregna Daksa, UC Davis; Desalegn Etalo, Utrecht University; Benjamin Thiombiano, Aimee Walmsley, Harro Bouwmeester, and Mario Schilder, Swammerdam Institute for Life; Mahdere Shimels, Dominika Rybka, Marcio Leite, Francisco Dini-Andreote, Eiko Kuramae, and Jos Raaijmakers, Netherlands Institute of Ecology; Alexander Bucksch, University of Georgia; and Taye Tessema, Ethiopian Institute of Agricultural Research.
The work was supported by the Bill and Melinda Gates Foundation, the Howard Hughes Medical Institute, the Advanced Research Projects Agency–Energy, and the National Science Foundation.
Media Resources
- The soil microbiome modulates the sorghum root metabolome and cellular traits with a concomitant reduction of Striga infection: Cell Reports
- Liana Wait is a freelance science writer based in Philadelphia. She has a Ph.D. in ecology and evolutionary biology and specializes in writing about the life sciences.
Primary Category
Thank you for visiting nature.com. You are using a browser version with limited support for CSS. To obtain the best experience, we recommend you use a more up to date browser (or turn off compatibility mode in Internet Explorer). In the meantime, to ensure continued support, we are displaying the site without styles and JavaScript.
- View all journals
- My Account Login
- Explore content
- About the journal
- Publish with us
- Sign up for alerts
- Perspective
- Open access
- Published: 21 August 2023
Perspectives for plant biology in space and analogue environments
- Veronica De Micco ORCID: orcid.org/0000-0002-4282-9525 1 ,
- Giovanna Aronne 1 ,
- Nicol Caplin 2 ,
- Eugénie Carnero-Diaz 3 ,
- Raúl Herranz ORCID: orcid.org/0000-0002-0246-9449 4 ,
- Nele Horemans 5 ,
- Valérie Legué ORCID: orcid.org/0000-0003-2090-9580 6 ,
- F. Javier Medina ORCID: orcid.org/0000-0002-0866-7710 4 ,
- Veronica Pereda-Loth 7 ,
- Mona Schiefloe 8 ,
- Sara De Francesco ORCID: orcid.org/0000-0001-8280-4237 1 ,
- Luigi Gennaro Izzo ORCID: orcid.org/0000-0001-5722-2497 1 ,
- Isabel Le Disquet 3 &
- Ann- Iren Kittang Jost 8
npj Microgravity volume 9 , Article number: 67 ( 2023 ) Cite this article
2182 Accesses
Metrics details
- Cell biology
- Plant sciences
Advancements in plant space biology are required for the realization of human space exploration missions, where the re-supply of resources from Earth is not feasible. Until a few decades ago, space life science was focused on the impact of the space environment on the human body. More recently, the interest in plant space biology has increased because plants are key organisms in Bioregenerative Life Support Systems (BLSS) for the regeneration of resources and fresh food production. Moreover, plants play an important role in psychological support for astronauts. The definition of cultivation requirements for the design, realization, and successful operation of BLSS must consider the effects of space factors on plants. Altered gravitational fields and radiation exposure are the main space factors inducing changes in gene expression, cell proliferation and differentiation, signalling and physiological processes with possible consequences on tissue organization and organogenesis, thus on the whole plant functioning. Interestingly, the changes at the cellular and molecular levels do not always result in organismic or developmental changes. This apparent paradox is a current research challenge. In this paper, the main findings of gravity- and radiation-related research on higher plants are summarized, highlighting the knowledge gaps that are still necessary to fill. Existing experimental facilities to simulate the effect of space factors, as well as requirements for future facilities for possible experiments to achieve fundamental biology goals are considered. Finally, the need for making synergies among disciplines and for establishing global standard operating procedures for analyses and data collection in space experiments is highlighted.
Similar content being viewed by others
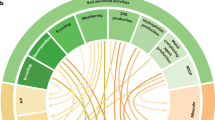
The interplay between microbial communities and soil properties
Laurent Philippot, Claire Chenu, … Noah Fierer
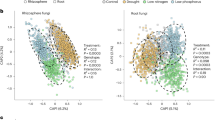
Heritable microbiome variation is correlated with source environment in locally adapted maize varieties
Xiaoming He, Danning Wang, … Peng Yu
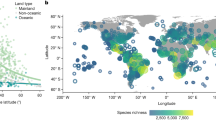
Mutualisms weaken the latitudinal diversity gradient among oceanic islands
Camille S. Delavaux, Thomas W. Crowther, … Evan M. Gora
Introduction
At the beginning of space exploration, research in space life science was focused on the human body to gain a fundamental understanding on the responses to the stressful space environment, to ultimately prevent health risks and protect astronauts by managing space-induced pathological issues 1 , 2 . In the last decades, increasing interest has been raised towards plant space biology due to the awareness that cultivation of higher plants in space is a requirement for long-duration human missions, where the regeneration of resources and plant-based food production onboard must be increased at the expense of re-supply from Earth 3 .
Plants play a crucial role in Bioregenerative Life Support Systems (BLSS). Artificial ecosystems such as the BLSS are high-technology systems, based on the integration of physico-chemical and biological processes, to support long interplanetary missions 4 , 5 . The BLSS concept includes several interconnected compartments in which different organisms are used to sequentially recycle resources 6 , 7 . Within a BLSS, the photoautotrophic compartment enables the production of edible biomass, oxygen, and water as resources for the astronaut, starting from carbon dioxide, wastewater and other wastes. An example of BLSS is the MELiSSA (Micro-Ecological Life Support System Alternative) loop by the European Space Agency (ESA) that aims to sustain astronaut life in space missions to reduce the initial payload and dependency from Earth 8 . The MELiSSA loop is made of interconnected compartments among which the requirements of the higher plant compartment need to be fulfilled at least in part by the outputs of other compartments 9 . As well, the species/cultivar choice and environmental control are dictated by the need to meet the requirements of the other compartments (e.g., oxygen requirements of the animal crew) in addition to the generally accepted requirements including short cultivation cycles, reduced plant size, high harvest index, and resistance to diseases. Increasing effort is posed on plants to produce healthy food specifically designed to sustain crew in long term missions 3 , 10 , 11 .
Additionally, research in space-plant biology and space-agriculture is required alongside the strong efforts to increase the knowledge on human biology in space with the aim to predict risk factors, prevent disease(s), and implement effective countermeasures to manage health emergencies during missions. Among countermeasures for assuring astronauts' well-being against degenerative diseases and psychological issues, there is the introduction, in their nutrition, of plant-derived fresh food produced directly on board or within a habitat. Further, there may also be benefits for crew mental health by allowing crews to participate in plant cultivation in the living and/or working pressurized modules in space 12 , 13 .
What are the main constraints to plant cultivation in space?
From an applied point-of-view, the design and realization of BLSS require a better understanding of plant acclimation and adaptation processes that determine the plant’s capability to complete a full life cycle in space (producing viable offspring) for both model plants and crops. As a matter of fact, the altered growth and behaviour of plants in space can alter the input/output balance between the different compartments as well as the potential nutritional value of the derived fresh-food. Indeed, as any other living organism, plants’ survival and reproduction strongly depend on interactions with environmental factors (e.g. temperature, light, oxygen/carbon dioxide, water, and volume availability) including the novel space factors (e.g., altered gravity and ionizing radiation). Among different environmental factors, altered gravity and ionizing radiation are recognized to be the main constraints for growth in space conditions. In the following section, we briefly summarize the current knowledge about the effects of microgravity and radiation on plants.
What is already known about the effects of microgravity and radiation
To date, European scientists studying plant space biology have focused on understanding the effects of altered gravity on several plant systems (e.g. different species/cultivars, target organs/tissues). Recently, more attention has been dedicated to the effect of ionizing radiation (IR) so far as it represents a clear challenge for exploratory-class missions 14 . Altered gravity and exposure to IR can induce changes in gene expression, cell proliferation and differentiation, signalling and physiological processes (see precise data and references in the following paragraphs). The expected consequence of these changes would be serious structural and functional alterations at the whole organism level. In addition, since these cellular and molecular changes are detected in early developmental stages, developmental alterations would also be expected. However, altered tissue organization, organogenesis and functioning have not always been detected under spaceflight conditions. For example, none of these effects has been reported to occur when plants have been cultured in space: adult organisms were produced with no evident aberrations and the entire seed-to-seed life cycle of plants was successfully achieved under spaceflight conditions 15 , 16 . Interestingly, this phenomenon is not exclusive to plants. It was first detected and reported by Marco et al. 17 in the fruitfly Drosophila melanogaster and defined as “an apparent paradox”. Up to now, this paradox has not been resolved and remains an important challenge for space biology research.
Effect of microgravity and altered gravity on plant growth and reproduction
Since 475 million years ago, land plants have evolved under the same 1 g gravity level. Early pioneering space experiments have demonstrated that plants are able to survive and grow in space, although morpho-physiological alterations were soon reported in crop species as lettuce and lentil 18 , 19 . In early research, deficiencies in the experimental setup and in used facilities were sometimes responsible for confusing and contradictory results. For example, nutrient absorption has been reported to increase, decrease or remain unchanged under reduced gravity, likely due to hardware limitations and species-specific responses 20 . Now, however, there is evidence that microgravity in space environment does not prevent plant growth and reproduction but causes serious alterations in plant physiology and development 21 . Plants respond to changes in mechanical inputs, therefore gravity-related research offers a unique opportunity to elucidate the mechanisms of gravity perception whose knowledge is necessary to better understand fundamental processes and the physiological changes in space. Moreover, in exploratory-class long-term missions, plants will face not only a lack of gravity (or residual gravity) during spaceflight (and inside the International Space Station - ISS) but also the lunar gravity (0.17 g ) and the Martian gravity (0.37 g ) which are substantially lower to what plants normally experience on Earth. Indeed, in forecasting possible acclimation of plants in environments with altered gravity, thresholds for gravity sensing should be considered. Previous studies indicated that the threshold acceleration perceived by lentil roots in spaceflight can be in the order of 10 –3 g or lower 22 , but the effects of altered gravity on cell cycle have been detected at higher levels, intermediate between Moon and Mars gravity, in simulation experiments on Earth 23 . Similarly, the range for the attenuation of phototropism in higher plants occurs in the same range 0.1–0.3 g 24 .
Plant developmental patterns and gravity perception: from gene expression to cell cycle and organ development
Most studies to reveal the effect of altered gravity on plant development have been focused on the root meristem 25 . Experiments under microgravity simulation and onboard the ISS revealed the disruption of the meristematic competence in seedlings, i.e. the loss of the coordinated progress of cell proliferation and cell growth that characterizes meristematic cells under gravity conditions on Earth 26 , 27 , 28 .
An acceleration in the cell cycle was detected in in-vitro cultured Arabidopsis MM2d cells grown in simulated microgravity conditions produced in a Random Positioning Machine (RPM), resulting from downregulation of genes involved in the G2/M transition checkpoint and upregulation of genes controlling the G1/S transition. Other phenomena were the downregulation of significant genes for ribosome biogenesis and the corresponding depletion of the levels of nucleolar proteins, the depletion of the nuclear transcription and an increase in chromatin condensation, related to the epigenetic regulation of gene expression 29 , 30 . Experiments using different levels of reduced gravity and hypergravity indicated modulation of alterations for each level, with Mars gravity inducing milder alterations 23 , 31 . Indeed, studies on gravitropism and phototropism have shown that the reduced gravity level on Mars of 0.38 g should not be a major problem for plant growth 24 .
Auxin is a key factor regulating the connection between perceived stimuli and cellular responses, controlling the balance between cell proliferation and cell differentiation, regulating the cell cycle progression and the coordination between cell growth and cell division 32 , 33 , 34 , 35 . The role of the LAZY proteins in gravity sensing has been recently described, being crucial players linking gravity perception and gravitropic curvature through the proper redistribution of auxin, the relocation of the auxin efflux carrier PIN-FORMED (PIN) proteins for the tropic response of both roots and shoots 36 . However, the role of auxin and its polar transport in plant growth and development under microgravity conditions is not fully understood and requires further investigation to solve controversial concerns due in part to its complex interaction with cytokinin. For example, it has been shown in Arabidopsis that real microgravity does not influence the distribution of auxin in the primary root, whereas it affects that of cytokinin 37 . In contrast, the inhibition of pea hypocotyl growth in microgravity is correlated with an attenuation of polarized auxin transport, a decrease in auxin levels and an increase in cytokinin levels 38 .
Parallel transcriptomic experiments exploring how simulated (i.e. clinorotation, RPM and diamagnetic levitation) and real microgravity change gene and protein expression have shown a complex response of plants at early developmental stages (mostly Arabidopsis seedlings), involving reprogramming of the gene expression pattern 39 . Specific genes of response to gravity alteration have not been found, while the main and most frequent targets of this gene reprogramming are: genes coding for heat shock-related elements, cell wall remodelling factors, oxidative burst intermediates and components of the general mechanisms of plant defence against stressors, being differently affected at the different spaceflight, lunar and martian g levels 40 . The results of several experiments in space using a centrifuge to produce different gravity levels showed a differential response to each level, triggering different adaptive responses, involving changes in the regulation of different sets of genes. Changes in gene expression were lower under Mars gravity compared to microgravity on the ISS 40 , 41 .
Interaction with other factors
Many plant responses are primed by the interaction between gravity and other physical, chemical, or biological factors. In the last decades, orbital platforms enabled comprehensive studies on the mechanisms underlying plant growth in microgravity 42 . Still, there is a need to further investigate the interactions between gravity and other environmental factors including temperature, light, oxygen/carbon dioxide, water availability, electric and magnetic fields, especially in the framework of plant morphogenesis and tropic responses, also considering intra-specific genotypic variability 43 , 44 . With the exception of a limited number of studies concerning microgravity interactions with magnetic fields, water or chemical stimuli on crops (i.e. flax, cucumber and carrot) 45 , 46 , 47 , previous research has mainly focused on the interactions between gravity and light. It has been shown that the sensitivity of plants to light is influenced by microgravity and that phototropic curvature of the shoot and root organs are largely affected by changes in gravity conditions 48 Experiments performed using the EMCS onboard the ISS or the ESA ground-based facilities resulted in discovering novel phototropic responses of plants, both the model Arabidopsis and Brassica oleracea , proving that the interaction between gravity and light changes according to the magnitude of g-force 49 , 50 , 51 . In this framework, light quality has a prominent role in determining the direction and strength of phototropic responses of shoots and roots, with major differences between blue and red wavelengths under a wide range of gravity levels 51 , 52 . On the other hand, previous studies also showed that light can control the sensitivity of plants to gravity through phytochrome-regulated pathways, indicating that phytochromes play a key role in integrating multiple environmental stimuli 53 .
Gene expression studies with different levels of altered gravity showed that the adaptive response appeared enhanced by red light photostimulation. Red light activates cell proliferation and ribosome biogenesis in pea 54 , and in the “Seedling Growth” series of experiments in the ISS, red light caused a concerted upregulation of marker genes for cell proliferation, cell growth and auxin polar transport 55 . Experiments with different levels of gravity (microgravity, Mars gravity and ground control gravity) with and without red light photoactivation have shown that red light restored the auxin distribution patterns which appeared altered under microgravity, while in roots grown at 0.3 g , the auxin polar transport was slightly altered, irrespective of photoactivation 56 . The red light was also shown to counteract the decoupling between cell proliferation and growth in root meristems reported in earlier experiments 41 .
Gene expression alterations, evaluated by RNA-seq, showed different responses to different gravity levels and modulation of gene expression by red light photoactivation. As an example, Mars gravity level induced an adaptive response, consisting of the activation of environmental acclimation-related transcription factors (WRKY and NACs families), especially in photostimulated samples 41 .
It appears clear that plant cultivation in future space missions implies research-based strategies that involve gravity-substituting factors (e.g., light) to counteract the effects of microgravity or partial gravity conditions such as on the Moon and Mars. Nowadays, the technological advancement in cultivation systems is providing effective and affordable tools to control environmental factors for plant growth in space with the possibility of using external cues for both application and research purposes 42 . Gravity might be replaced by specific stimulation in terms of light wavelengths and photon flux density for the regulation of plant growth and development. Prospectively, other factors are expected to play such a role including water, electric and magnetic fields, chemicals, or microorganism, but further investigation is needed.
Seed-to-seed cycle
Nowadays, it is crucial to delve into the mechanisms by which altered gravity conditions can affect plant reproduction and seed viability, in order to develop cultivation strategies for the improvement of plant-based BLSS in future human settlements on the Moon and Mars 57 . The completion of the seed-to-seed cycle, in fact, will be essential to produce viable seeds to be used for the cultivation of plants over time without relying on terrestrial supply.
Early studies with plants grown for extended periods in microgravity reported an overall reduction of plant growth and difficulties in the transition to the reproductive stage 58 . Since the first seed production in space by Arabidopsis thaliana plants in 1982, a few experiments on plant reproduction have been performed 59 . Overall, previous studies showed that the seed-to-seed cycle can be accomplished in most species tested in microgravity, although with reduced quality of embryos and seeds produced by plants due to delayed embryo development, modification in storage reserves, delayed starch use in cotyledons, and decreased cell number in cotyledons 15 , 58 , 60 , 61 , 62 . Furthermore, experiments using simulated microgravity (e.g., clinorotation) showed significant alterations during the development of male gametes in several crop species 63 .
Given that most studies have investigated the effect of microgravity on early stages of plant development with sporadic studies on plant reproduction, there is a large gap of information to be filled to fully the effects on the growth of plants in the adult stage and on plant reproduction.
Effects of ionizing radiation on plant growth and development
Outside Low Earth Orbit, IR is variable in space and time and can severely constrain organisms’ growth 3 , 14 . IR can cause direct damage to the structures encountered, but also indirect due to the generation of reactive oxygen species (ROS) 64 , 65 . The oxidative stress due to ROS production may damage important components of plant cells, including lipids and proteins, but especially DNA 66 , 67 . The degree of direct DNA damage and proper functioning of DNA repair systems determine the consequences of IR exposure for plants at morpho-structural and physiological level 68 .
Nevertheless, plants’ responses to IR are not fully understood yet. Experiments in space where plants, either the model Arabidopsis or crops such as beans and tomato, were exposed to cosmic radiation and on the ground with exposure to low- and high- linear energy transfer (LET) radiation have shown that IR can have positive, null or negative effects on plants, at genetic and morphophysiological levels depending on IR properties and plant intrinsic factors such as type of radiation, its LET, exposure time (acute or chronic), dose, plant species/cultivar, developmental stage at the time of irradiation 69 . The effect of IR is also tissue-specific and depends on tissue architecture: complex tissues in beans and tomatoes seem less sensitive to damage 70 , 71 , and, on the contrary, the meristematic cells are the most sensitive to radiation 72 .
High-LET radiation, like protons and heavy ions, is more harmful in inducing genetic mutations compared to low-LET radiation such as X- and γ-rays 73 , 74 . Concerning the dose of exposure, high doses (>100 Gy for seeds; >50–70 Gy for vegetative stages) can lead to harmful outcomes, such as reduced levels of photosynthesis and germination, embryo lethality, loss of apical dominance, dwarf architecture, altered leaf anatomy, and accelerated senescence 67 , 69 , 75 . Low doses of IR, on the other hand, appear to induce hormetic response in plants, stimulating germination, growth, photosynthetic and respiration rate, improving the content in chlorophyll, carotenoids, non-enzymatic (ascorbic acid, glutathione, and anthocyanin) and enzymatic antioxidants (ascorbate peroxidase, catalase, and superoxide dismutase) and phenolic compounds, effective in counteracting the oxidant action of ROS, thus increasing plant nutritional value and radioresistance 75 , 76 , 77 , 78 , 79 , 80 .
Most of the studies performed on plants have been conducted by irradiating dry seeds with acute doses (due to limitations in the volume and time of irradiation availability) 75 , 81 . Moreover, most of the studies involving crop species have mainly been focused on using IR to introduce genetic variation and selecting plant cultivars with specific traits 82 . Only a few studies have considered the effects on the yield, nutritional value as well as interaction with other factors. A recent study has indicated that the effect of X-rays delivered to germinated seeds at different doses is strongly influenced by light quality during subsequent cultivation 83 .
As a result, little information is available on the variation of radiosensitivity during the different phenological phases in the case of acute exposure and on the effects of chronic exposure. Indeed, it is important to emphasize that resistance to large doses of radiation delivered in an acute way (in shorter times than those necessary for the repair of cellular damage) often does not translate into resistance to chronic exposures for multiple generations and vice versa 84 . Therefore, in the sight of future space exploration, the time is ripe for increasing the efforts to investigate plant responses to chronic low dose-rate and high LET radiation to clarify all the processes and mechanisms behind the radioresistance phenomenon 14 .
Knowledge gaps in microgravity and radiation research in plant biology
According to the current scientific knowledge on plants’ responses to space factors, in order to successfully achieve the in-progress target of crewed missions in space, it is necessary to fill knowledge gaps in plant biology. They can be synthesized in the following five points (Fig. 1 ).
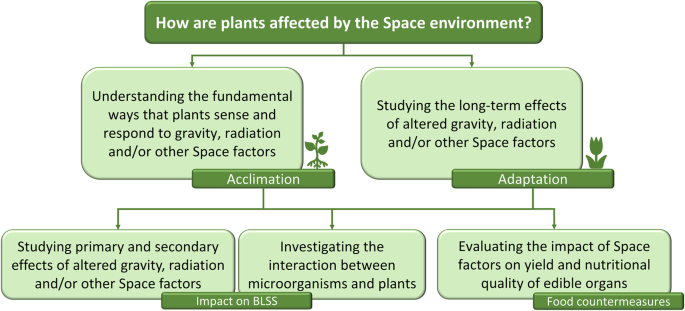
Understanding such processes is fundamental to evaluate the impact on the functioning of BLSS and on the value of plant-derived food for the integration of astronauts’ nutrition.
1 - Understanding the fundamental ways that plants sense and respond to gravity alone or in combination with radiation and other space and environmental/cultivation factors. This point is mainly related to short-term effects and acclimation strategies and includes studies on tropisms and morphogenesis.
2 - Studying the long-term effects of altered gravity, radiation and/or other space environment factors on plants and understanding how plants adapt to this new kind of environment.
3 - Studying primary and secondary effects of altered gravity, radiation and/or other space factors on plant growth and reproduction.
4 - Investigating the interaction between microorganisms and plants (beneficial and pathogenic) under space conditions in light of the realization of cultivation modules in BLSS.
5 - Investigating the effects of space factors on yield and nutritional value and quality (e.g. production of nutraceutical compounds) of edible organs targeting the use of plant-derived fresh food as countermeasures to improve astronauts’ health.
Points 3–5 can be investigated in the short-period, thus being mainly targeted to unravelling the acclimation strategies of plants to space factors. The same points can be investigated in the long-term and over multiple generations to evaluate the heritability of varied traits, hence leading to adaptation. In the latter case, constraints to reproduction become crucial to be analyzed.
Overall, the main objective of research activities in plant space biology is to reveal potential acclimation and adaptation mechanisms and processes in the response of plants (crops and model species) to microgravity, partial gravity and variable space radiation in combination with other environmental/cultivation conditions (e.g. airflow, light), through the developmental phases of a whole life cycle. This will allow the resolution of the “apparent paradox” between molecular and cellular effects versus organismic and developmental effects by understanding the mechanisms by which plants overcome the impacts occurring at early plant life stages after exposure to spaceflight conditions.
To understand if exposure to adverse space conditions potentially leads to acclimation and adaptation, the long-term responses of plants have to be investigated through sequential studies of plants after different times of exposure to single or multiple space factors, at different phases of the plant development. The acclimation has to be studied during the life cycle of the plant and adaptation has to be studied after several generations of plants exposed to a space environment.
Knowledge of plant-microorganism interactions is also important. Plants naturally attract microorganisms, some detrimental to plant health while others establish symbiotic relationships. Plants also have endophytes (bacteria and fungi) living between plant cells, some of which are transmitted to the following generations. Understanding the effect of the space environment on the relations between microorganisms and plants can help assess risks to future crew food supply or discover opportunities for microorganisms-mediated enhanced crop yield.
Unravelling the plant acclimation and adaptation processes, responsible for producing essentially viable adult individuals, is important not only for our fundamental understanding within plant biology but also for the realization of BLSS.
Indeed, addressing the five points mentioned above would allow to evaluate:
How the growth processes and regeneration capacity of plants are affected by space factors and thus impact the cultivation requirements in producers’ modules of BLSS.
If reproductive success is achieved and whether multiple generations of plants are possible to obtain, in order to guarantee the possibility to produce seeds for successive cultivation cycles.
If and how the yield and nutritional value and quality of edible organs are affected and thus impact the astronauts’ nutrition.
The two first points regard every plant species, not only crops but also model species such as Arabidopsis , while the second and third mainly refer to crop species.
Facilities in space and on Earth to study the effects of microgravity and radiation on plants
The ISS is an important research platform to study not only the effects of reduced gravity but also the long-term consequences of low dose space radiation on plants. The daily dose received in the ISS has been estimated at 0.5 mSv, assessed by physical dosimetry using phantoms 85 , which is about 100 times higher than the dose on Earth, and about 2.6 and 1.28 times lower than the dose on the Moon and Mars surface 86 , 87 . By combining studies on ISS of plants exposed to various space factors (gravity/space radiation) and environmental cultivation conditions (e.g. different airflow or light conditions), additional required knowledge for future space agriculture can be obtained. Long-term experiments and full cycle studies of plants require a minimum cultivation area. Although the growth area has become larger in the newer ISS facilities (compared to systems such as Kubik and Icecubes), they are still considered too small for crops and full life cycles. When a 1 g or simulated Moon/Mars gravity exposure is required, the growth area is limited due to the diameter of the centrifuge rotor that has to fit into standard-sized racks on the ISS. An extensive review was done on the space plant growth systems where more than 20 systems are described 42 . The ESA BIOLAB facility on ISS has 4 Advanced Experiment Containers (AEC) on two rotors with a limited growth area per AEC ( http://wsn.spaceflight.esa.int/docs/Factsheets/8%20Biolab%20LR.pdf ) (Fig. 2 ) (Table 1 ). The BIOLAB allows unique experimental equipment to be built inside the AEC, and the available growth area for the plant will depend on the instrumentation required in the AEC to perform the experiment but still remains limited. The NASA systems such as Vegetable Production System (VEGGIE, 2014) and Advanced Plant Habitat (APH, 2017) have increased crop growth area, but they are still relatively small 88 , 89 , 90 . The APH is a closed, controlled growth system with full environmental monitoring and control. The VEGGIE system is simpler, with less control, and designed for more crew interaction (Table 1 ). For plant experiments where smaller volumes are required, the NASA Advanced Biological Research System (ABRS) is available with two experimental research chambers (growth area 0.053 m 2 ). The chamber has light and environment control, and one of the two chambers is outfitted with Green Fluorescent Protein Imaging System. The JAXA Plant Experiment Unit (PEU), available on the Kibo laboratory on ISS, is equipped with a LED lighting system with red and blue LEDs, a growth chamber (growth area 0.027 m 2 ), an automated watering system and a CCD camera.
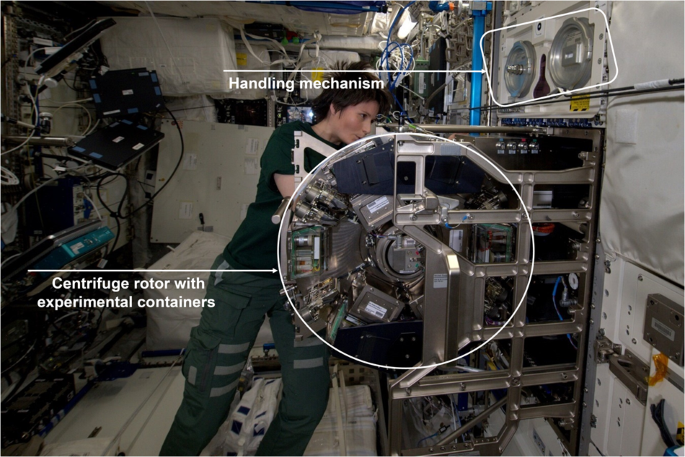
It appears evident the presence of the centrifuge but the strict limitations of volume available for the experimental containers. Credits: ©ESA/NASA.
What is further required?
Larger growth areas, where both model plants and crop plants can be cultivated for long-term experiments (during the whole life cycle), are needed to fully understand the plant acclimation and adaptation to the space conditions. Such a facility requires the control of environmental factors (including temperature, light, gasses, relative humidity, airflow, nutrient solution, and watering), and continuous imaging of the plant growth (e.g., visible light images, IR images using thermal cameras, fluorescence images). Environmental and imaging data should as a minimum be partially and periodically downloaded for feedback from the science teams collaborating from the ground. To collect data for the molecular, cell and anatomical studies, plant samples need to be harvested after sequential exposures to reduced gravity, preserved on ISS either by freezing or chemical fixation and brought back to the ground for analyses. In addition, the possibility to make microscopy analyses onboard has become a reality with the ESA/DLR Fluorescence Microscopy Analysis System (FLUMIAS), a high-resolution fluorescence microscope for live-cell imaging that is available on the ISS 91 .
A specific point affecting the technical needs of space facilities for plant culture is the possibility of performing comparative studies in spaceflight at different levels of gravity, including the Moon and Mars gravity, as well as the inflight 1 g control. The latter is of the highest importance and makes mandatory the implementation of centrifuges in the facilities used for plant cultivation in the ISS. The advanced sophisticated facilities for plant cultivation now available on ISS, such as VEGGIE, APH and the Exposed Roots On-Orbit Test System (X-ROOTS), which have proven successful in the cultivation of a wide range of plant species, are not equipped with centrifuges. ESA in 2018 decommissioned the European Modular Cultivation System (EMCS), a highly useful facility in which different successful experiments were carried out, some of them including pioneering comparative analyses at different levels of gravity in addition to the necessary in flight 1 g controls. Later on, in 2020, ESA promoted a discussion group to adapt the ESA Biolab facility to harbour experiments using crop species and encompassing the full plant life cycle while being exposed to different gravity levels. Whatever the final decision adopted, the need for an effective plant cultivation facility in space with the capabilities mentioned above, as a key to gaining knowledge to support human life in space exploration, is becoming more and more urgent within the next years.
Applications and benefits for Earth
Understanding how plants are able to grow and adapt to space conditions will ensure reliable and predictable food supplies for human space exploration and has strong synergies with the United Nations Sustainable Development Goals, global food security and circular economy.
The sophisticated agro-technologies developed for space applications (e.g., innovative lighting, watering and nutrient delivery systems, fine environmental monitoring with automated control, imaging systems to analyze plant health, etc.) bring innovations to agriculture on Earth to improve sustainable plant cultivation and food production. For example, developing volume-saving, highly efficient plant growth controlled environments is beneficial for food and drug production particularly in densely populated urban areas in line with vertical farming technologies, in underground facilities with no natural light source, and in general in extreme environments such as deserts and poles.
Developing smart and safe pest control methods is applicable in confined volumes where aerosols are undesirable and natural predation for the reduction/removal of pests is not possible.
Besides these more human operational and exploration-oriented goals, it should also be stressed that the unique near weightlessness environment, as well as the high levels of ionizing radiation, also provide a research laboratory that cannot be obtained in on-ground laboratories and as such can answer specific and fundamental questions in life sciences. Improving the knowledge on how plants respond to ionizing radiation can provide information applicable in all the fields in which radiation is studied on Earth ranging from breeding programs, decontamination methods and radioecology.
Future perspectives and recommendations in the short and long term
Today there is a need for fundamental research that goes beyond the demonstration of plants’ ability to acclimate and adapt to the space environment. A multi-parameter facility would help unravel the effects of altered gravity in combination with other factors, either typical of space (i.e. ionizing radiation) or of confined volumes of cultivation chambers. Moreover, by modulating the exposure to specific environmental conditions (e.g., air flow or light), it would be possible to study the direct and indirect effect of the space factors on plants, bridging the knowledge gaps of the acclimation/adaptation mechanisms.
Currently, to study plant development in the space environment through the whole life cycle of a plant, including crops/food plants, is possible only using the ISS research platform: no other active platforms possess capabilities for answering the plant biology research questions listed in this paper. The infrastructure present on ISS can be modernized to achieve the needed goals to point to the sustainability of space exploration with BLSS.
Using the ISS platform for research will be the defining stepping stone into exploratory-class missions deeper into space. International efforts are ongoing to design and develop additional payloads in the frame of the Artemis program (e.g., NASA PRISM solicitation) ( https://www.nasa.gov/feature/nasa-releases-prism-call-for-potential-lunar-surface-investigations ). The Gateway platform is also currently planned for lunar orbit in the mid-late 2020 s. This should provide opportunities for experiments deeper into space, particularly outside of Earth’s magnetic field and the unique radiation environment can be exploited to further develop our understanding of the effect that different types of radiation may have on plants in combination with other space factors.
It is not straightforward to indicate what are the more urgent goals of plant space biology since it has been recognized that space farming is becoming more and more a necessity as long as the roadmap for human space exploration goes beyond LEO (BLEO). A summary of possible goals (targets) within the main knowledge gaps (open fundamental scientific questions) identified in the previous paragraphs is reported in Fig. 3 .
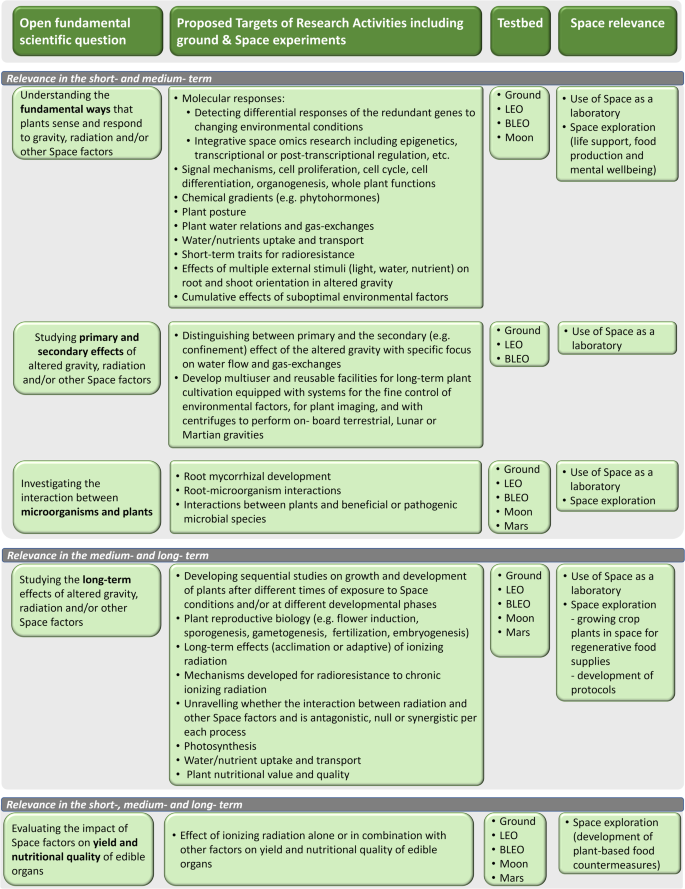
Other research platforms such as ground, Moon, Mars, LEO and BLEO (beyond LEO) are also included. They represent both the basis for the research on ISS and future research activities post-ISS (e.g. the GATEWAY orbiting the Moon).
The first, second and third knowledge gaps should be addressed in experiments to be performed in the short- and medium-term since they will provide fundamental information that is the basis for the realization of more complex experiments in the long-term. The knowledge obtained in the short- and medium-term will be fundamental to defining requirements and developing new hardware to support long-term exploratory-class human missions. Indeed, in such missions, completely closed BLSS are themselves the “main requirement” although with technological differences depending on the scenarios determining the environmental constraints and mission duration.
In conclusion, to achieve successful space exploration, it is fundamental to apply an integrative approach in space biology merging together the information gained at different biological levels (e.g. molecular, cellular, tissue/organ up to the whole individual) as well as integrating knowledge among organisms (producers, consumers and degraders) also to understand the effect of space factors on their capability of networking in the artificial ecosystem as in nature on Earth. Space biology has been historically divided into sub-disciplines dealing with animals/mammals, microbes and plants without much interaction. However, some processes of altered metabolism as those regarding DNA-repair mechanisms as well as ROS and peptide signaling as stress responses are well conserved among species (including animals and plants). This paves the way towards the need for making synergies among disciplines to achieve an integrated picture of common vs distinct responses to space factors in different organisms. Possibly, the establishment of global standard operating procedures for space omics (including metagenomics) data sets generation and annotation, including but not limited to those generated within the framework of ESA and NASA projects, can allow the expansion of statistical power in space flight experiments by means of the federation of data sets.
Indeed, it has been recognized that to achieve BLSS operating in space and guarantee better protection of human health and well-being in space, an integrated, multidisciplinary approach linking together different branches of life science (e.g. animal and human physiology, plant biology, microbiology, etc.) is needed, as well as the cooperation with physical sciences, technologies and engineering. Therefore, there is a need to bridge and strengthen the interconnections between them also through the design, proposal, and realization of new studies and experiments.
Patel, Z. S. et al. Red risks for a journey to the red planet: the highest priority human health risks for a mission to Mars. NPJ Microgravity 6 , 33 (2020).
Article PubMed PubMed Central Google Scholar
Afshinnekoo, E. et al. Fundamental biological features of spaceflight: advancing the field to enable deep-space exploration. Cell 183 , 1162–1184 (2020).
Article CAS PubMed PubMed Central Google Scholar
De Pascale, S. et al. Biology and crop production in Space environments: challenges and opportunities. Life Sci. Space Res. 29 , 30–37 (2021).
Article Google Scholar
De Micco, V., Aronne, G., Colla, G., Fortezza, R. & De Pascale, S. Agro-biology for bioregenerative life support systems in long-term space missions: general constraints and the Italian efforts. J. Plant Interact. 4 , 241–252 (2009).
Tamponnet, C. & Savage, C. Closed ecological systems. J. Biol. Educ. 28 , 167–174 (1994).
Gòdia, F. et al. MELISSA: a loop of interconnected bioreactors to develop life support in Space. J. Biotechnol. 99 , 319–330 (2002).
Article PubMed Google Scholar
Häder, D. P., Braun, M. & Hemmersbach, R. Bioregenerative life support systems in space research in Gravitational biology. In Gravitational Biology I. SpringerBriefs in Space Life Sciences (eds. Braun, M. Böhmer, M., Häder, D. P., Hemmersbach, R., Palme, K.) 113–122. (Springer, Cham, 2018)
Hendrickx, L. et al. Microbial ecology of the closed artificial ecosystem MELiSSA (Micro-Ecological Life Support System Alternative): reinventing and compartmentalizing the Earth’s food and oxygen regeneration system for long-haul space exploration missions. Res. Microbiol. 157 , 77–86 (2006).
Garcia-Gragera, D. et al. Integration of nitrifying, photosynthetic and animal compartments at the MELiSSA Pilot Plant. Front. Astron. Space Sci. 8 , 177 (2021).
Tang, H., Rising, H. H., Majji, M. & Brown, R. D. Long-term space nutrition: a scoping review. Nutrients 14 , 194 (2022).
Article CAS Google Scholar
Perchonok, M. H., Cooper, M. R. & Catauro, P. M. Mission to mars: food production and processing for the final frontier. Annu. Rev. Food Sci. Technol. 3 , 311–330 (2012).
Article CAS PubMed Google Scholar
Koga, K. & Iwasaki, Y. Psychological and physiological effect in humans of touching plant foliage - using the semantic differential method and cerebral activity as indicators. J. Physiol. Anthropol. 32 , 1–9 (2013).
Odeh, R. & Guy, C. L. Gardening for therapeutic people-plant interactions during long-duration space missions. Open Agric. 2 , 1–13 (2017).
De Micco, V., Arena, C., Di Fino, L. & Narici, L. Radiation environment in exploration-class space missions and plants’ responses relevant for cultivation in Bioregenerative Life Support Systems. Front. Plant Sci. 13 , 1001158 (2022).
Yano, S. et al. Improvements in and actual performance of the Plant Experiment Unit onboard Kibo, the Japanese experiment module on the international space station. Adv. Space Res. 51 , 780–788 (2013).
Khodadad, C. L. M. et al. Microbiological and nutritional analysis of lettuce crops grown on the International Space Station. Front. Plant Sci. 11 , 199 (2020).
Marco, R., Husson, D., Herranz, R., Mateos, J. & Medina, F. J. Drosophila melanogaster and the future of ‘evo-devo’ biology in space. Challenges and problems in the path of an eventual colonization project outside the earth. Adv. Space Biol. Med. 9 , 41–81 (2003).
Merkys, A. J., Laurinavičius, R. S. & Švegždiene, D. V. Plant growth, development and embryogenesis during Salyut-7 flight. Adv. Space Res. 4 , 55–63 (1984).
Perbal, G., Driss‐Ecole, D., Rutin, J. & Sallé, G. Graviperception of lentil seedling roots grown in space (Spacelab Dl Mission). Physiol. Plant. 70 , 119–126 (1987).
Wolff, S. A., Coelho, L. H., Zabrodina, M., Brinckmann, E. & Kittang, A.-I. Plant mineral nutrition, gas exchange and photosynthesis in space: A review. Adv. Space Res. 51 , 465–475 (2013).
Vandenbrink, J. P. & Kiss, J. Z. Space, the final frontier: a critical review of recent experiments performed in microgravity. Plant Sci. 243 , 115–119 (2016).
Driss-Ecole, D., Legué, V., Carnero-Diaz, E. & Perbal, G. Gravisensitivity and automorphogenesis of lentil seedling roots grown on board the International Space Station. Physiol. Plant. 134 , 191–201 (2008).
Manzano, A. I. et al. Novel, Moon and Mars, partial gravity simulation paradigms and their effects on the balance between cell growth and cell proliferation during early plant development. NPJ Microgravity 4 , 9 (2018).
Kiss, J. Z. Plant biology in reduced gravity on the Moon and Mars. Plant Biol. 16 , 12–17 (2014).
Herranz, R. & Medina, F. J. Cell proliferation and plant development under novel altered gravity environments. Plant Biol. 16 , 23–30 (2014).
Matía, I. et al. Plant cell proliferation and growth are altered by microgravity conditions in spaceflight. J. Plant Physiol. 167 , 184–193 (2010).
Manzano, A. I. et al. Meristematic cell proliferation and ribosome biogenesis are decoupled in diamagnetically levitated Arabidopsis seedlings. BMC Plant Biol. 13 , 1–15 (2013).
Boucheron-Dubuisson, E. et al. Functional alterations of root meristematic cells of Arabidopsis thaliana induced by a simulated microgravity environment. J. Plant Physiol. 207 , 30–41 (2016).
Kamal, K. Y., Herranz, R., van Loon, J. J. W. A. & Medina, F. J. Cell cycle acceleration and changes in essential nuclear functions induced by simulated microgravity in a synchronized Arabidopsis cell culture. Plant Cell Environ. 42 , 480–494 (2019).
Kamal, K. Y., van Loon, J. J. W. A., Medina, F. J. & Herranz, R. Differential transcriptional profile through cell cycle progression in Arabidopsis cultures under simulated microgravity. Genomics 111 , 1956–1965 (2019).
Kamal, K. Y., Herranz, R., van Loon, J. J. W. A. & Medina, F. J. Simulated microgravity, Mars gravity, and 2 g hypergravity affect cell cycle regulation, ribosome biogenesis, and epigenetics in Arabidopsis cell cultures. Sci. Rep. 8 , 1–16 (2018).
Muday, G. K. & Murphy, A. S. An emerging model of auxin transport regulation. Plant Cell 14 , 293–299 (2002).
David, K. M. et al. The auxin-binding protein 1 is essential for the control of cell cycle. Plant J. 50 , 197–206 (2007).
Perrot-Rechenmann, C. Cellular responses to auxin: division versus expansion. Cold Spring Harb. Perspect. Biol. 2 , a001446 (2010).
Medina, F. J. & Herranz, R. Microgravity environment uncouples cell growth and cell proliferation in root meristematic cells: The mediator role of auxin. Plant Signal Behav. 5 , 176–179 (2010).
Jiao, Z., Du, H., Chen, S., Huang, W. & Ge, L. LAZY gene family in plant gravitropism. Front. Plant Sci. 11 , 606241 (2021).
Ferl, R. J. & Paul, A.-L. The effect of spaceflight on the gravity-sensing auxin gradient of roots: GFP reporter gene microscopy on orbit. NPJ Microgravity 2 , 15023 (2016).
Yamazaki, C. et al. Comprehensive analyses of plant hormones in etiolated pea and maize seedlings grown under microgravity conditions in space: Relevance to the International Space Station experiment “Auxin Transport”. Life Sci. Space Res. 36 , 138–146 (2023).
Manzano, A., Carnero-Diaz, E., Herranz, R. & Medina, F. J. Recent transcriptomic studies to elucidate the plant adaptive response to spaceflight and to simulated space environments. iScience 25 , 104687 (2022).
Herranz, R. et al. RNAseq analysis of the response of Arabidopsis thaliana to fractional gravity under blue-light stimulation during spaceflight. Front. Plant Sci. 10 , 1529 (2019).
Villacampa, A. et al. From spaceflight to MARS G-levels: adaptive response of A. thaliana seedlings in a reduced gravity environment is enhanced by red-light photostimulation. Int. J. Mol. Sci. 22 , 899 (2021).
Zabel, P., Bamsey, M., Schubert, D. & Tajmar, M. Review and analysis of over 40 years of space plant growth systems. Life Sci. Space Res. 10 , 1–16 (2016).
Muthert, L. W. F., Izzo, L. G., van Zanten, M. & Aronne, G. Root tropisms: investigations on earth and in space to unravel plant growth direction. Front. Plant Sci. 10 , 1807 (2020).
Lionheart, G., Vandenbrink, J. P., Hoeksema, J. D. & Kiss, J. Z. The impact of simulated microgravity on the growth of different genotypes of the model legume plant Medicago truncatula . Microgravity Sci. Technol. 30 , 491–502 (2018).
Hasenstein, K. H., Scherp, P. & Ma, Z. Gravisensing in flax roots - Results from STS-107. Adv. Space Res. 36 , 1189–1195 (2005).
Morohashi, K. et al. Gravitropism interferes with hydrotropism via counteracting auxin dynamics in cucumber roots: clinorotation and spaceflight experiments. N. Phytol. 215 , 1476–1489 (2017).
Izzo, L. G. et al. Chemotropic vs hydrotropic stimuli for root growth orientation in microgravity. Front. Plant Sci. 10 , 1547 (2019).
Kiss, J. Z., Wolverton, C., Wyatt, S. E., Hasenstein, K. H. & van Loon, J. J. W. A. Comparison of microgravity analogs to spaceflight in studies of plant growth and development. Front. Plant Sci. 10 , 1577 (2019).
Millar, K. D. L. et al. A novel phototropic response to red light is revealed in microgravity. N. Phytol. 186 , 648–656 (2010).
Vandenbrink, J. P., Herranz, R., Medina, F. J., Edelmann, R. E. & Kiss, J. Z. A novel blue-light phototropic response is revealed in roots of Arabidopsis thaliana in microgravity. Planta 244 , 1201–1215 (2016).
Izzo, L. G. et al. Interaction of gravitropism and phototropism in roots of Brassica oleracea . Environ. Exp. Bot. 193 , 104700 (2022).
Kiss, J. Z., Millar, K. D. L. & Edelmann, R. E. Phototropism of Arabidopsis thaliana in microgravity and fractional gravity on the International Space Station. Planta 236 , 635–645 (2012).
Boccalandro, H. E. et al. PHYTOCHROME KINASE SUBSTRATE1 regulates root phototropism and gravitropism. Plant Physiol. 146 , 108–115 (2008).
Reichler, S. A. et al. Light differentially regulates cell division and the MRNA abundance of pea nucleolin during de-etiolation. Plant Physiol. 125 , 339–350 (2001).
Valbuena, M. A. et al. The combined effects of real or simulated microgravity and red-light photoactivation on plant root meristematic cells. Planta 248 , 691–704 (2018).
Medina, F. J., Manzano, A., Villacampa, A., Ciska, M. & Herranz, R. Understanding reduced gravity effects on early plant development before attempting life-support farming in the Moon and Mars. Front. Astron. Space Sci. 8 , 729154 (2021).
Kordyum, E. & Hasenstein, K. H. Plant biology for space exploration – Building on the past, preparing for the future. Life Sci. Space Res. 29 , 1–7 (2021).
Musgrave, M. E. & Kuang, A. Plant reproductive development during spaceflight. Adv. Space Biol. Med. 9 , 1–23 (2003).
Merkys, A. L. & Laurinavicius, R. S. Complete cycle of individual development of Arabidopsis thaliana (L.) Heynh. plants on board the Salyut-7 orbital station. Dokl. Akademii Nauk SSSR 271 , 509–512 (1983).
Google Scholar
Link, B. M., Busse, J. S. & Stankovic, B. Seed-to-seed-to-seed growth and development of Arabidopsis in microgravity. Astrobiology 14 , 866–875 (2014).
Kuang, A., Popova, A., Xiao, Y. & Musgrave, M. E. Pollination and embryo development in Brassica rapa L. in microgravity. Int. J. Plant Sci. 161 , 203–211 (2000).
Kuang, A., Xiao, Y., McClure, G. & Musgrave, M. E. Influence of microgravity on ultrastructure and storage reserves in seeds of Brassica rapa L. Ann. Bot. 85 , 851–859 (2000).
De Micco, V., De Pascale, S., Paradiso, R. & Aronne, G. Microgravity effects on different stages of higher plant life cycle and completion of the seed-to-seed cycle. Plant Biol. 16 , 31–38 (2014).
Le Caër, S. Water radiolysis: Influence of oxide surfaces on H2 production under ionizing radiation. Water 3 , 235–253 (2011).
Caplin, N. & Willey, N. Ionizing radiation, higher plants, and radioprotection: from acute high doses to chronic low doses. Front. Plant Sci. 9 , 847 (2018).
Hasanuzzaman, M., Hossain, M. A., da Silva, J. A. T. & Fujita, M. Plant response and tolerance to abiotic oxidative stress: Antioxidant defense is a key factor in Crop Stress and its Management: Perspectives and Strategies (eds. Venkateswarlu, B. et al.) 261-315 (Springer, 2012).
Gudkov, S. V., Grinberg, M. A., Sukhov, V. & Vodeneev, V. Effect of ionizing radiation on physiological and molecular processes in plants. J. Environ. Radioact . 202 , 8-24 (2019).
Agathokleous, E., Feng, Z. Z. & Peñuelas, J. Chlorophyll hormesis: are chlorophylls major components of stress biology in higher plants? Sci. Total Environ. 726 , 138637 (2020).
De Micco, V., Arena, C., Pignalosa, D. & Durante, M. Effects of sparsely and densely ionizing radiation on plants. Radiat. Environ. Biophys. 50 , 1–19 (2011).
De Micco, V., Arena, C. & Aronne, G. Anatomical alterations of Phaseolus vulgaris L. mature leaves irradiated with X-rays. Plant Biol. 16 , 187–193 (2014).
De Micco, V. et al. Leaf anatomy and photochemical behaviour of Solanum lycopersicum L. Plants from seeds irradiated with low-let ionising radiation. Sci. World J. 2014 , 428141 (2014).
Fulcher, N. & Sablowski, R. Hypersensitivity to DNA damage in plant stem cell niches. Proc. Natl Acad. Sci. 106 , 20984–20988 (2009).
Arena, C. et al. Anatomy, photochemical activity, and DNA polymorphism in leaves of dwarf tomato irradiated with X-rays. Biol. Plant. 61 , 305–314 (2017).
Shikazono, N., Tanaka, A., Kitayama, S., Watanabe, H. & Tano, S. LET dependence of lethality in Arabidopsis thaliana irradiated by heavy ions. Radiat. Environ. Biophys. 41 , 159–162 (2002).
Arena, C., De Micco, V., Macaeva, E. & Quintens, R. Space radiation effects on plant and mammalian cells. Acta Astronaut. 104 , 419–431 (2014).
Luckey, T. D. (ed.). Hormesis with Ionizing Radiation . CRC Press, Inc. (1980).
Van Hoeck, A. et al. Lemna minor plants chronically exposed to ionising radiation: RNA-seq analysis indicates a dose rate dependent shift from acclimation to survival strategies. Plant Sci. 257 , 84–95 (2017).
Mulinacci, N. et al. Effects of ionizing radiation on bio-active plant extracts useful for preventing oxidative damages. Nat. Prod. Res. 33 , 1106–1114 (2019).
Rezk, A. A., Al-Khayri, J. M., Al-Bahrany, A. M., El-Beltagi, H. S. & Mohamed, H. I. X-ray irradiation changes germination and biochemical analysis of two genotypes of okra ( Hibiscus esculentus L.). J. Radiat. Res. Appl. Sci. 12 , 393–402 (2019).
Volkova, P. Y., Bondarenko, E. V. & Kazakova, E. A. Radiation hormesis in plants. Curr. Opin. Toxicol. 30 , 100334 (2022).
Shi, J. M. et al. Cytogenetic effects of low doses of energetic carbon ions on rice after exposures of dry seeds, wet seeds and seedlings. J. Radiat. Res. 51 , 235–242 (2010).
Riviello-Flores, M. et al. Use of gamma radiation for the genetic improvement of underutilized plant varieties. Plants 11 , 1161 (2022).
De Micco, V. et al. Effect of light quality and ionising radiation on morphological and nutraceutical traits of sprouts for astronauts’ diet. Acta Astronaut. 185 , 188–197 (2021).
Shuryak, I. Review of resistance to chronic ionizing radiation exposure under environmental conditions in multicellular organisms. J. Environ. Radioact. 212 , 106128 (2020).
Cucinotta, F. A., Kim, M. H. Y., Willingham, V. & George, K. A. Physical and biological organ dosimetry analysis for international space station astronauts. J. Radiat. Res. 170 , 127–138 (2008).
Takahashi, A., Ikeda, H. & Yoshida, Y. Role of high-linear energy transfer radiobiology in space radiation exposure risks. Int. J. Part. Ther. 5 , 151–159 (2018).
Zhang, S. et al. First measurements of the radiation dose on the lunar surface. Sci. Adv. 6 , eaaz1334 (2020).
Monje, O. et al. Hardware validation of the Advanced Plant Habitat on ISS: canopy photosynthesis in reduced gravity. Front. Plant Sci. 11 , 673 (2020).
Massa, G. D., Newsham, G., Hummerick, M. E., Morrow, R. C. & Wheeler, R. M. Plant pillow preparation for the Veggie Plant Growth System on the International Space Station. Gravit. Space Res. 5 , 24–34 (2017).
Massa, G. D., Wheeler, R. M., Morrow, R. C. & Levine, H. G. Growth chambers on the International Space Station for large plants. Acta Hortic. 1134 , 215–222 (2016).
Thiel, C. S. et al. Real-time 3D high-resolution microscopy of human cells on the International Space Station. Int. J. Mol. Sci. 20 , 2033 (2019).
Download references
Acknowledgements
This work was supported by the European Space Agency (ESA). This perspective paper is based upon work from the Contributors to the Topic C “Plant Biology” of the ESA SciSpacE white paper “Biology in Space and Analogue Environments”, listed in alphabetic order: Giovanna Aronne, Nicol Caplin, Eugénie Carnero-Diaz, Raúl Herranz, Nele Horemans, Ann-Iren Kittang Jost (Coordinator), Valerie Legue, F. Javier Medina, Veronica De Micco, (Coordinator), Veronica Pereda-Campos, Mona Schiefloe.
Author information
Authors and affiliations.
Department of Agricultural Sciences, University of Naples Federico II, via Università 100, 80055, Portici (NA), Italy
Veronica De Micco, Giovanna Aronne, Sara De Francesco & Luigi Gennaro Izzo
SciSpacE Team, Directorate of Human and Robotic Exploration Programmes, European Space Agency (ESA), Noordwijk, Netherlands
Nicol Caplin
Institute of Systematic, Evolution, Biodiversity, Sorbonne University, National Museum of Natural History, CNRS, EPHE, UA, 45, rue Buffon CP50, 75005, Paris, France
Eugénie Carnero-Diaz & Isabel Le Disquet
Centro de Investigaciones Biológicas Margarita Salas - CSIC, Ramiro de Maeztu 9, 28040, Madrid, Spain
Raúl Herranz & F. Javier Medina
Belgian Nuclear Research Centre (SCK CEN), Biosphere Impact Studies (BIS), Boeretang 200, 2400, Mol, Belgium
Nele Horemans
Université Clermont Auvergne, INRAE, PIAF, F-63000, Clermont-Ferrand, France
Valérie Legué
GSBMS/ Evolsan UFR Santé, University of Toulouse III, Toulouse, France
Veronica Pereda-Loth
NTNU Social Research, Centre for Interdisciplinary Research in Space (CIRiS) Dragvoll Allé 38 B, 7049, Trondheim, Norway
Mona Schiefloe & Ann- Iren Kittang Jost
You can also search for this author in PubMed Google Scholar
Contributions
V.D., G.A., N.C., E.C., R.H., N.H., V.L., J.M., V.P., M.S. and A.K. developed the concept of this perspective paper. V.D., G.A., S.D. and L.I. developed a first structure of the manuscript. V.D. took lead in coordination and writing; G.A., N.C., E.C., S.D., R.H., N.H., L.I., I.L.D., V.L., J.M., V.P., M.S. and A.K. wrote specific parts of the manuscript. All authors provided critical feedback and helped shape the concept and perspectives. All authors revised and approved the submitted version of the manuscript.
Corresponding author
Correspondence to Veronica De Micco .
Ethics declarations
Competing interests.
The authors declare no competing interests.
Additional information
Publisher’s note Springer Nature remains neutral with regard to jurisdictional claims in published maps and institutional affiliations.
Rights and permissions
Open Access This article is licensed under a Creative Commons Attribution 4.0 International License, which permits use, sharing, adaptation, distribution and reproduction in any medium or format, as long as you give appropriate credit to the original author(s) and the source, provide a link to the Creative Commons license, and indicate if changes were made. The images or other third party material in this article are included in the article’s Creative Commons license, unless indicated otherwise in a credit line to the material. If material is not included in the article’s Creative Commons license and your intended use is not permitted by statutory regulation or exceeds the permitted use, you will need to obtain permission directly from the copyright holder. To view a copy of this license, visit http://creativecommons.org/licenses/by/4.0/ .
Reprints and permissions
About this article
Cite this article.
De Micco, V., Aronne, G., Caplin, N. et al. Perspectives for plant biology in space and analogue environments. npj Microgravity 9 , 67 (2023). https://doi.org/10.1038/s41526-023-00315-x
Download citation
Received : 10 November 2022
Accepted : 02 August 2023
Published : 21 August 2023
DOI : https://doi.org/10.1038/s41526-023-00315-x
Share this article
Anyone you share the following link with will be able to read this content:
Sorry, a shareable link is not currently available for this article.
Provided by the Springer Nature SharedIt content-sharing initiative
Quick links
- Explore articles by subject
- Guide to authors
- Editorial policies
Sign up for the Nature Briefing newsletter — what matters in science, free to your inbox daily.

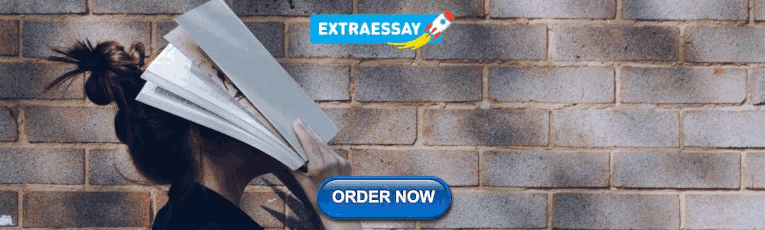
IMAGES
VIDEO
COMMENTS
Call for Papers - Special Issues Impacts of Droughts and Drought Legacies on Plants and Ecosystems Edited by: Michael Bahn, Thorsten E.E. Grams, Charlotte Grossiord, Lucy Rowland, Christiane Werner ... Research and Application . January 2014 Special Issue: Plant Biology in Space . ... Plant Biology preceding journal backfiles now available!
Overview. Journal of Plant Research is an international publication focusing on fundamental knowledge in all areas of plant sciences. Encourages work based on unique approaches and unprecedented findings. Welcomes interdisciplinary studies and newly developing areas of basic plant biology. Papers should be hypothesis-driven, not purely descriptive.
Haploids fast-track hybrid plant breeding. Two studies report the use of paternal haploids to enable one-step transfer of cytoplasmic male sterility in maize and broccoli, which resolves a key ...
Journal of Plant Biology is an international journal dedicated to essential research in various fields of plant science. Recognized for detailing significant research contributions to plant science. Broad coverage, including biotechnology, biochemistry, and macromolecular structure. Focuses on cellular and developmental biology, ecology ...
Nature Plants is a scientific journal publishing primary research papers concerned with all aspects of plant biology, technology, ecology and evolution.
Unearth groundbreaking research in BMC Plant Biology, an open access journal with open collections, 5.3 Impact Factor and 17 days to first decision. ... BMC Plant Biology is an open access, peer-reviewed journal that considers articles on all aspects of plant biology, ... 1.415 - SNIP (Source Normalized Impact per Paper) 1.159 - SJR (SCImago ...
Unearth groundbreaking research in BMC Plant Biology, an open access journal with open collections, 5.3 Impact Factor and 17 days to first decision. ... Citation: BMC Plant Biology 2024 24:169 Content type: Research Published on: 5 March 2024. View Full Text ... 1.415 - SNIP (Source Normalized Impact per Paper) 1.159 - SJR (SCImago Journal Rank ...
Plant biology is a key area of science that bears major weight in the mankind's ongoing and future efforts to combat the consequences of global warming, climate change, pollution, and population growth. An in-depth understanding of plant physiology is paramount to our ability to optimize current agricultural practices, to develop new crop ...
The resulting publications will be open access. This journal aims to acknowledge and encourage interdisciplinary research in fundamental plant sciences with scope to address crop improvement, biodiversity, nutrition and human health. It publishes review articles, original research papers, method papers and short articles in plant research ….
ISSN: 1543-5008. eISSN: 1545-2123. AIMS AND SCOPE OF JOURNAL: The Annual Review of Plant Biology covers the significant developments in the field of plant biology, including biochemistry and biosynthesis, genetics, genomics and molecular biology, cell differentiation, tissue, organ and whole plant events, acclimation and adaptation, and methods ...
Explore our most highly accessed plant science articles in 2017. Featuring authors from around the World, these papers highlight valuable research within plant science from an international community.
Involvement of GLR-mediated nitric oxide effects on ROS metabolism in Arabidopsis plants under salt stress. Azime Gokce. Askim Hediye Sekmen Cetinel. Ismail Turkan. Regular Paper - Physiology/Biochemistry/Molecular and Cellular Biology Open access 06 March 2024.
Non-Destructive Quality Assessment and Intelligent Packaging of Agricultural Products. Jiangbo Li. Yuzhen Lu. Hailiang Zhang. Jun Wang. 300 views. The most cited plant science journal advances our understanding of plant biology for sustainable food security, functional ecosystems and human health.
International Journal of Plant Biology is an international, peer-reviewed, open access journal on all different subdisciplines of plant biology, published quarterly online by MDPI (from Volume 13, Issue 1 - 2022).. Open Access — free for readers, with article processing charges (APC) paid by authors or their institutions.; High Visibility: indexed within Scopus, Biological Abstracts and ...
This paper provides a comprehensive review of the current state of plant biodiversity, the main threats it faces, and the possible solutions for its conservation. It covers the definition ...
Plant biotechnology is a mature technology. Modern biotechnology was a scientifically obvious outcome of the striking advances in molecular biology that followed the discovery of the bacterial DNA restriction-modification system (Luria and Human, 1952; Luria, 1953; Dussoix and Arber, 1962; Nathans and Smith, 1975).Microorganisms and plants were the first organisms to be manipulated to serve ...
In this Focus Issue, we go back to the "roots" of the journal, that gave it its name, and turn our attention to the many fascinating facets of plant cell biology. The 11 review papers and 11 original research articles in this issue exemplify the breadth of the field and highlight both established concepts and recent achievements.
Abstract. The advance in genomics technology leads to the dramatic change in plant biology research. Plant biologists now easily access to enormous genomic data to deeply study plant high-density genetic variation at molecular level. Therefore, fully understanding and well manipulating bioinformatics tools to manage and analyze these data are ...
Recent papers in Plant Biology. Top Papers; Most Cited Papers; Most Downloaded Papers; Newest Papers; ... Key research areas in plant science include plant species identification, weed classification using hyper spectral images, monitoring plant health and tracing leaf growth, and the semantic interpretation of leaf information. ...
The Plant Biology Section is home to some of the most influential plant science research in the world. The Section represents diverse intellectual interests and boasts a collaborative community culture. Including emeritus professors, there are three members of the National Academy of Sciences. Undergraduates, graduate students, and post-doctoral researchers can join field-leading faculty as ...
Haploids fast-track hybrid plant breeding. Two studies report the use of paternal haploids to enable one-step transfer of cytoplasmic male sterility in maize and broccoli, which resolves a key ...
Sergii Litvinov is a research associate in Kyiv, Ukraine. He is a plant and molecular biologist currently studying the effects of stress factors on the production of prion-like proteins in plants. His research is also related to radiation-induced genomic instability, DNA repair and transcriptional response to DNA damage.
Purdue University. Summary: A research team has begun translating the complex molecular language of petunias. Their grammar and vocabulary are well hidden, however, within the countless proteins ...
The findings of his research with Dr. Dorothy Shippen offer insight into the effects of spaceflight on plant chromosomes and how these findings could impact human health. Postdoctoral research associate at Texas A&M University Borja Barbero explains the importance of studying plant telomeres in space. ... Plant Biology. Microbiology. Cell and ...
A Feature Paper should be a substantial original Article that involves several techniques or approaches, provides an outlook for future research directions and describes possible research applications. Feature papers are submitted upon individual invitation or recommendation by the scientific editors and must receive positive feedback from the ...
Plant cell biology is the study of all aspects of plant cells. It is particularly concerned with structure, growth, division, signalling, differentiation and death of plant cells. Cell edges are ...
March 27, 2024. Source: School of Science, The University of Tokyo. Summary: Researchers have discovered the first species pollinated by sap beetles in the genus Pandanus, a group of palm-like ...
Sorghum, or broomcorn, is a staple crop in sub-Saharan Africa, but approximately 20% of annual yields are lost due to infections with witchweed (Striga hermonthica), a parasitic plant that steals nutrients and water by latching onto the plant's roots.In a new study in Cell Reports published on March 26, University of California, Davis researchers show that soil microbes can suppress ...
This perspective paper is based upon work from the Contributors to the Topic C "Plant Biology" of the ESA SciSpacE white paper "Biology in Space and Analogue Environments", listed in ...