Featured Topics
Featured series.
A series of random questions answered by Harvard experts.
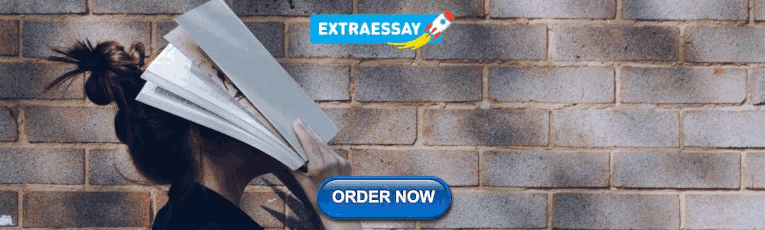
Explore the Gazette
Read the latest.
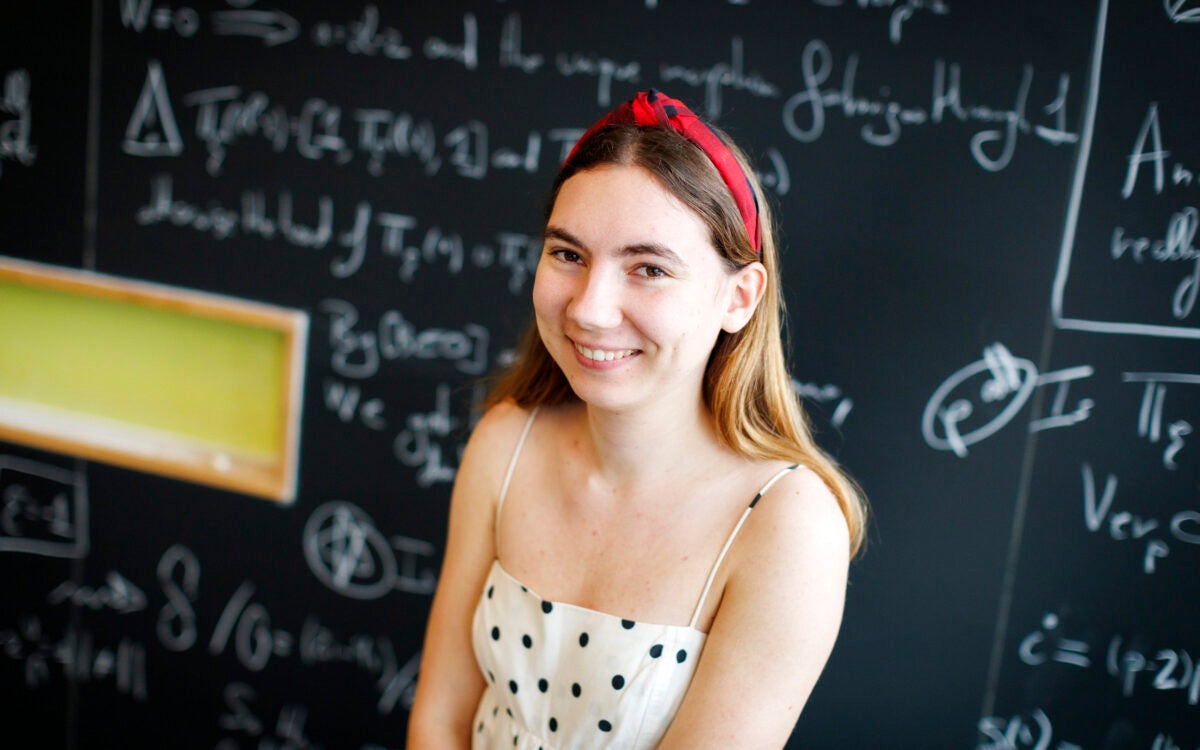
When math is the dream
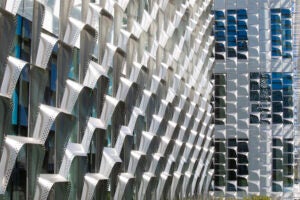
A space for researchers to meet, and AI and natural intelligence to do the same

A fresh take on must-see TV
Daniel Wegner redefined social psychology as the science of human experience. He was arguably most famous for his experiments on thought suppression, in which people were unable to keep from thinking of a white bear.
File photo by Jon Chase/Harvard Staff Photographer
Daniel M. Wegner famous for ‘thought suppression’
Peter Reuell
Harvard Staff Writer
Pioneer in social psychology, explored human experience with wit, ingenuity
Daniel M. Wegner, a pioneering social psychologist who helped to reveal the mysteries of human experience through his work on thought suppression, conscious will, and mind perception, died July 5 as a result of amyotrophic lateral sclerosis (ALS). He was 65.
The John Lindsley Professor of Psychology in Memory of William James, Wegner redefined social psychology as the science of human experience. He was arguably most famous for his experiments on thought suppression, in which people were unable to keep from thinking of a white bear.
Wegner also broke ground in other areas of social psychology, including transactive memory (how memories are distributed across groups and relationship partners) and action identification (what people think they are doing). He had also explored the experience of conscious will, and most recently focused on mind perception (how people perceive human and nonhuman minds).
“Dan was, I believe, the most original thinker in modern psychology,” said Dan Gilbert, the Edgar Pierce Professor of Psychology, who knew Wegner for three decades. “Most of us work on problems that are important in our field, and we use theories others have invented to make progress. Dan didn’t make progress — Dan made new highways, new roads. He opened doors in walls that we didn’t know had doors in them, and he did this over and over.”
Gilbert said he was privileged to call Wegner one of his closest friends. The two met while they both worked in Texas — Gilbert at the University of Texas and Wegner at Trinity University.
“Being among the few social psychologists in Texas, we were introduced by a mutual friend, and it was love at first sight,” Gilbert said. “We’ve been true friends ever since.” He added. “I’m heartbroken to lose my friend of 30 years, but I guess the only thing worse would have been not to have a friend of 30 years.”
While Wegner was known for his pioneering work on the mind, Gilbert said his intellectual curiosity seemed never to rest.
“The thing about Dan is he didn’t take the lab coat off,” Gilbert said. “For him, being a psychologist wasn’t a job, it was a way of being. He simply spent all his waking time thinking about the interesting aspects of the mind. It was 24/7 for him.”
That intellectual heft, however, never masked Wegner’s humor.
“Dan Wegner was the funniest human being I’ve ever known, and everybody else was a distant second,” Gilbert said. “To say someone was funny may sound frivolous, but I would make the claim that Dan understood something important, which is that humor is the place where intelligence and joy meet. Dan understood that … humor is where a brilliant mind tickles itself.”
That sense of humor, Gilbert said, often showed up in Wegner’s writing, and helped transform the way social psychology is described in many journals today. “If you open a psychology journal now,” he said, “many, many people write in a Wegner-esque style.”
Even in his final days, Gilbert said, Wegner’s restless mind faced the challenge of his death with an inspirational degree of curiosity.
“It was a privilege to sit by his side as he took this journey to the end,” Gilbert said. “About a month ago, I asked him, ‘If you had to think of one word to describe this experience, what would it be?’ He looked at me, and he said ‘fascinating.’ He was a student of the human experience, and he was having an experience unlike most of us ever have. And rather than bemoaning it or crying about it, he took it as another fascinating thing to study and learn about and think about.”
Born in Calgary, Alberta, Canada, Wegner studied as an undergraduate and graduate student at Michigan State University, earning his Ph.D. in 1974. He was appointed an assistant professor and rose to full professor and chair of the psychology department at Trinity in San Antonio.
Wegner joined the faculty in the psychology department at the University of Virginia in 1990, where he was the William R. Kenan Jr. Professor of Psychology before joining the Harvard faculty in 2000.
Wegner was the author of four academic books, an introductory psychology textbook, and nearly 150 journal articles and book chapters.
Wegner’s research was funded by the National Science Foundation and the National Institute of Mental Health. In 1996-1997 he was a fellow at the Center for Advanced Study in the Behavioral Sciences, and in 2011 was inducted as a fellow of the American Academy of Arts and Sciences. He received many of the top honors in his field, including the William James Fellow Award from the Association for Psychological Science, the Distinguished Scientific Contribution Award from the American Psychological Association, the Distinguished Scientist Award from the Society of Experimental Social Psychology, and the Donald T. Campbell Award from the Society for Personality and Social Psychology.
Wegner is survived by his wife of 29 years, Toni Giuliano Wegner of Winchester, and his daughters, Kelsey Wegner Hurlburt of Dunkirk, Md., and Haley Wegner of Winchester. At Wegner’s request, his body was donated to the Massachusetts General Hospital’s Neurological Clinical Research Institute for ALS Research.
A memorial service will be held at 4 p.m. on Saturday at the Winchester Unitarian Society, 478 Main St., Winchester, Mass. Wegner requested that his service be a celebration of life, and so would welcome Hawaiian shirts.
In lieu of flowers, donations can be made to:
Compassionate Care ALS P.O. Box 1052 West Falmouth, Mass. 02574
Winchester Unitarian Society 478 Main Street Winchester, Mass. 01890
Share this article
You might like.
Dora Woodruff was drawn to beauty of numbers as child. Next up: Ph.D. at MIT.

The Kempner Institute buzzes with new code, numbers, ideas
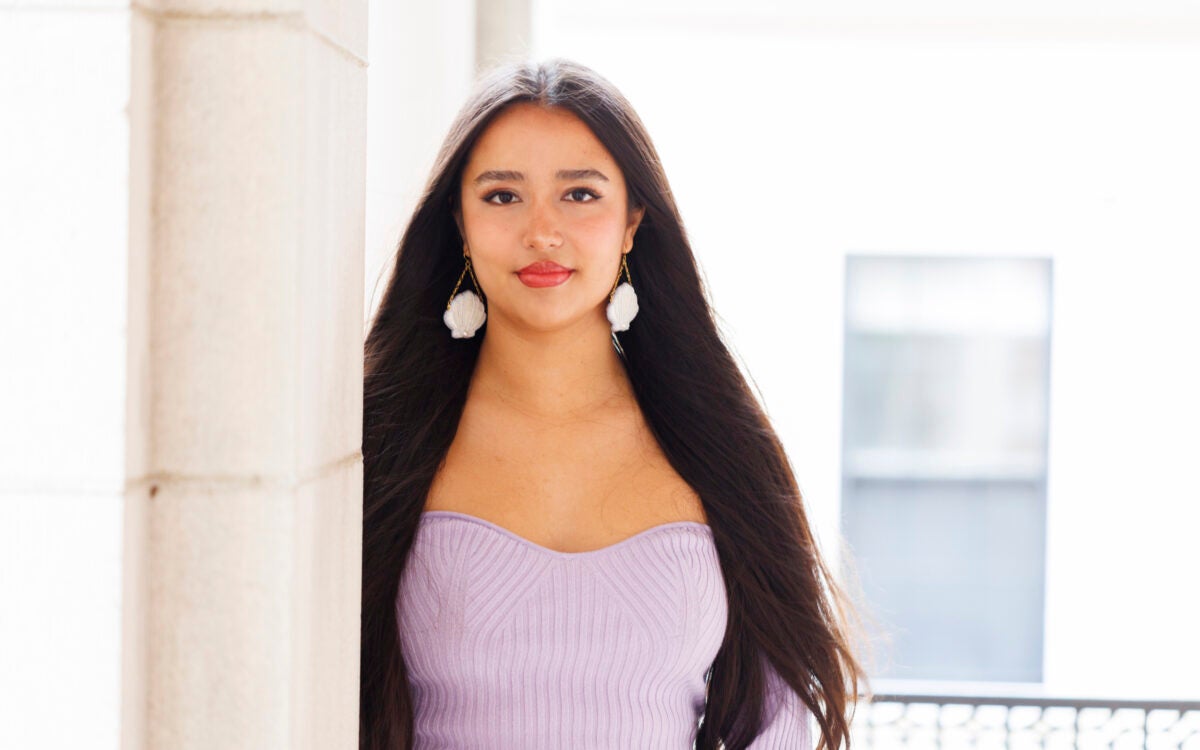
Isabella Madrigal has a vision: storytelling that’s compelling, inspires better, more equitable world
Exercise cuts heart disease risk in part by lowering stress, study finds
Benefits nearly double for people with depression
So what exactly makes Taylor Swift so great?
Experts weigh in on pop superstar's cultural and financial impact as her tours and albums continue to break records.
Good genes are nice, but joy is better
Harvard study, almost 80 years old, has proved that embracing community helps us live longer, and be happier
Age-related differences in the attentional white bear
- Open access
- Published: 10 June 2019
- Volume 26 , pages 1870–1888, ( 2019 )
Cite this article
You have full access to this open access article
- Brandon K. Ashinoff 1 , 2 , 3 ,
- Yehoshua Tsal 4 , 5 &
- Carmel Mevorach 1 , 2
2078 Accesses
2 Citations
3 Altmetric
Explore all metrics
A Correction to this article was published on 01 November 2019
This article has been updated
The cognitive aging literature suggests that aging populations exhibit impairments in the proactive inhibition of attention. Although proactive inhibition is often preceded by the allocation of attention toward the predicted or known spatial location of to-be-ignored stimuli, proactive allocation of attention has not been assessed in aging populations. In this study, an older and younger cohort engaged in the attentional-white-bear paradigm which measures proactive allocation of attention. In this task, on 80% of trials, participants must identify a centrally located letter surrounded by congruent or incongruent flanker letters. The flanker locations are fixed and predictable within each block of the study. On 20% of trials, they must identify which of two dots appear first on the screen. One dot appears in the same location as the flanker, and one appears in an empty location during the flanker task. The typical white-bear effect is that, despite the dots appearing at the same time, participants more often report the dot in the location of the flanker (i.e., the potentially to-be-ignored location) to appear first. The magnitude of this effect is interpreted as the magnitude of attentional allocation prior to inhibition. In Experiment 1, there was no difference in the magnitude of the attentional white bear between younger and aging cohorts. However, when the attentional system was sufficiently taxed by reducing the flanker presentation (Experiments 2a and 2b), age-related differences emerged. In particular, older participants showed a reduced white-bear effect, reflecting a potential impairment in the proactive allocation of attention toward the location of expected distractors.
Similar content being viewed by others
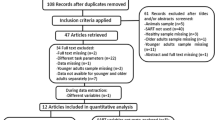
Age differences in sustained attention tasks: A meta-analysis
Antonino Vallesi, Virginia Tronelli, … Rachele Pezzetta
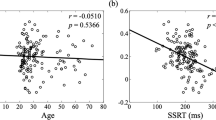
Imaging the effects of age on proactive control in healthy adults
Sien Hu, Manna Job, … Chiang-shan R. Li
Inattentional blindness in older adults: Effects of attentional set and to-be-ignored distractors
Sally Horwood & Vanessa Beanland
Avoid common mistakes on your manuscript.
As we age, many changes take place that alter our cognitive abilities (Craik & Salthouse, 2011 ; Grady, 2012 ; Hedden & Gabrieli, 2004 ; Persson et al., 2006 ). The dual-mechanisms theory of proactive and reactive control (DMC; Braver, 2012 ) argues that cognitive control is driven by two primary mechanisms. Proactive control is an “early selection” mechanism that allows one to select and maintain goal-relevant information prior to stimulus presentation in order to prepare a response to a given stimulus based on prior knowledge. Reactive control is a “late correction” mechanism that allows one to alter behavioral plans in the moment when suddenly presented with new and relevant information. Recent research has shown that older participants tend to favor reactive over proactive control strategies (Jimura & Braver, 2010 ; Paxton, Barch, Racine, & Braver, 2008 ), due to a presumed age-related impairment to proactive control mechanisms.
These studies generally report age-related impairments related to the proactive inhibition of attention. This is important because several researchers have argued that a prominent aspect of cognitive aging is a decline in the ability to proactively ignore (i.e., inhibit) distracting information (the inhibition-deficit theory of cognitive aging; Braver, 2012 ; Hasher, Stoltzfus, Zacks, & Rypma, 1991 ; Hasher & Zacks, 1988 ; Kramer, Humphrey, Larish, & Logan, 1994 ; Lustig & Jantz, 2015 ). However, some accounts of inhibition processes suggest that an initial allocation of attention toward distractors is necessary for their (ostensibly proactive) inhibition. This may have important implications for inhibition-deficit theories of cognitive aging (e.g., Lustig, Hasher, & Zacks, 2007 ) because impairments in attentional allocation prior to inhibition could result in cascading effects that would appear as inhibition deficits. Essentially, it may be more difficult to inhibit something if you are bad at identifying and locating it in the first place. To date, few studies have attempted to assess proactive allocation of attention in aging populations. This study attempts to fill in this gap in the literature by investigating whether proactive allocation of attention prior to inhibition is impaired in aging populations.
One method that has been used to measure proactive allocation prior to inhibition is the preview search paradigm (Watson & Humphreys, 1997 ), in which participants have to search for a predefined target among distractors, but a subset of the distractors is presented (the preview display) at least 400 ms prior to the target display. Typically, it is found that participants are able to inhibit the preview array, so that search is restricted to the new items presented later (as if the new items are presented on their own). Interestingly, using dot probes (Allen, Humphreys, & Matthews, 2008 ; Humphreys, Stalmann, & Olivers, 2004 ; Olivers, Humphreys, & Braithwaite, 2006 ) and electrophysiological measures (Belopolsky, Peterson, & Kramer, 2005 ), it has been demonstrated that the relevant to-be-ignored locations are initially proactively attended to and identified prior to adopting an inhibitory bias against the locations of the previewed distractors—a process called visual marking. Using the preview search paradigm with older participants, Watson and Maylor ( 2002 ; Kramer & Atchley, 2000 ; Kramer & Kray, 2006 ) showed that older participants produced standard visual marking effects, as long as static displays were used. While this points to a possible difference in how older participants search static versus dynamic displays (also see Becic, Kramer, & Boot, 2007 ), it is unclear if this is related to the initial allocation of attention to the preview items or to differences in inhibition processes. Therefore, while this may suggest that proactively allocating attention toward to-be ignored distractors is intact in old age, it is by no means conclusive.
Other paradigms have also been used to assess proactive allocation of attention prior to inhibition in young, neurotypical cohorts. For example, Moher and Egeth ( 2012 ; Cepeda, Cave, Bichot, & Kim, 1998 ; Cunningham & Egeth, 2016 ; Jollie, Ivanoff, Webb, & Jamieson, 2016 ; Munneke, Van der Stigchel, & Theeuwes, 2008 ) showed that cueing nontarget (i.e., distractor) features (i.e., IGNORE RED) resulted in the allocation of attention toward a to-be-ignored item prior to inhibition in a visual search task (also measured using detection of a probe dot). In another example, Max and Tsal ( 2015 ) characterized the temporal dynamics of proactive allocation and inhibition. Participants were presented with a flanker task that began with identical target and distractor items. However, at a random interval during the trial, the distractor items would mutate into incongruent or neutral distractors. They found that performance was impaired if the distractor mutation occurred within the first 50 ms of stimulus presentation, suggesting that at least some attention was allocated toward them early on. If the mutation occurred after 50 ms, the new identities of the flanker items were successfully inhibited and had no effect on task performance. However, Max and Tsal ( 2015 ) noted that in their paradigm the process they characterized might be preattentive or reflective of an attentional “zoom lens” contracting around the target, rather than a shift from allocation to inhibition. Crucially, none of these paradigms were tested with an older cohort, and it is therefore still not clear whether age-related differences in proactive attentional allocation occur.
A more direct measure of proactive allocation of attention to distractors is provided in the attentional-white-bear (AWB) paradigm (Tsal & Makovski, 2006 ). In the AWB paradigm, participants engage in a primary flanker task—identifying a central letter flanked by two diagonal distractors (e.g., top left and bottom right) appearing in the same locations throughout a block of trials. However, on a small minority of trials (20%), a temporal-order-judgment task appears instead of the flankers’ display, in which participants indicate which of two simultaneously presented dots appeared first. The purpose of the flanker task is to induce an expectation that participants will have to ignore a flanker at a specific location and time (the flanker location was blocked, and the inter-stimulus interval (ISI) was consistent throughout the study). The flanker appears in the same location as one of the two dots, so the participants are preparing to inhibit a flanker, but get a dot instead on some trials. The critical finding (Tsal & Makovski, 2006 ) is a tendency to identify the dot that appeared at an expected distractor position as appearing before the dot occupying the expected empty location—referred to as the attentional-white-bear effect. In fact, the effect holds even when significant perceptual, memory, and sensory constraints are placed on the flanker task (Lahav, Makovski, & Tsal, 2012 ). This bias toward the expected (to-be-ignored) flanker position is attributed to attention allocation to the expected flanker position prior to the flanker presentation and therefore represents a direct measure of proactive allocation of attention to distractors prior to expected inhibition. Thus, the magnitude of the AWB effect is a metric representing the proactive allocation of attention.
It should be noted that we are not claiming that all of these paradigms are necessarily reflective of the exact same process. However, each of these paradigms arguably engage the proactive allocation of attention ostensibly as a precursor to the proactive inhibition of attention. Crucially, these processes do not necessarily engage proactive allocation and inhibition in the exact same manner, leading to distinct inhibition processes based on similar mechanisms. In other words, even if the attentional-white-bear, visual marking, and other studies do not reflect the exact same process, they do engage the same mechanism (proactive allocation of attention) as part of those processes, and our test of this mechanism has implication for all of the processes in which it is engaged.
To directly assess proactive allocation of attention to distractors in aging populations, we employed the fixed-block attentional-white-bear paradigm (Tsal & Makovski, 2006 ) in two groups of younger and older healthy adults. Specifically, we ask whether the magnitude of the white-bear effect (proportion of responses identifying the dot appearing in the expected distractor location as appearing first in time, relative to those appearing at the expected empty location), which arguably reflects the magnitude of the resources proactively allocated to the expected distractor location, differs between younger and older participants. In addition, we also manipulated the contrast of the flankers to include both equal-contrast (compared with the target) and low-contrast flankers. Low-contrast flankers may require more attention to be allocated to them within an allocate-first scenario, and this enables us to test whether differences in the AWB effect between the age groups emerge when flankers are harder to process. Thus, two versions of the task were developed. In one, the flanker distractors are equal in contrast to the target letters. In the second version, the flanker distractors are of significantly lower contrast than the target letters.
Experiment 1: Methods
Power analysis.
An a priori statistical power analysis for a repeated-measures ANOVA was performed for sample size estimation, based on data from Lahav et al. ( 2012 , Experiment 1). In this study, they found a significant white-bear effect, defined as a main effect of distractor location in a temporal-order-judgment task—similar to the one used in our study. Although not reported in the original paper, through personal communication with the authors we obtained the partial eta squared ( \({\upeta}_{\mathrm{p}}^2\) = .242) of the white-bear effect in this study, which converted to an effect size f (U) of 0.565. (The other parameters of the analysis were set as the following: alpha = .05, power = 0.95, number of groups = 2, number of measurements = 2, nonsphericity correction = 1.) Based on this, the total projected sample size (across all groups) needed to detect a white-bear effect with an effect size of .565 (G*Power, Version 3.1.9.2; Faul, Erdfelder, Buchner, & Lang, 2009 ; Faul, Erdfelder, Lang, & Buchner, 2007 ; Lakens, 2013 , Appendix A ) is 46 for a repeated-measures ANOVA with a within–between subjects interaction. Thus, an ideal study would include 23 participants per group to have a power of .956 (see Appendix A ). In Experiment 1, there are 21 younger and 22 older participants ( N = 43; a priori power = .942; see Appendix A ). In Experiment 2a, there are 20 younger and 20 older participants ( N = 40; a priori power = .924; see Appendix A ). In Experiment 2b, there are 20 younger and 19 older participants ( N = 39; a priori power = .917; see Appendix A ).
Participants
Twenty-five younger and 26 older participants took part in this study; however, because of technical issues during a couple of experiment sessions, we had to exclude two younger and two older participants. We further excluded two younger and two older participants because of poor performance (>20% incorrect button presses during the temporal-order-judgment task). Twenty-one younger ( M age = 18.71 years, SEM age = 0.18, age range: 18–21 years, 20 females) and 22 older ( M age = 70.77 years, SEM age = 1.37, age range: 60–82 years, 13 females) participants were included in the final analysis. The two groups participated in two successive behavioral experiments. The order of the tasks was counterbalanced to account for possible fatigue and order effects. Younger participants were recruited from the undergraduate population in the school of psychology at the University of Birmingham, UK. They were compensated for their participation with course credits. The older participants were recruited from a volunteer pool maintained by the School of Psychology at the University of Birmingham. They were compensated for 1.5 hours of their time with a one-time payment of £7. All participants had to sign an informed consent form prior to the study. Participants were healthy with no history of head injury, mental health issues, or neurological disorders. The older participants were screened for decline in cognitive functions using the Montreal Cognitive Assessment (MoCA). All of the older participants scored within the normal range ( M score = 27.5, SEM score = .23).
Stimuli and procedure
Two versions of the attentional-white-bear task (Lahav et al., 2012 ; Tsal & Makovski, 2006 ) were used. These versions were the same except where indicated below. Color was defined using RGB color coordinates. The background color of the screen thorough the experiment was gray [100, 100, 100]. Participants were presented with four blocks of 180 trials each. Each block consisted of 80% (144) flanker displays (see Fig. 1a–b ) and 20% (36) two-dot displays trials (see Fig1 c). These displays were randomly intermixed with the exception that two-dot displays could not appear consecutively. Flanker trials consisted of three letters oriented along a diagonal through the center of the screen. Upon being presented with a flanker display, participants had to identify the central letter and respond based on its identity. The central letter was randomly drawn from H , K , C , or S . Participants were required to press the A key if the central letter was an H or a K , and the L key if the central letter was a C or an S . The two flanking letters were also drawn from the same group of four letters, though both distractor letters were always the same within a trial. Therefore, on each trial the distractor letter could be congruent or incongruent with the correct response to the central letter. Since there are four possible central letters and four possible distractor letters, there are 16 possible combinations of central and distractor letters, and each combination appeared an equal number of times in each block (nine repetitions yielding 144 flanker trials per block).
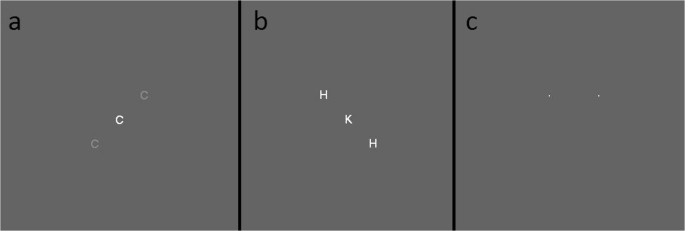
a The low-contrast display with distractors in the upper right/lower left flanker configuration. b The equal-contrast display with distractors in the upper left/lower right flanker configuration. c The dot display
On two of the four blocks, the distractors were located toward the upper left and bottom right of the central letter, and on the other two blocks the distractors were located toward the upper right and lower left. The center of the distractors was 1.58 (1.122degrees of vertical visual angle) degrees of visual angle from the center of the central letter. Critically, within a block, the flanker configurations never changed. Letters were displayed in 14-point Arial font. The central letter was white [255, 255, 255]. As mentioned earlier, there were two versions of this task. In each version the contrast of the distractor letters relative to the background were different. In the equal-contrast version, the distractor letters were white [255, 255, 255], the same color as the central target. In the low-contrast version, the distractor letters were a light gray that was defined as 25% of the difference (rounded up) between the background color and white [139, 139, 139]. The phenomenological effect is that in the low-contrast version of the task, the distractors are harder to see because they blend in more with the background.
The two-dot displays consisted of two white dots (one pixel wide) that, during the main experiment, appeared simultaneously at the two possible top distractor positions (1.58 degrees of visual angle from the center of the screen to the top left or the top right). Thus, one of the dots appeared in the same location as the “upper” distractor letters in the flanker task in that block. Participants were instructed to judge which dot they perceived to appear first. To indicate that the left dot appeared first, they would press the S key. To indicate that the right dot appeared first, they would press the K key. To enhance the likelihood participants will make a genuine attempt to judge the temporal order, during the practice trials one of the two dots would appear 50 ms prior to the second dot. However, during the actual experimental run, the two dots appeared simultaneously.
Every trial began with 500 ms of a fixation cross presented at the center of the screen. The fixation cross was black [0, 0, 0]. Next, there was a 500-ms blank interval. Finally, the appropriate stimulus (flanker display or dots, depending on the trial) was displayed until a response was made. Participants were given the chance to rest in between blocks for as long as they wanted, though no one took a break for more than a few minutes (<5 min). Each session began with 20 practice trials consisting of 16 flanker trials and four dot trials. During the practice, participants received visual feedback such that if they made an error on the flanker task, after their response the following fixation cross would turn red for the first 250 ms it was displayed, and then turn black for another 250 ms.
Experiment 1: Results
Flanker-task performance.
Response time in ms (RT) and accuracy rate (i.e., proportion of correct responses) were measured as dependent variables for the flanker task. The response-time data was cleaned to account for outliers (±2 SD s). Cutoff points were calculated independently for congruent and incongruent responses for each participant. Within these conditions, the outlier analysis was conducted at the smallest cell level. For the younger participants, this resulted in the loss of an average of 4.41% ( SEM = .24%) of the equal-contrast response time data and 4.39% (.22%) of the low-contrast response-time data, per participant. For the older participants, this resulted in the loss of an average of 4.30% ( SEM = .22%) of the equal-contrast response-time data and 4.30% (.19%) of the low-contrast response-time data, per participant. All results reported as mean ± standard error of the mean ( SEM ).
Response-time data were analyzed using a repeated-measures ANOVA, with contrast (low vs. regular), flanker configuration (upper left/lower right vs. upper right/lower left), and congruency (congruent vs. incongruent) as within-subjects factors, and age group (younger vs. older) as a between-subjects factor. As there was no main effect of flanker configuration, F (1, 41) = .711, p = .404, and no significant interactions with flanker configuration (all p s > .261, all F s < 1.298), we collapsed the data across flanker configuration and conducted a repeated-measures ANOVA including contrast, congruency, and age group (see Fig. 2 ).
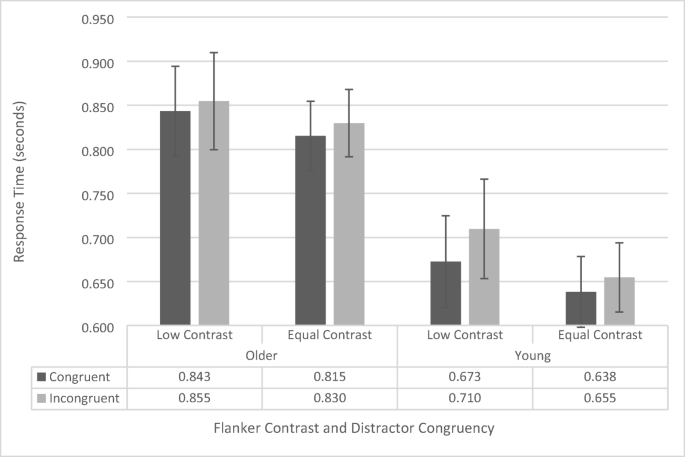
Flanker-task response-time data as a function of congruency, flanker configuration, and age group. Error bars reflect standard error of the mean
The analysis revealed a significant main effect of congruency, F (1, 41) = 18.697, p < .001, \({\eta}_p^2\) = .313, as across both groups participants responded faster for congruent trials (742 ms ± 31 ms) than for incongruent trials (762 ms ± 32 ms). There was also a significant main effect of age group, F (1, 41) = 7.004, p = .011, \({\upeta}_{\mathrm{p}}^2\) = .146, as younger participants were generally faster (669 ms ± 44 ms) than the older participants (836 ms ± 45 ms) were. The main effects of contrast, F (1, 41) = 3.289, p = .077, approached, but was not significant (low contrast: 770 ms ± 38 ms, equal contrast: 734 ms ±28 ms). None of the interactions were significant (all p s > 0.136, all F s < 2.310).
The accuracy data were analyzed using a repeated-measures ANOVA, with contrast (low vs. regular), flanker configuration (upper left/lower right vs. upper right/lower left), and congruency (congruent vs. incongruent) as within-subjects factors, and age group (younger vs. older) as a between-subjects factor. There was no main effect of flanker configuration, F (1, 41) = 2.734, p = .106, and there were no significant interactions with flanker configuration, although it should be noted that the four-way interaction approached significance, F (1, 41) = 3.164, p = .083 (all other p s > .196 and all other F s < 1.728). Therefore, we collapsed the data across flanker configuration and conducted a repeated-measures ANOVA including contrast, congruency, and age group (see Fig. 3 ).
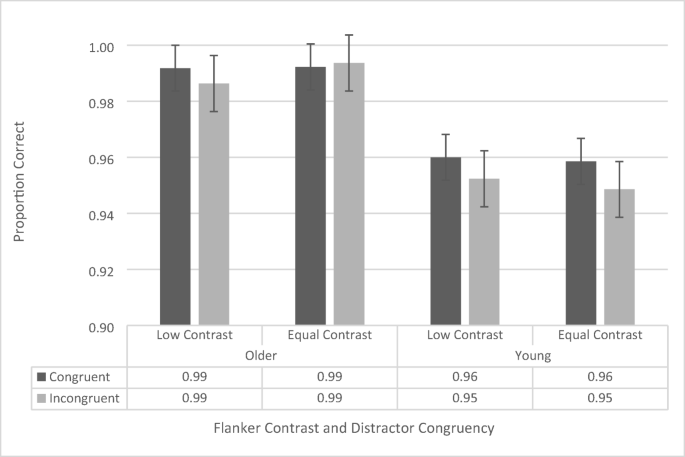
Flanker-task accuracy data as a function of congruency, flanker configuration, distractor contrast, and age group. Error bars reflect standard error of the mean
The repeated-measures ANOVA revealed a significant main effect of congruency, F (1, 41) = 8.61 , p = .005, \({\upeta}_{\mathrm{p}}^2\) = .174, as participants across both groups were more accurate during congruent trials (97.6% ± 0.3%) than during incongruent trials (97.0% ± 0.4%). The analysis also revealed a significant main effect of age group, F (1, 41) = 28.786, p < .001, \({\upeta}_{\mathrm{p}}^2\) = .412, with the older participants performing more accurately (99.1% ± 0.5%) than the younger ones (95.5% ± 0.5%). The main effect of contrast, F (1, 41) = .036, p = .851, was not significant. The two-way interaction between age group and congruency approached significance, F (1, 41) = 3.343, p = .075, as the congruency effect for the younger participants (congruent: 95.9% ± 0.5%, incongruent: 95.0% ± 0.5%) trended toward being larger than that of the older participants (congruent: 99.2% ± 0.4%, incongruent: 99.0% ± 0.5%). None of the other interactions were significant (all p s > .128, all F s < 2.412).
Temporal-order-judgment task
The AWB effect (see Fig. 4 ) is indexed by the difference in likelihood of identifying the left or right dots as appearing first as a function of flanker position. Thus, we have used a repeated-measures ANOVA (similar to Tsal & Makovski, 2006 ) on the proportion of “left” responses with contrast (low vs. equal) and flanker configuration (upper left vs. upper right) as within-subjects factors and age group (younger vs. older) as a between-subjects factor (see Fig. 4 ). The ANOVA revealed a main effect of flanker configuration, F (1, 41) = 26.056, p < .001, \({\eta}_p^2\) = .389, as participants across both groups had a greater proportion of left responses when a flanker appeared in the upper left position (65.2% ± 3.4%) compared with the upper right position (41.1% ± 4.2%). This is the standard AWB effect. The main effects of contrast, F (1, 41) = 1.48, p = .231, and age group, F (1, 41) = .067, p = .796, were not significant. There is a common misconception that simple main effects cannot be interpreted if there is a nonsignificant interaction. However, this is not the case. According to Wei, Carroll, Harden, and Wu ( 2012 ), the simple effects are a reasonable and interpretable analysis when there is a nonsignificant interaction given that the main effect of one of the variables is significant. As the main effect of flanker configuration is significant, and the Age × Flanker Configuration interaction is the primary effect of interest in this study, we further confirmed these results by calculating simple main effects, which revealed that both the younger (upper left flanker: 65.4% ± 4.9%, upper right flanker: 42.4% ± 6.0%, p = .001) and older (upper left flanker: 64.9% ± 4.8%, upper right flanker: 39.8% ± 5.8%, p < .001) cohorts independently showed a significant white-bear effect.
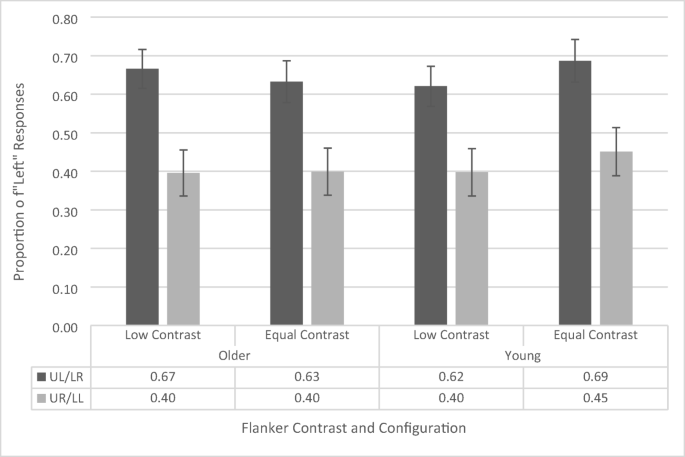
Proportion of “left” responses on temporal-order-judgement task as a function of flanker configuration, distractor contrast, and age group. UL/LR refers to upper left/lower right and UR/LL refers to upper right/lower left. Error bars reflect standard error of the mean
A marginally significant interaction between contrast and age group was also found, F (1, 41) = 4.079, p = .05, \({\eta}_p^2\) = .090. Analysis of simple main effects revealed that this was driven by a lower proportion of left responses in the low-contrast condition (50.9%, 4.6%) than in the equal-contrast condition (56.9%, 4.3%) for the younger participants ( p = .029), but no difference in proportion of left responses between low (53.1%, 4.5%) and equal (51.6%, 4.2%) contrast conditions for the older participants ( p = .569). This interaction suggests that younger participants were more sensitive to the contrast manipulation. However, since this was not with respect to the flanker configuration, it does not reflect AWB-related processes. Indeed, two-tailed one-sample t tests revealed that, for the younger participants, the overall proportion of left responses (across both flanker configurations) in the low-contrast condition, t (19) = .177, p = .861, and the equal-contrast condition, t (19) = 1.63, p = .119, did not differ significantly from .50 (i.e., chance performance). This supports the notion that the increased tendency of the younger participants to respond left during the equal-contrast trials cannot account for the white-bear effect in that condition. The remaining interactions did not reach significance levels (all p s > .551, all F s < .361).
This data suggest that both younger and older participants exhibit the standard white-bear effect and that the magnitude of the effect is consistent across age groups. To further interrogate this result, we conducted a Bayesian repeated-measures ANOVA (using JASP; Jarosz & Wiley, 2014 ; Marsman & Wagenmakers, 2017 ; Wagenmakers, 2007 ) and calculated the inclusion Bayes factor for each main effect and interaction. The inclusion Bayes factor is a measure of the extent to which a factor of interest should be included in a model of the data, compared with all other models. The inclusion Bayes factor for the main effect of flanker configuration was 1.197e+9, indicating that there is very strong/decisive evidence in favor of a model that includes flanker configuration to explain the data. However, the inclusion Bayes factors provides less than weak/anecdotal evidence for all other main effects and interactions (all BF Inclusion < .138; it should be noted that the terms very strong/decisive and weak/anecdotal are based on guidelines detailed in Jarosz & Wiley, 2014 ). This supports the conclusion that in Experiment 1, both younger and older participants exhibited a white-bear effect with a similar magnitude.
Experiment 1: Discussion
We found no age difference in the temporal-order-judgment task (the AWB statistic). Across both younger and older participants, a similar tendency was observed to perceive the dot appearing in an expected flanker position as appearing first. At first glance, proactive allocation of attention prior to inhibition appears to be intact in aging populations. As expected, interference from the flankers was observed in both RTs and accuracy (main effect of congruency) for all participants regardless of age. The group difference in RT was also expected, with older participants typically responding overall slower. The group difference in accuracy with older participants performing overall more accurately than younger participants is also quite common in the aging literature. These findings may represent a simple speed–accuracy trade-off in the two groups or, alternatively, may be attributable to a generalized age-related deficit in processing speed (Salthouse, 2000 ; Salthouse & Meintz, 1995 ; Verhaeghen & De Meersman, 1998 ). Critically, the data did not point to increased interference in old age in this task and, if anything, the marginally significant interaction between age and congruency reported for the accuracy data suggested more interference in younger compared with older participants.
Although the lack of a between-group inhibition deficit is arguably unexpected given the ample evidence that proactive inhibition is impaired in aging (Jimura & Braver, 2010 ; Paxton et al., 2008 ), it does fit with previous studies using the flanker task in older participants who did not show increased interference in older participants (Fernandez-Duque & Black, 2006 ; Kawai, Kubo-Kawai, Kubo, Terazawa, & Masataka, 2012 ; Salthouse, 2010 ; Verhaeghen, 2011 ). One possibility is that flanker tasks are not sensitive enough to detect age-related differences in inhibition, as a number of previous studies using this task have also failed to find group differences. For instance, Salthouse ( 2010 ) found no age-related behavioral differences in flanker-task performance and questioned its utility as a measure of executive function and inhibition (also see Fernandez-Duque & Black, 2006 ; Kawai et al., 2012 ; Verhaeghen, 2011 ). A further possibility for the comparable congruency effects here could be that performance in the flanker task reflects reactive (rather than proactive) inhibition, which is supposedly intact in old age (Braver, Satpute, Rush, Racine, & Barch, 2005 ; Jimura & Braver, 2010 ; Paxton et al., 2008 ).
That being said, a review of our study and others revealed that the studies that fail to find age differences may have been not sensitive to differences in inhibition because the task was simply too easy. In these tasks the flanker stimuli were presented for up to 2,000 ms (Kawai et al., 2012 ), 1,500 ms (Salthouse, 2010 , Study 1), or 500 ms (Fernandez-Duque & Black, 2006 ; in this study, the flanker was also combined with a cueing task). In our study, the flanker stimuli were presented until a response was made. Although this was consistent with the original AWB studies, this may not be the appropriate timing for this study where we are interested in proactive allocation of attention prior to inhibition. Under these long presentation times, even though proactive inhibition is clearly taking place (i.e., the white-bear effect), subsequent inhibition of the flankers may not be necessary, because there is enough time to process the flankers and the target sequentially, rather than simultaneously. Therefore, in the next set of experiments we assessed the possibility that long presentation times may be making flanker tasks and the AWB task not sensitive to age-related inhibition deficits. We conducted the same attentional-white-bear study across younger and older participants, but this time the presentation time for the flanker stimuli was limited to either 100 ms or 200 ms.
Experiments 2a and 2b: Methods
Twenty-one younger and 21 older participants took part in this study, each completing four successive behavioral experiments (low and regular contrast with 200 ms or 100 ms presentation); however, because of poor performance (>20% invalid button presses during the temporal-judgement task in either presentation time condition), one older participant was excluded from the study entirely. One younger participant was excluded from the 200-ms analysis. One additional older participant and a different younger participant was excluded from the 100-ms analysis. For the 200-ms experiments, 20 younger participants ( M age = 25.10 years, SEM age = 0.746, age range: 20–32 years, 17 females) and 20 older participants ( M age = 71.75, SEM age = .99, age range: 65–81 years, 13 females) were included in the analysis. For the 100-ms experiments, 20 younger participants ( M age = 24.85 years, SEM age = 0.805, age range: 19–32 years, 17 females) and 19 older participants ( M age = 71.57 years, SEM age = 1.03, age range: 65–81 years, 12 females) were included in the analysis. Younger participants were recruited from the undergraduate population in the School of Psychology at the University of Birmingham, UK. They were compensated for their participation with course credits. The older participants were recruited from a volunteer pool maintained by the School of Psychology at the University of Birmingham. They were compensated for 1.5 hours of their time with a one-time payment of £7. All participants had to sign an informed consent form prior to the study. Participants’ were healthy with no history of head injury, mental health issues, or neurological disorders. The older participants were screened for decline in cognitive functions using the Montreal Cognitive Assessment (MoCA). All of the older participants scored within the normal range (>26).
The stimuli and procedure for this study were nearly identical to the procedure described in the Methods section of Experiment 1. The only differences were that flanker stimuli were presented for either 100 ms or 200 ms, instead of until response. After the presentation time was up, the screen displayed a fixation cross until a response was made. The stimulus timing condition was blocked, and conditions were presented to participants in the following order: low-contrast flankers with 200-ms presentation time; equal-contrast flankers with 200-ms presentation time; low-contrast flankers with 100-ms presentation time; equal-contrast flankers with 100-ms presentation time. This order was selected so that participants could not establish strategies during the faster 100-ms condition that they could then easily deploy during the 200-ms condition.
Experiment 2a: 200-ms presentation-time results
Response time (RT) in ms and accuracy rate (i.e., proportion of correct responses) were measured as dependent variables for the flanker task. The response-time data were cleaned to account for outliers (±2 SD s). Cutoff points were calculated independently for congruent and incongruent responses for each participant. Within these conditions, the outlier analysis was conducted at the smallest cell level. For the younger participants, this resulted in the loss of an average of 4.38% ( SEM = .21%) of the equal-contrast response-time data and 3.94% (.39%) of the low-contrast response-time data, per participant. For the older participants, this resulted in the loss of an average of 3.73% ( SEM = .35%) of the equal-contrast response-time data and 3.78% (.25%) of the low-contrast response-time data, per participant. All results reported as mean ± standard error of the mean ( SEM ).
Response-time data were analyzed using a repeated-measures ANOVA, with contrast (low vs. regular), flanker configuration (upper left/lower right vs. upper right/lower left), and congruency (congruent vs. incongruent) as within-subjects factors, and age group (younger vs. older) as a between-subjects factor. As there was no main effect of flanker configuration, F (1, 38) = .840, p = .365) and no significant interactions with flanker configuration (all p s > .145, all F s < 2.214), we collapsed the data across flanker configuration and conducted a repeated-measures ANOVA including contrast, congruency, and age group (see Fig. 5 ).
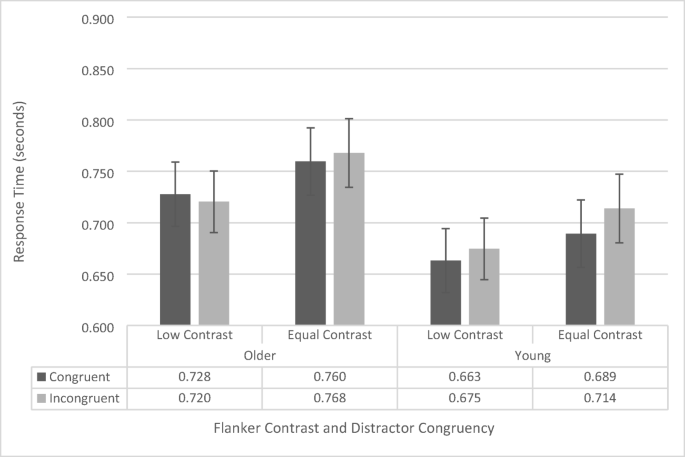
200-ms stimulus presentation-time flanker-task response-time data as a function of congruency, flanker contrast, and age group. Error bars reflect standard error of the mean
The main effect of congruence was significant, F (1, 38) = 4.27, p = .045, \({\eta}_p^2\) = .101, with participants responding faster on congruent (710 ms ± 22 ms) trials than to incongruent (719 ms ± 21 ms) trials. The main effect of age group was not significant, F (1, 38) = 1.81, p = .186. The interaction between congruence and age group approached significance, F (1, 38) = 3.87, p = .057, \({\eta}_p^2\) = .092. To further investigate this potential effect, we assessed simple main effects, which revealed that this effect is driven by a significant congruency effect for younger participants ( p = .007; congruent RT: 676 ms ± 31 ms; incongruent RT: 694ms ± 31 ms), but no significant difference in congruence for the older participants, who showed virtually identical RTs for congruent (744 ms ± 31 ms) and incongruent (744 ± 31 ms) displays. The interaction between contrast and congruence also approached significance, albeit to a lesser degree, F (1, 38) = 3.006, p = .091, \({\eta}_p^2\) = .073. Simple main effects revealed this was driven by a significant congruency effect during regular-contrast trials ( p = .007; congruent RT: 725 ms ± 23 ms; incongruent RT: 741 ms ± 24 ms), but no significant difference in congruence during low-contrast trials ( p = .750; congruent RT: 696 ms ± 22 ms; incongruent RT: 698 ms ± 21 ms). The interaction between contrast and age group, F (1, 38) = .130, p = .720, and the three-way interaction, F (1, 38) = .024, p = .878, were not significant.
For the accuracy data, we conducted a repeated-measures ANOVA, with contrast (low vs. regular), flanker configuration (upper left/lower right vs. upper right/lower left), and congruency (congruent vs. incongruent) as within-subjects factors and age group (younger vs. older) as a between-subjects factor. As there was no main effect of flanker configuration, F (1, 38) = .899, p = .349, or significant interactions with flanker configuration (all p s > .284, all F s < 1.183), we collapsed the data across flanker configuration and conducted a repeated-measures ANOVA including contrast, congruency, and age group (see Fig. 6 ).
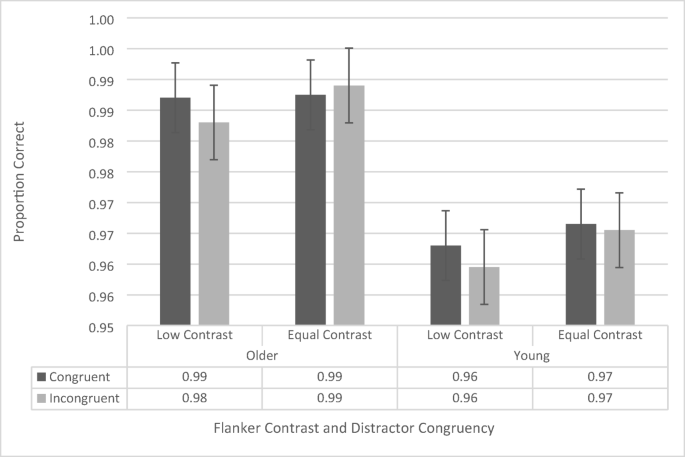
200-ms stimulus presentation-time flanker-task proportion-correct data as a function of congruency, flanker contrast, and age group. Error bars reflect standard error of the mean
The ANOVA revealed a significant main effect of age group, F (1, 38) = 7.954 , p = .008, \({\eta}_p^2\) = .173, in which older participants (98.7% ± 0.6%) were more accurate than younger participants (96.4% ± 0.6%) were. The main effect of contrast, F (1, 38) = 1.90, p = .176, and congruence, F (1, 38) = .844 , p = .364, were not significant. None of the interactions were significant (all p s > .323, all F s < 1.002).
Next, we assessed the presence and magnitude of the attentional-white-bear effect (see Fig. 7 ). We conducted a repeated-measures ANOVA on the proportion of “left” responses, with contrast (low vs. equal) and flanker configuration (upper left vs. upper right) as within-subjects factors, and age group (younger vs. older) as a between-subjects factor. The ANOVA revealed a main effect flanker configuration, F (1, 38) = 7.196, p = .011, \({\eta}_p^2\) = .159, as participants across both groups had a greater proportion of left responses when a flanker appeared in the upper left position (52.7% ± 3.6%) compared with the upper right position (44.3% ± 3.9%). This is the standard AWB effect. The main effects of contrast, F (1, 38) = .041, p = .840, and age group, F (1, 38) = 1.358, p = .251, were not significant. In contrast to Experiment 1, there were no significant interactions (all p s > .185, all F s < 1.823). To be consistent with our analysis of Experiment 1 and also to further investigate our effect of interest, we calculated the simple main effects of the Age × Distractor Location interaction (Note: The Age × Flanker Configuration interaction is nonsignificant, but the main effect of flanker configuration is significant). This analysis revealed that the younger participants (upper left flanker: 58.2% ± 5.2%; upper right flanker: 46.8% ± 5.6%; p = .014) showed a significant white-bear effect, but the older participants did not (upper left flanker: 47.2% ± 5.2%; upper right flanker: 41.8% ± 5.6%; p = .228).
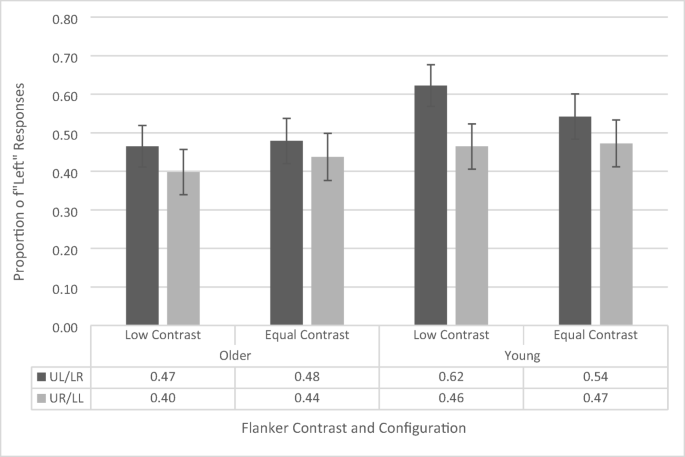
Proportion of “left” responses on temporal-order-judgement task with 200-ms flanker-presentation time as a function of flanker configuration, flanker contrast, and age group. UL/LR refers to upper left/lower right and UR/LL refers to upper right/lower left. Error bars reflect standard error of the mean
However, given the disagreement between the nonsignificant interaction and the simple main effects, this result must be interpreted with care. To further assess this potential effect, we calculated the Bayes factor in support of the alternative hypothesis that there is an interaction between age group and configuration. First, we used JASP to compare the Bayes factor for a model that included just the main effects of age group and flanker configuration (BF 10 = 12.669) and for a model that included the main effects and their interaction (BF 10 = 5.138). Next, we divided the model with the interaction by the model without the interaction (5.138/12.669 = 0.405). This suggests that the observed data are 0.405 times more likely to occur under a model where there is an interaction between age group and configuration (compared with a model where there is no interaction). Consistent with the null hypothesis significance testing, the Bayes factor analysis essentially provides no evidence in favor of the interaction.
Experiment 2b: 100-ms presentation-time results
Response time (RT) in ms and accuracy rate (i.e., proportion of correct responses) were measured as dependent variables for the flanker task. The response-time data were cleaned to account for outliers (±2 SD s). Cutoff points were calculated independently for congruent and incongruent responses for each participant. Within these conditions, the outlier analysis was conducted at the smallest cell level. For the younger participants, this resulted in the loss of an average of 4.23% ( SEM = .22%) of the equal-contrast response-time data and 4.18% (.30%) of the low-contrast response-time data, per participant. For the older participants, this resulted in the loss of an average of 3.95% ( SEM = .29%) of the equal-contrast response-time data and 3.455% (.26%) of the low-contrast response-time data, per participant. All results reported as mean ± standard error of the mean ( SEM ).
Response-time data were analyzed using a repeated-measures ANOVA, with contrast (low vs. regular), flanker configuration (upper left/lower right vs. upper right/lower left), and congruency (congruent vs. incongruent) as within-subjects factors, and age group (younger vs. older) as a between-subjects factor. Although there was a main effect of flanker configuration, F (1, 38) = 5.70, p = .022, \({\eta}_p^2\) = .134, driven by faster responses when the flanker appeared in the upper left/lower right (725 ms ± 22 ms) compared with the upper right/lower left (707 ms ± 21 ms), there were no significant interactions with flanker configuration (all p s > .265, all F s < 1.283). Therefore, we collapsed the data across flanker configuration and conducted a repeated-measures ANOVA including contrast, congruency, and age group (see Fig. 8 ).
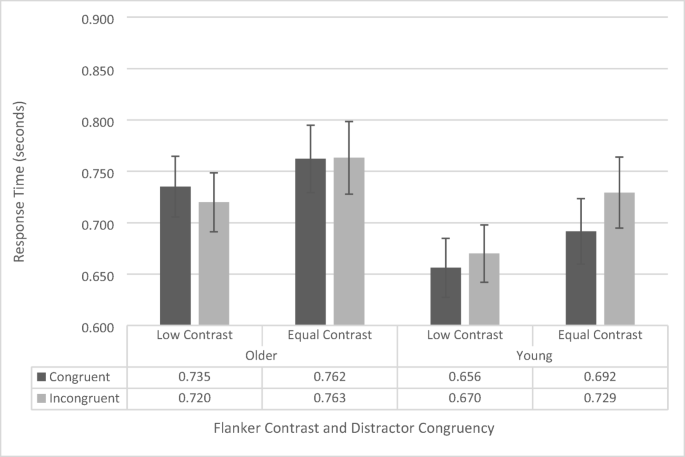
100-ms stimulus presentation-time flanker-task response-time data as a function of congruency, flanker contrast, and age group. Error bars reflect standard error of the mean
The analysis revealed that there was a main effect of contrast, F (1, 38) = 11.407, p = .002, \({\eta}_p^2\) = .236, as across both groups’ participants responded faster when presented with low-contrast flankers (695 ms ± 20 ms) than with equal-contrast flankers (737 ms ± 24 ms). The main effect of congruence was also significant, F (1, 38) = 5.754, p = .022, \({\eta}_p^2\) = .135, as participants responded faster on congruent trials (711 ms ± 21 ms) than on incongruent trials (721 ms ± 21 ms). However, these were qualified by an interaction between contrast and congruence, F (1, 38) = 5.582, p = .024, \({\eta}_p^2\) = .131. Simple main effects revealed that this was driven by faster responses on congruent trials when presented with equal-contrast flankers ( p = .002; congruent: 727 ms ± 23 ms; incongruent: 746 ms ± 25 ms), but no difference in congruency when presented with low-contrast flankers ( p = .907; congruent: 696 ms ± 21 ms; incongruent: 695 ms ± 20 ms). The main effect of age group was not significant, F (1, 38) = 1.916, p = .175, but this too was qualified by an interaction between age group and congruence, F (1, 38) = 17.769, p < .001, \({\eta}_p^2\) = .324. Simple main effects revealed that, like in the 200-ms experiments, this interaction was driven by a significant congruency effect for younger participants ( p < .001; congruent RT: 674 ms ± 29 ms; incongruent RT: 700 ms ± 30 ms), but no significant difference in congruence for the older participants ( p = .213; congruent RT: 749 ms ± 30 ms; incongruent RT: 742 ms ± 30 ms). Neither the interaction between contrast and group nor the three-way interaction were significant (both p s > .619).
Accuracy data were analyzed using a repeated-measures ANOVA, with contrast (low vs. regular), flanker configuration (upper left/lower right vs. upper right/lower left), and congruency (congruent vs. incongruent) as within-subjects factors, and age group (younger vs. older) as a between-subjects factor. The main effect of flanker configuration was not significant, F (1, 38) = .114, p = .738, but there was a marginally significant four-way interaction, F (1, 38) = 4.148, p = .049, \({\eta}_p^2\) = .101. To assess if this interaction is meaningful, we compared the Bayes factor of a model with and without the four-way interaction (BF 10 -with/BF 10 -without = .011/.006 = 1.833). The Bayes factor (BF 10 ) is a measure of how likely a set of data is under the alternative hypothesis compared with the null hypothesis. This suggested that the data were 1.833 times more likely under a model with the four-way interaction than without, constituting weak or anecdotal evidence (Jarosz & Wiley, 2014 ) in favor of the four-way interaction.
Given the limited evidence that the four-way interaction is meaningful, and to be consistent with the other analyses throughout the paper, we collapsed the data across flanker configuration and conducted a repeated-measures ANOVA including contrast, congruency, and age group (see Fig. 9 ). The ANOVA revealed a significant main effect of age group, F (1, 38) = 12.815, p = .001, \({\eta}_p^2\) = .257, in which older participants (99.1% ± 0.5%) were more accurate than in younger participants (96.6% ± 0.5%). There was also a main effect of congruence, F (1, 38) = 13.817, p = .001, \({\eta}_p^2\) = .272) as participants were more accurate on congruent trials (98.2% ± 0.3%) than on incongruent trials (97.4% ± 0.4%). However, these effects were qualified by a significant interaction between age group and congruence, F (1, 38) = 7.619, p = .009, \({\eta}_p^2\) = .171. Simple main effects revealed that this was driven by better performance in the congruent condition (97.3% ± 0.4%) than in the incongruent condition (95.9% ± 0.6%) for the younger participants ( p < .001), but no difference in performance (congruent: 99.2% ± 0.4%; incongruent: 98.9% ± 0.6%) for the older participants ( p = .508). The main effect of contrast, F (1, 38) = .165, p = .687, and none of the interactions were significant (all p s > .252).
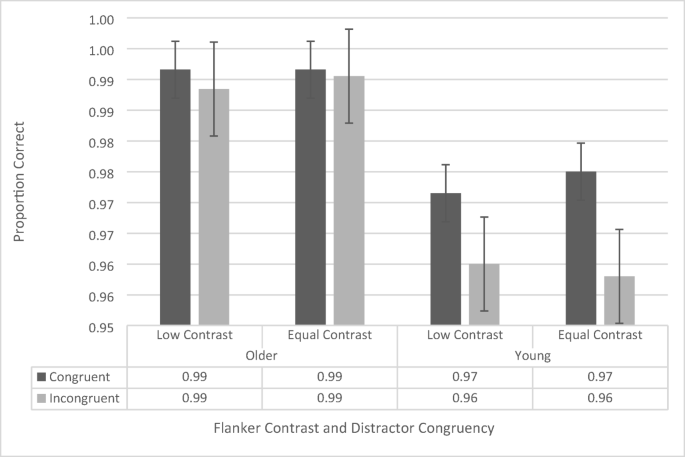
100-ms stimulus presentation-time flanker-task proportion-correct data as a function of congruency, flanker contrast, and age group. Error bars reflect standard error of the mean
A repeated-measures ANOVA (see Fig. 10 ) on the proportion of “left” responses, with contrast (low vs. equal) and flanker configuration (upper left vs. upper right) as within-subjects factors and age group (younger vs. older) as a between-subjects factor (see Fig. 9 ). The ANOVA revealed a main effect of flanker configuration, F (1, 38) = 12.896, p = .001, \({\eta}_p^2\) = .258, as participants across both groups had a greater proportion of left responses when a flanker appeared in the upper left position (59.0% ± 3.7%) compared with the upper right position (45.6% ± 3.5%). This is the standard AWB effect.
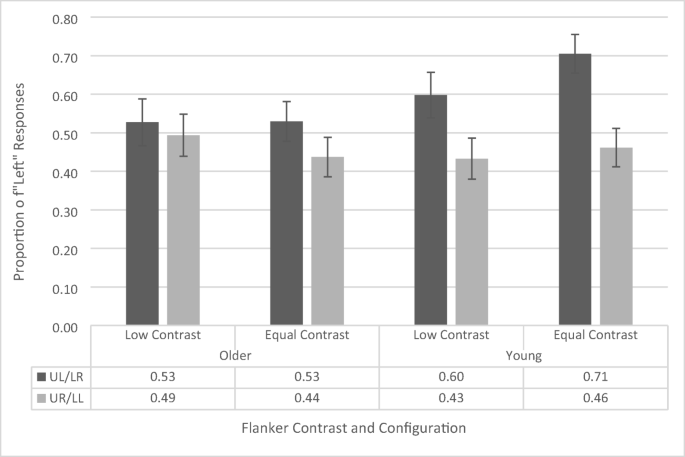
Proportion of “left” responses on the temporal-order-judgement task with 100-ms flanker presentation time as a function of flanker configuration, flanker contrast, and age group. UL/LR refers to upper left/lower right and UR/LL refers to upper right/lower left. Error bars reflect standard error of the mean
The main effects of contrast, F (1, 38) = 1.344, p = .254, and age group, F (1, 38) = .727, p = .399, were not significant. However, this was qualified by a significant interaction between contrast and age group, F (1, 38) = 7.317, p = .010, \({\eta}_p^2\) = .165. Simple main effects revealed that this interaction was driven by a lower proportion of left responses in the low-contrast condition (51.6% ± 4.8%) than in the equal-contrast condition (58.3% ± 4.1%) for the younger participants ( p = .009), but no difference in proportion of “left” responses between low (51.1% ± 4.9%) and equal (48.3%± 4.2%) contrast conditions for the older participants ( p = .287). This is the same effect that was found in Experiment 1, but not in Experiment 2a, suggesting that younger participants may be more sensitive to the contrast manipulation. A two-tailed one-sample t test revealed that, for the younger subjects, the overall proportion of left responses (across both flanker configurations) in the low-contrast condition, t (19) = −.340, p = .738, did not differ significantly from .50 (i.e., chance performance). However, the overall proportion of left responses did differ in the equal-contrast condition, t (19) = 2.35, p = .029, d = .52, suggesting that younger participants exhibited a bias for responding “left” during the equal-contrast trials. However, since this effect is not with respect to the flanker configuration, it does not reflect AWB-related processes and does not affect our ability to interpret AWB effects.
Critically, the interaction between flanker configuration and age group approached significance, F (1, 38) = 3.611, p = .065, \({\eta}_p^2\) = .089. We further assessed this interaction with simple main effects since the main effect of flanker configuration was significant (Wei et al., 2012 ). Simple main effects revealed that younger participants appeared to respond left more often when flankers were in the upper left (65.1% ± 5.2%) compared with the upper right (i.e., the standard white-bear effect; p < .001; 44.7% ± 4.9%), whereas older participants showed no difference in left responses due to flanker configuration ( p = .245; 52.8% ± 5.3%; 46.6% ± 5.0%, for upper left and upper right flankers, respectively). However, given the nonsignificant interaction, this result must be interpreted with care. To further assess this potential effect, we calculated the Bayes factor in support of the alternative hypothesis that there is an interaction between age group and configuration. First, we used JASP to compare the Bayes factor for a model that included just the main effects of age group and flanker configuration (BF 10 = 3,308.896) and for a model that included just the main effects and their interaction (BF 10 = 17,518.914). Next, we divided the model with the interaction by the model without the interaction (17,518.914/3,308.896 = 2.94). This suggests that the observed data is 2.94 times more likely to occur under a model where there is an interaction between age group and configuration (compared with a null model). According to Jarosz and Wiley ( 2014 ), this value is right on the cutoff between being considered weak/anecdotal evidence (<3) or positive/substantial evidence (>3).
The interaction between contrast and flanker configuration, F (1, 38) = 3.266, p = .079, as well as the three-way interaction, F (1, 38) = .070, p = .792, were not significant.
Allocation versus inhibition
In the 100-ms condition, older participants showed differences in both allocation of attention to expected distractors (absence of a white-bear effect) and inhibition of these distractors (absence of a congruency effect). Here, we tested if there was a relationship between these two variables. To reflect the allocation of attention, we calculated a white-bear metric, which was the difference in “left” responses between flankers in the upper left or upper right corner of the stimuli. Higher values of this metric reflect a larger white-bear effect and presumably more allocation of attention toward the expected flankers prior to their occurrence. To reflect the inhibition of attention, we calculated the response-time congruency effect, which was the difference in response time between congruent and incongruent trials (Incongruent − Congruent). Higher values of this metric reflect a larger congruency effect and ostensibly less inhibition of the distractors. It should be noted here that the white-bear and congruency effects reflect performance on different trials with distinct tasks (temporal order judgment vs. flanker), therefore this is an indirect measure of the relationship between attention allocation and inhibition. We calculated these values separately for the low-contrast and equal-contrast conditions.
For the low-contrast trials, the correlation between the white-bear metric and the congruency effect was not significant for both the younger, r (18) = .011, 95% CI Upper = .452, 95% CI Lower = −.433, p = .962, and older, r (17) = −.342, 95% CI Upper = .133, 95% CI Lower = −.689, p = .151, participants. For the equal-contrast trials, the correlation was not significant for the younger participants, r (18) = .065, 95% CI Upper = .494, 95% CI Lower = −.388, p = .784, but it was significant for the older participants, r (17) = .524, 95% CI Upper = .790, 95% CI Lower = .092, p = .021 (see Fig. 11 ). This correlation suggests that for the older participants, a larger white-bear effect was associated with a larger congruency effect. In other words, more effective proactive allocation of attention in the temporal-order-judgment task was associated with less effective inhibition in the flanker task.
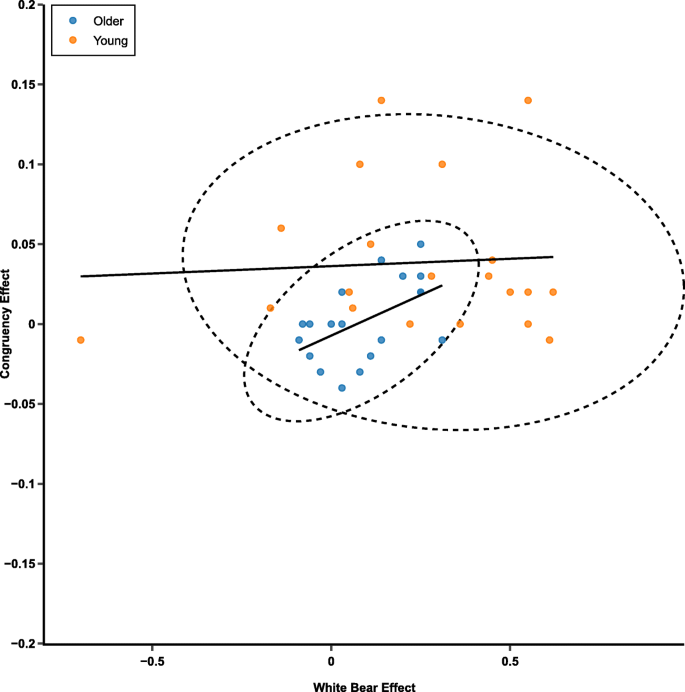
Scatterplot reflecting the white bear effect and the congruency effect for equal-contrast flankers presented for 100 ms. Larger values of the congruency effect reflect worse inhibition. Larger values of the white bear statistic reflect more allocation of attention. The dotted lines reflect 95% confidence ellipses based on a t distribution. (Color figure online)
However, these results must be interpreted with care because a sample size of 21 per group is small for a correlational analysis. To further assess this effect, we used JASP to calculate the Bayes factor (Jarosz & Wiley, 2014 ; Marsman & Wagenmakers, 2017 ; Wagenmakers, 2007 ) for the equal-contrast correlation results for the younger and older cohort independently. The Bayes factor is a measure of how much more likely is a set of data under the alternative or null hypothesis. For the older participant data, the alternative hypothesis is a positive correlation, and the null hypothesis is no correlation. The Bayes factor in favor of the alternative hypothesis was 6.625, suggesting that the observed data is 6.625 times more likely to occur under a model where there is a positive correlation between the congruency effect and the white-bear effect than one in which there is no correlation. This is considered positive or substantial evidence in favor of the alternative hypothesis (a positive correlation; based on guidelines detailed in Jarosz & Wiley, 2014 ). For the younger participant data, we calculated the inverse Bayes factor such that the alternative hypothesis is no correlation and the null hypothesis is any (positive or negative) correlation. The inverse Bayes factor in favor of the alternative hypothesis was 3.488, suggesting that the observed data is 3.488 times more likely to occur under a model where there is no correlation between the congruency effect and the white-bear effect than one in which there is a correlation (positive or negative). This value is right on the cutoff between being considered weak/anecdotal evidence (<3) or positive/substantial evidence (>3; based on guidelines detailed in Jarosz & Wiley, 2014 ).
Although the Bayes factor analysis does increase our confidence in the correlation for the older participants, because of the sample size, the correlation analysis is exploratory/speculative at best. It does, however, suggest that future studies of the attentional-white-bear paradigm should assess the relationship between allocation and inhibition of attention with a larger sample size to confirm or disconfirm these findings and better understand the assumed relationship between allocation and inhibition in the attentional-white-bear paradigm (and in some inhibition processes in general).
Experiment 2a and 2b: Discussion
When the flanker stimuli were presented for 200 ms, both younger and older participants exhibited a white-bear effect of similar magnitude, similar to Experiment 1. However, in contrast to Experiment 1, only the younger participants exhibited a congruency effect (faster RTs on congruent trials). Furthermore, older participants were more accurate than younger participants. While increased accuracy in aging populations is often attributed to speed–accuracy trade-offs, in this case there was no difference in overall response time between the age groups, making this interpretation unlikely. In fact, the lack of a congruency effect for the older participants suggests that they are better able to suppress the distractors than younger participants are, which in turn may have led to the improvement in accuracy.
When the flanker stimuli were presented for 200 ms or 100 ms, the younger participants exhibited a standard white-bear effect, but the older participants appear to show an attenuated white-bear effect. Although neither of the Age × Flanker configurations reached the 0.05 significance level, the simple main effects analysis clearly showed a difference in performance. Further, for the 100-ms condition, the Bayes factor analysis provided some additional support that there was an age-related difference in performance. Notably, the difference in performance appears to be larger in the 100-ms condition, ostensibly due to it being a more difficult condition. Overall, these data suggest that older participants were not effectively proactively allocating attention to the flankers, whereas younger participants were. In both the 200-ms and 100-ms conditions, younger participants exhibited a congruency effect during the flanker task, but older participants did not. We further investigated this lack of a congruency effect and white bear by looking at the relationship between the two in the 100-ms condition (since the difference in the white bear was more pronounced). Crucially, the correlation analysis suggested that the white-bear effect was actually associated with increased distractor interference for the older participants, but not for the younger participants. Although, given the small sample size, this analysis is exploratory at best.
Furthermore, in both the 200-ms and 100-ms experiments, the low-contrast condition did not induce a congruency effect, whereas the equal-contrast condition did, suggesting that the flankers in the low-contrast condition did not require significant attentional resources to process and inhibit. This is consistent with prior literature showing that dimmer flankers lead to reduced conflict cost (Wyatt & Machado, 2013 ; Zeischka, Coomans, Deroost, Vandenbossche, & Soetens, 2011 , Experiment 2).
General discussion and conclusions
In the present study, we aimed to assess proactive allocation of attention to expected distractor location in old age. While previous studies have pointed to impaired proactive control in old age, such studies focused almost solely on measures of inhibition rather than allocation. To test this issue, we used the attentional-white-bear paradigm (AWB; Tsal & Makovski, 2006 ), which provides a direct measure of the proactive allocation of attention to expected distractor locations. In Experiment 1, we provided a direct replication of Tsal and Makovski ( 2006 ), in both younger and older participants. Both groups demonstrated intact proactive allocation of attention to the expected distractor location, and to the same degree. However, in Experiment 1 participants had an unlimited amount of time to see the stimuli. In Experiments 2a and 2b, we taxed the attentional system more by decreasing the flanker stimulus presentation time. Under these conditions, an age-related deficit in the allocation of attention toward expected distractors emerged. In particular, older participants showed reduced allocation of attention compared with their younger counterparts. A secondary finding was that this reduced allocation of attention during the temporal-order-judgment task was associated with better inhibition during the flanker task, but only for the older participants.
Although the proactive allocation of attention elicited by the AWB yields a seemingly benign effect in younger controls, that does not mean it is not the same mechanism engaged by other processes (albeit used to a different end). This is crucial because our main finding is that allocation of attention was reduced in our older population when the attention system was taxed sufficiently. Therefore, allocation of attention may be impaired in other processes as well, such as visual marking. Studies of visual marking and aging have shown no age-related differences in performance in static displays (Kramer & Atchley, 2000 ; Kramer & Kray, 2006 ), but, notably, they have emerged in dynamic displays (Becic et al., 2007 ; Watson & Maylor, 2002 ). This may be attributed to the fact that dynamic displays are more difficult than static displays, similar to how differences in the AWB did not emerge until the stimulus presentation time was reduced. It has been shown that, in visual marking studies, the inhibition in the preview search acts via a location-based inhibitory bias against the to-be-ignored distractors (Humphreys et al., 2004 ; Olivers et al., 2006 ; Watson & Humphreys, 2004). Therefore, since a moving target (i.e., a dynamic display) may only be in a location for a limited amount of time, this may effectively act as a short stimulus presentation time at each given location (inducing age-related differences like in our study). This is speculative, of course, but future research could assess these possibilities.
A secondary finding was that, for the older participants (during equal-contrast trials), the magnitude of the AWB effect during the temporal-order-judgment task correlated with the magnitude of the congruency effect during the flanker task. In other words, more proactive allocation of attention in the temporal-order-judgment task seems to be associated with worse inhibition in the flanker task. This makes sense, because if older participants do not allocate their attention to the expected location of the distractor (low white bear), then they may never process them in the first place, leading to a lower or eliminated congruency effect because there is nothing to inhibit. An alternative possibility is that this may be attributed to impaired attentional disengagement (Greenwood & Parasuraman, 1994 ; Owsley, 2016 ) in older populations. Older participants who allocated their attention toward the expected distractor locations more often or efficiently may not have been as able to disengage from the distractor, leading to enhanced processing of the flankers and larger congruency effects. This is in line with recent findings from our lab where older participants were less likely to rapidly reject a salient nontarget compared to younger participants (Ashinoff, Geng, & Mevorach, 2019 ). Given that the white-bear effect was reduced in older populations relative to younger populations, it seems more likely that this effect is being driven by reduced distractor processing. Somewhat surprisingly, for the younger participants there was no relationship between the allocation of attention to expected flanker locations during the temporal-order-judgment task and inhibition of those distractors during the flanker task. This has implications for any future interpretations of the AWB effect in healthy, younger controls, as it now seems unlikely that the AWB plays a beneficial, proactive role in inhibition processes. Furthermore, if the allocation of attention in the white-bear paradigm is not a benefit to subsequent inhibition processes, then another possibility is that the age-related reduction in the white bear is a strategic choice, perhaps a result of a rapid disengagement impairment.
It is important to note that the attentional-white-bear paradigm and its eponymous effect has never been defined by inhibition taking place following allocation. In fact, the task design guarantees that allocation cannot be followed directly by inhibition (the temporal-order-judgment task and the flanker task occur on independent trials). In previous studies (Tsal & Makovski, 2006 ), and in ours, it was inferred that attention was being proactively allocated toward the expected distractor location in anticipation of having to subsequently inhibit those locations, but this has always been an assumption and has not been directly tested. Even our result must be interpreted with care since it only indirectly measures this potential relationship and has a small sample size for a correlational analysis.
In summary, older participants do exhibit an age-related difference in performance during the AWB when the attentional system is sufficiently taxed, reflecting differences in the allocation of attention to the location of an expected distractor. Future research will have to establish if this difference reflects an impairment or a strategic choice, since the allocation of attention seems to convey no obvious benefit in this paradigm.
Authors note
The authors would like to thank Denise Clissett for her instrumental role in recruiting older participants, as well as Samantha Ellis, Charlie-Louise Milward, Alice Sarhanis, and Leah Supra for their contributions to this project as undergraduate research assistants. This paper is based on Chapter 5 of author B.K.A’s dissertation (Ashinoff, 2017 ). This research was funded in part by a grant from the ISF (Grant No. 1898/2015) awarded to Yehoshua Tsal. Brandon K. Ashinoff is currently supported by a T32 Postdoctoral Fellowship (T32-MH018870) at Columbia University. Experimental data from this study and program code are publicly available via the Open Science Framework (OSF): https://osf.io/f576c/
Change history
01 november 2019.
This article was originally published with errors in the graphs. It has been republished with corrections.
Allen, H. A., Humphreys, G. W., & Matthews, P. M. (2008). A neural marker of content-specific active ignoring. Journal of Experimental Psychology: Human Perception and Performance , 34 (2), 286–297. doi: https://doi.org/10.1037/0096-1523.34.2.286
Ashinoff, B. K. (2017). The behavioral and neural correlates of cognitive control across the lifespan (Doctoral thesis, University of Birmingham, Birmingham, UK). Retrieved from http://etheses.bham.ac.uk/7738/
Ashinoff, B. K., Geng, J. J., & Mevorach, C. (2019). Delayed reactive distractor suppression in aging populations. Psychology and Aging, 34 (3), 418–430. doi: https://doi.org/10.1037/pag0000336
Article PubMed Google Scholar
Becic, E., Kramer, A. F., & Boot, W. R. (2007). Age-related differences in visual search in dynamic displays. Psychology and Aging , 22 (1), 67–74. doi: https://doi.org/10.1037/0882-7974.22.1.67
Belopolsky, A. V., Peterson, M. S., & Kramer, A. F. (2005). Visual search in temporally segregated displays: Converging operations in the study of the preview benefit. Cognitive Brain Research , 24 (3), 453–466. doi: https://doi.org/10.1016/j.cogbrainres.2005.02.023
Braver, T. S. (2012). The variable nature of cognitive control: A dual mechanisms framework. Trends in Cognitive Sciences , 16 (2), 106–113. doi: https://doi.org/10.1016/j.tics.2011.12.010
Article PubMed PubMed Central Google Scholar
Braver, T. S., Satpute, A. B., Rush, B. K., Racine, C. A., & Barch, D. M. (2005). Context processing and context maintenance in healthy aging and early stage dementia of the Alzheimer’s type. Psychology and Aging , 20 (1), 33–46. doi: https://doi.org/10.1037/0882-7974.20.1.33
Cepeda, N. J., Cave, K. R., Bichot, N. P., & Kim, M.-S. (1998). Spatial selection via feature-driven inhibition of distractor locations. Perception & Psychophysics , 60 (5), 727–746. doi: https://doi.org/10.3758/BF03206059
Article Google Scholar
Craik, F. I. M., & Salthouse, T. A. (2011). The handbook of aging and cognition (3rd ed.). New York, NY: Psychology Press.
Book Google Scholar
Cunningham, C. A., & Egeth, H. E. (2016). Taming the white bear: Initial costs and eventual benefits of distractor inhibition. Psychological Science , 27 (4), 476–485. doi: https://doi.org/10.1177/0956797615626564
Faul, F., Erdfelder, E., Buchner, A., & Lang, A.-G. (2009). Statistical power analyses using G*Power 3.1: Tests for correlation and regression analyses. Behavior Research Methods , 41 (4), 1149–1160. doi: https://doi.org/10.3758/BRM.41.4.1149
Faul, F., Erdfelder, E., Lang, A.-G., & Buchner, A. (2007). G*Power 3: A flexible statistical power analysis program for the social, behavioral, and biomedical sciences. Behavior Research Methods , 39 (2), 175–191. doi: https://doi.org/10.3758/BF03193146
Fernandez-Duque, D., & Black, S. E. (2006). Attentional networks in normal aging and Alzheimer’s disease. Neuropsychology , 20 (2), 133–143. doi: https://doi.org/10.1037/0894-4105.20.2.133
Grady, C. (2012). The cognitive neuroscience of ageing. Nature Reviews Neuroscience , 13 (7), 491–505. doi: https://doi.org/10.1038/nrn3256
Greenwood, P. M., & Parasuraman, R. (1994). Attentional disengagement deficit in nondemented elderly over 75 years of age. Aging, Neuropsychology, and Cognition , 1 (3), 188–202. doi: https://doi.org/10.1080/13825589408256576
Hasher, L., Stoltzfus, E. R., Zacks, R. T., & Rypma, B. (1991). Age and inhibition. Journal of Experimental Psychology: Learning, Memory, and Cognition , 17 (1), 163.
PubMed Google Scholar
Hasher, L., & Zacks, R. T. (1988). Working memory, comprehension, and aging: A review and a new view. In G. H. Bower (Ed.), Psychology of learning and motivation (Vol. 22, pp. 193–225). New York, NY: Academic Press.
Google Scholar
Hedden, T., & Gabrieli, J. D. E. (2004). Insights into the ageing mind: a view from cognitive neuroscience. Nature Reviews Neuroscience , 5 (2), 87–96. doi: https://doi.org/10.1038/nrn1323
Humphreys, G. W., Stalmann, B. J., & Olivers, C. (2004). An analysis of the time course of attention in preview search. Perception & Psychophysics , 66 (5), 713–730.
Jarosz, A. F., & Wiley, J. (2014). What are the odds? A practical guide to computing and reporting Bayes factors. The Journal of Problem Solving , 7 (1). doi: https://doi.org/10.7771/1932-6246.1167
Jimura, K., & Braver, T. S. (2010). Age-related shifts in brain activity dynamics during task switching. Cerebral Cortex , 20 (6), 1420–1431. doi: https://doi.org/10.1093/cercor/bhp206
Jollie, A., Ivanoff, J., Webb, N. E., & Jamieson, A. S. (2016). Expect the unexpected: A paradoxical effect of cue validity on the orienting of attention. Attention, Perception, & Psychophysics , 78 (7), 2124–2134. doi: https://doi.org/10.3758/s13414-016-1164-x
Kawai, N., Kubo-Kawai, N., Kubo, K., Terazawa, T., & Masataka, N. (2012). Distinct aging effects for two types of inhibition in older adults: A near-infrared spectroscopy study on the Simon task and the flanker task. NeuroReport , 23 (14), 819–824. doi: https://doi.org/10.1097/WNR.0b013e3283578032
Kramer, A. F., & Atchley, P. (2000). Age-related effects in the marking of old objects in visual search. Psychology and Aging , 15 (2), 286–296.
Kramer, A. F., Humphrey, D. G., Larish, J. F., & Logan, G. D. (1994). Aging and inhibition: Beyond a unitary view of inhibitory processing in attention. Psychology and Aging , 9 (4), 491–512. doi: https://doi.org/10.1037/0882-7974.9.4.491
Kramer, A. F., & Kray, J. (2006). Aging and attention. In E. Bialystock & F. I. M. Craik (Eds.), Lifespan cognition: Mechanisms of change (pp. 57–69). New York, NY: Oxford University Press. doi: https://doi.org/10.1093/acprof:oso/9780195169539.003.0005
Chapter Google Scholar
Lahav, A., Makovski, T., & Tsal, Y. (2012). White bear everywhere: Exploring the boundaries of the attentional white bear phenomenon. Attention, Perception, & Psychophysics , 74 (4), 661–673. doi: https://doi.org/10.3758/s13414-012-0275-2
Lakens, D. (2013). Calculating and reporting effect sizes to facilitate cumulative science: A practical primer for t -tests and ANOVAs. Frontiers in Psychology , 4 . doi: https://doi.org/10.3389/fpsyg.2013.00863
Lustig, C., Hasher, L., & Zacks, R. T. (2007). Inhibitory deficit theory: Recent developments in a new view. Inhibition in Cognition , 17 , 145–162.
Lustig, C., & Jantz, T. (2015). Questions of age differences in interference control: When and how, not if? Brain Research , 1612 , 59–69. doi: https://doi.org/10.1016/j.brainres.2014.10.024
Marsman, M., & Wagenmakers, E.-J. (2017). Bayesian benefits with JASP. European Journal of Developmental Psychology , 14 (5), 545–555. doi: https://doi.org/10.1080/17405629.2016.1259614
Max, R., & Tsal, Y. (2015). The mutations paradigm: Assessing the time course of distractor processing. Attention, Perception, & Psychophysics , 77 (7), 2344–2355. doi: https://doi.org/10.3758/s13414-015-0928-z
Moher, J., & Egeth, H. E. (2012). The ignoring paradox: Cueing distractor features leads first to selection, then to inhibition of to-be-ignored items. Attention, Perception, & Psychophysics , 74 (8), 1590–1605. doi: https://doi.org/10.3758/s13414-012-0358-0
Munneke, J., Van der Stigchel, S., & Theeuwes, J. (2008). Cueing the location of a distractor: An inhibitory mechanism of spatial attention? Acta Psychologica , 129 (1), 101–107. doi: https://doi.org/10.1016/j.actpsy.2008.05.004
Olivers, C. N. L., Humphreys, G. W., & Braithwaite, J. J. (2006). The preview search task: Evidence for visual marking. Visual Cognition , 14 (4/8), 716–735. doi: https://doi.org/10.1080/13506280500194188
Owsley, C. (2016). Vision and aging. Annual Review of Vision Science , 2 (1), 255–271. doi: https://doi.org/10.1146/annurev-vision-111815-114550
Paxton, J. L., Barch, D. M., Racine, C. A., & Braver, T. S. (2008). Cognitive control, goal maintenance, and prefrontal function in healthy aging. Cerebral Cortex , 18 (5), 1010–1028. doi: https://doi.org/10.1093/cercor/bhm135
Persson, J., Nyberg, L., Lind, J., Larsson, A., Nilsson, L.-G., Ingvar, M., & Buckner, R. L. (2006). Structure–function correlates of cognitive decline in aging. Cerebral Cortex , 16 (7), 907–915. doi: https://doi.org/10.1093/cercor/bhj036
Salthouse, T. A. (2000). Aging and measures of processing speed. Biological Psychology , 54 (1/3), 35–54. doi: https://doi.org/10.1016/S0301-0511(00)00052-1
Salthouse, T. A. (2010). Is flanker-based inhibition related to age? Identifying specific influences of individual differences on neurocognitive variables. Brain and Cognition , 73 (1), 51–61. doi: https://doi.org/10.1016/j.bandc.2010.02.003
Salthouse, T. A., & Meinz, E. J. (1995). Aging, inhibition, working memory, and speed. The Journals of Gerontology Series B: Psychological Sciences and Social Sciences , 50B (6), P297–P306. doi: https://doi.org/10.1093/geronb/50B.6.P297
Tsal, Y., & Makovski, T. (2006). The attentional white bear phenomenon: The mandatory allocation of attention to expected distractor locations. Journal of Experimental Psychology: Human Perception and Performance , 32 (2), 351–363. doi: https://doi.org/10.1037/0096-1523.32.2.351
Verhaeghen, P. (2011). Aging and executive control: Reports of a demise greatly exaggerated. Current Directions in Psychological Science , 20 (3), 174–180. doi: https://doi.org/10.1177/0963721411408772
Verhaeghen, P., & De Meersman, L. (1998). Aging and the Stroop effect: A meta-analysis. Psychology and Aging , 13 (1), 120–126. doi: https://doi.org/10.1037/0882-7974.13.1.120
Watson, D. G., & Humphreys, G. W. (1997). Visual marking: prioritizing selection for new objects by top-down attentional inhibition of old objects. Psychological Review , 104 (1), 90–122.
Watson, D. G., & Maylor, E. A. (2002). Aging and visual marking: Selective deficits for moving stimuli. Psychology and Aging , 17 (2), 321–339. doi: https://doi.org/10.1037//0882-7974.17.2.321
Wagenmakers, E.-J. (2007). A practical solution to the pervasive problems of p values. Psychonomic Bulletin & Review , 14 (5), 779–804. doi: https://doi.org/10.3758/BF03194105
Wei, J., Carroll, R. J., Harden, K. K., & Wu, G. (2012). Comparisons of treatment means when factors do not interact in two-factorial studies. Amino Acids , 42 (5), 2031–2035. doi: https://doi.org/10.1007/s00726-011-0924-0
Wyatt, N., & Machado, L. (2013). Evidence inhibition responds reactively to the salience of distracting information during focused attention. PLOS ONE , 8 (4), e62809. doi: https://doi.org/10.1371/journal.pone.0062809
Zeischka, P., Coomans, D., Deroost, N., Vandenbossche, J., & Soetens, E. (2011). Target–flanker discriminability affects conflict size but not sustained suppression. Acta Psychologica , 136 (1), 148–156. doi: https://doi.org/10.1016/j.actpsy.2010.11.004
Download references
Author information
Authors and affiliations.
School of Psychology, University of Birmingham, Edgbaston, UK
Brandon K. Ashinoff & Carmel Mevorach
Centre for Human Brain Health, University of Birmingham, Edgbaston, UK
Department of Psychiatry, Columbia University, New York, NY, USA
Brandon K. Ashinoff
School of Psychological Sciences, Tel Aviv University, Tel Aviv, Israel
Yehoshua Tsal
Department of Behavioral Sciences, Zefat Academic College, Safed, Israel
You can also search for this author in PubMed Google Scholar
Corresponding author
Correspondence to Brandon K. Ashinoff .
Additional information
Publisher’s note.
Springer Nature remains neutral with regard to jurisdictional claims in published maps and institutional affiliations.
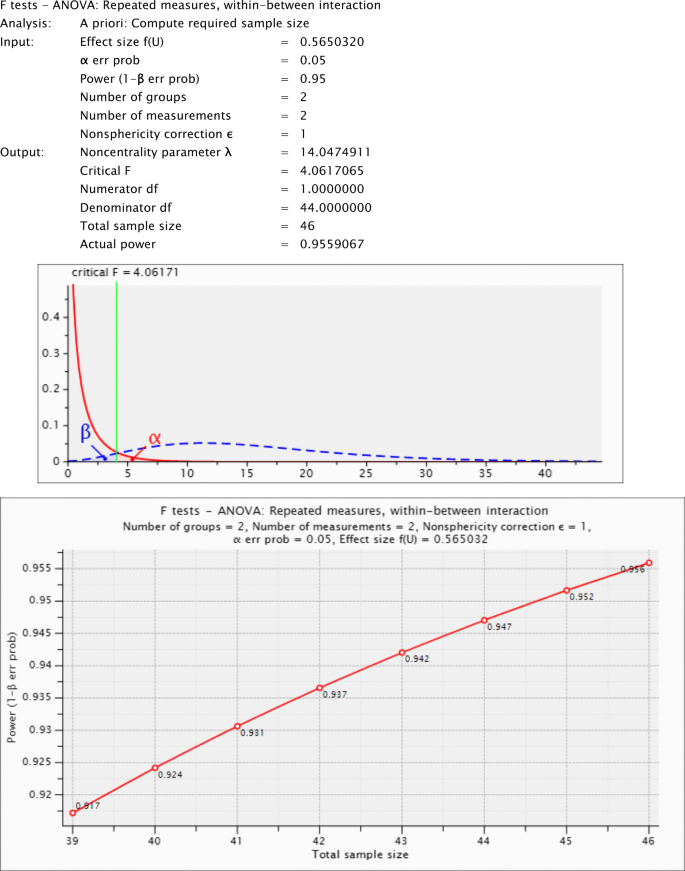
Rights and permissions
Open Access This article is distributed under the terms of the Creative Commons Attribution 4.0 International License (http://creativecommons.org/licenses/by/4.0/), which permits unrestricted use, distribution, and reproduction in any medium, provided you give appropriate credit to the original author(s) and the source, provide a link to the Creative Commons license, and indicate if changes were made.
Reprints and permissions
About this article
Ashinoff, B.K., Tsal, Y. & Mevorach, C. Age-related differences in the attentional white bear. Psychon Bull Rev 26 , 1870–1888 (2019). https://doi.org/10.3758/s13423-019-01622-9
Download citation
Published : 10 June 2019
Issue Date : December 2019
DOI : https://doi.org/10.3758/s13423-019-01622-9
Share this article
Anyone you share the following link with will be able to read this content:
Sorry, a shareable link is not currently available for this article.
Provided by the Springer Nature SharedIt content-sharing initiative
- Aging and attention
- Cognitive aging
- Cognitive and attentional control
- Cognition and aging
- Find a journal
- Publish with us
- Track your research
DNA Pulled From Paw Prints May Help Researchers Study Elusive Polar Bears
As rising temperatures threaten the Arctic mammals, scientists are turning to new, non-invasive methods to study them
/https://tf-cmsv2-smithsonianmag-media.s3.amazonaws.com/accounts/headshot/SarahKuta.png)
Daily Correspondent
:focal(1000x671:1001x672)/https://tf-cmsv2-smithsonianmag-media.s3.amazonaws.com/filer_public/81/de/81dea282-dec0-4c63-93ac-bf1872179d5f/natdiglib_34179_extralarge.jpg)
Polar bears are mostly solitary creatures that can cover huge swaths of rugged, remote terrain in Canada , Norway, Russia, Greenland and Alaska. This makes them difficult to find and study—even as scientists have lots of questions about how they’re faring amid rising global temperatures .
Now, an emerging research method may be able to help. Scientists can identify individual bears by analyzing tiny amounts of DNA they leave behind in footprints as they traipse through the snow, according to two papers published recently in the journal Frontiers in Conservation Science .
This technique could make it cheaper, easier and safer to study polar bears and other similarly elusive creatures , which may ultimately bolster conservation efforts. Polar bears face numerous threats related to human activities, including declining sea ice from human-caused climate change, toxic pollution and habitat loss.
Historically, when scientists have wanted to collect genetic material from polar bears, they’ve had to locate, tranquilize and capture the gigantic mammals, which can weigh up to 1,700 pounds . This invasive method can be dangerous for both the bears and the researchers; in addition, some Indigenous communities oppose the practice over animal welfare concerns or fears of eating meat that’s possibly tainted by sedatives .
So, rather than relying on DNA obtained from the creatures directly, scientists wondered if they could utilize other means of collecting genetic material. They turned their attention toward environmental DNA , also known as eDNA, which animals leave behind as they wander through landscapes. They do so by dropping dead skin cells, shedding bits of fur and depositing feces, for example.
Past studies have successfully used eDNA to study other types of animals, including birds and fish . But this is the first time anyone has been able to link eDNA with an individual animal, reports Science ’s Susan Cosier.
In a study published in Frontiers in Conservation Science this summer, researchers described how they identified six individual polar bears and determined the sex of each—based only on snow gathered from the surface of their tracks. The team investigated a total of 130 polar bear paw prints and was able to successfully isolate DNA from 46 percent of them.
In a second paper , published in the same journal this week, a different group of researchers demonstrated similar capabilities with both wild and captive Alaskan polar bears and Swedish Eurasian lynx, as well as a captive snow leopard. From snow gathered from the animals’ footprints, the team isolated DNA in all the captive samples, 59 percent of the wild lynx samples and 88 percent of the wild polar bear samples. They also linked DNA from the wild paw prints with 12 individual bears.
Researchers decided to home in on eDNA from paw prints, rather than from feces, because DNA degrades as it passes through the animal’s digestive system. Tracks, meanwhile, “usually contain fresh cells, and the DNA is intact because of the cold ‘storage’ temperature,” says ecologist Micaela Hellström , lead author of the study published this week and co-founder of the eDNA research company MIX Research Sweden AB, in a statement .
Moving forward, eDNA likely won’t replace all other polar bear research methods entirely. But scientists may be able to use it in concert with other techniques and technologies , such as tracking collars and other wearable devices, aerial studies using synthetic aperture radar and artificial intelligence models, and “bear-dar” detection systems.
“It’s like putting together a puzzle: You get small pieces of information from different sources,” says Jon Aars , a biologist at the Norwegian Polar Institute who was not involved in the new studies, to Science .
Get the latest stories in your inbox every weekday.
/https://tf-cmsv2-smithsonianmag-media.s3.amazonaws.com/accounts/headshot/SarahKuta.png)
Sarah Kuta | READ MORE
Sarah Kuta is a writer and editor based in Longmont, Colorado. She covers history, science, travel, food and beverage, sustainability, economics and other topics.
- About the WRI
- Mission & Contact Info
- Lynn Rogers, Ph.D.
- Field Station
- Promotional Images
- About the Courses
- Reserve Your Spot
- Testimonials
- Bear Research
- Lily, Hope, Faith & Jason
- Daily Updates
- Yearly Updates
- Make a Donation
- Latest Donors
Published Papers
- Presentations
- Other Information
Welcome! Be sure to visit the NABC website as well.
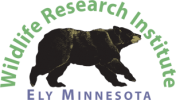
Click highlighted titles to download full texts.
143. Stringham, S. F., Rogers, L. L., Are Brown Bears Less Aggressive in Europe Than in North America? , Autumn 2023 #1, DOI: 10.13140/RG.2.2.32369.45923
142. Roger A. Powell, Susan A. Mansfield, and Lynn L. Rogers. Comparison of behaviors of black bears with and without habituation to humans and supplemental research feeding. Journal of Mammalogy Vol. 103, Issue 6, December 2022, pages 1350-1363
141. Susan A. Mansfield, Lynn L. Rogers, Sean Robison and Roger A. Powell. Bed site selection by female North American black bears (Ursus americanus) Journal of Mammalogy Vol. 103, Issue 2, April 2022, pages 361-372
140. Lynn L. Rogers, Linda McColley, Janet Dalton, Jim Stroner, Douglas Hajicek, Adam Partin and Gordon M. Burghardt. Behavior in Free-Living American Black Bear Dens: Parturition, Maternal Care, and Cub Behavior. Animals 2020, 10, 1123; doi:10,3390/ani10071123
139. Stephen F. Stringham, Lynn L. Rogers, and Ann Bryant (2019). Have Black and Grizzly Bears Become More Dangerous? Insights From Human-Bear Fatality Trends Research Gate, doi: 10.13140/RG.2.2.28390.88648 18 pp.
138. Stringham, Stephen F., Lynn L. Rogers , Ann Bryant (2017). Semantic vs. Empirical Issues in the Bear Diversionary Baiting Controversy. Environment and Ecology Research, 5 , 436 - 442. doi: 10.13189/eer.2017.050604.
137. Stringham, Stephen F. and Lynn L. Rogers. (2017). Fear of Humans by Bears and Other Animals (Anthropophobia): How Much is Natural? Journal of Behavior 2(2): 1009, 16 pages.
136. Rogers, L. L., Mansfield, S. A., Hornby, K., Hornby, S., Debruyn, T. D., Mize, M., Clark, R., Burghardt, G. M. (2014), Black Bear Reactions to Venomous and Non-venomous Snakes in Eastern North America . Ethology, 120: 641–651. doi: 10.1111/eth.12236
135. Rogers, L. L. and S. A. Mansfield. 2013. Contrasts in the languages of wolves and black bears . 2013 International Wolf Symposium. Duluth, Minnesota. October 10-13, 2013. (Abstract)
134. Rogers, L. L. and S. A. Mansfield. 2013. Relations between wolves and black bears . 2013 International Wolf Symposium. Duluth, Minnesota. October 10-13, 2013. (Abstract)
133. Rogers, L. L. and S. A. Mansfield. 2013. Black bear behavior in seven dens in northeastern Minnesota, 2010–2013 . Twenty-second International Conference on Bear Research and Management. Provo, Utah. September 15-20, 2013. (Abstract)
132. Rogers, L. L. and S. A. Mansfield. 2013. Understanding Nervous Black Bear Behavior . Twenty-second International Conference on Bear Research and Management. Provo, Utah. September 15-20, 2013. [Poster]
131. Dykstra, J.A., L. L. Rogers, S. A. Mansfield, and A. Wünschmann. 2012. Fatal disseminated blastomycosis in a free-ranging American black bear ( Ursus americanus ) . Journal of Veterinary Diagnostic Investigation. 24(6) 1125–1128.
130. Rogers, L. L. 2011. Case study: Black bears in Minnesota . [invited talk]. Summary, pp 31-32 In Polar Bear Diversionary Feeding Workshop Report. Karla Dutton, Susanne Miller, and Terry DeBruyn, editors. Anchorage, Alaska. June 8-9, 2011.
129b. Rogers, L. L. and S. A. Mansfield. 2011. Can food lead black bears out of trouble? [invited talk]. 20th International Conference on Bear Research & Management, Ottawa, Ontario. July 17-23, 2011. (Abstract)
129a. Rogers, L. L. and S. A. Mansfield. 2011. Can food lead black bears out of trouble? [invited talk]. 20th International Conference on Bear Research & Management, Ottawa, Ontario. July 17-23, 2011.
128. Rogers, L. L. and S. A. Mansfield. 2011. Behavior of supplementally-fed black bears in Eagles Nest Township, Minnesota . [Poster]. 20th International Conference on Bear Research & Management, Ottawa, Ontario. July 17-23, 2011.
127. Rogers, L. L. 2011. Does diversionary feeding create nuisance bears and jeopardize public safety? Human-Wildlife Interactions. 5(2): 287-295.
126. Rogers, L. L. and S. Mansfield. 2011. Misconceptions about black bears: a response to Geist (2011) . Human-Wildlife Interactions. 5(2): 173–176.
125. Rogers, L. L. 2010. Does diversionary feeding create nuisance bears and jeopardize public safety? Pages 72-82 in C. Lackey and T. A. Beausoleil, editors, Western Black Bear Workshop 10:72-82.
124. Rogers, L. L. 2008. Understanding Black Bears. The Leader. 38(8): 4-6.
123. Peters, G., Owen, M. & Rogers, L. 2007. Humming in bears: a peculiar sustained mammalian vocalization . Acta Theriologica 52: 379-389.
122. Mansfield, S. A. 2007. Effects of supplemental food on weights and reproductive success of black bears in northeastern Minnesota . Unpublished master’s thesis. Antioch University New England, Keene, New Hampshire. 49pp. plus Appendix.
121. Fair, J., and L. Rogers. 2006. Bears for kids, 2nd edition. Wildlife Research Institute. Ely, Minnesota. 48 pages.
120. Rogers, L. L. 2005. Weight-carrying ability and caching behavior of Gray Jays, Perisoreus canadensis: Adaptations to boreal winter . The Canadian Field-Naturalist 119(1):101-104.
119. Mansfield, S. A., and L. L. Rogers. 2005. Behavior, wild diets, and weight gains of supplementally-fed black bears in northeastern Minnesota . [Poster]. 15th Eastern Black Bear Workshop, Tallahassee, FL. Apr 2005.
118. Rogers, L. L. 2000. Bear family group . Fish & Game Finder, vol 23, no. 7, November 2000, pages 9-13. (Slightly edited version of a letter Rogers wrote to Ainslie Willock of the Animal Alliance of Canada on March 18, 1998)
117. Rogers, L. L. 1999. Black bear . Pages 157-160 in Don E. Wilson and Sue Ruff, eds. The Smithsonian Book of North American Mammals. Smithsonian Institution Press. Washington, D.C.
116. Rogers, L. L. 1999. Bear center conducts hibernation study . North American Bear Center Newsletter 3(4):1-3.
115. Rogers, L. L. 1999. The summer of the bear attacks: What's behind the Wisconsin bears in the news? North American Bear Center Newsletter 3(3), Summer, 1999:1-2. Reprinted in Northland Reader 3(57), September 16, 1999: 14.
114. Rogers, Lynn. 1999. Black bear fun facts. Lake Country Journal 3(4)July/August 1999:cover, 8-9.
113. Rogers, L. L. 1999. Spring and the mating season . North American Bear Center Newsletter 3(2), Spring, 1999:1-2.
112. McRoberts, R. E., R. T. Brooks, and L. L. Rogers. 1998. Using nonlinear mixed effects models to estimate size-age relationships for black bears. Canadian J. Zool. 76:1098-1106.
111. Brooks, Robert T., Ronald McRoberts, and Lynn L. Rogers. 1998. Predictive relationships between age and size and front foot pad width of northeastern Minnesota black bears, Ursus americanus . Canadian Field-Naturalist 112(1):82-85.
110. Rogers, Lynn. 1997. The orphaned cubs: a true story. Pages 85-89 In: The end of the road reader (Karin Hokkanen and Anne Stewart, eds). Published by Northwoods Writers Guild, PO Box 749, Ely, MN 55731-0749. Printed by Echo Press, Alexandria, MN.
109. Rogers, L. L. 1997. Nectar-feeding by Cape May, Tennessee, and Nashville Warblers in Minnesota. The Loon 69(2):55-56.
108. Rogers, L. L. 1996. How dangerous are black bears? Can we coexist with them? Take Action 5(1):5. Reprinted in The North Bearing News (North American Bear Center Publication) 1(1):1-2. December 1996. Reprinted in The Botany Bulletin (North Carolina), December 2000.
107. Rogers, L. L. 1995. The black and white of it: black bears and their white pines. Bears Magazine, Fall 1995:9-11, 44.
106. Rogers, L. L. 1995. White pine use by female black bears in northeastern Minnesota . In Abstracts of the Tenth International Conference on Bear Research and Management. Fairbanks, Alaska. July 16-20, 1995.
105. Rogers, L. L. 1994. The waning Minnesota white pine. Inner Voice 6(2):15 (March/April 1994).
104. Rogers, L. L. 1993. Studying habituated black bears . Pages 210-211 In Ian Stirling, ed. Bears: majestic creatures of the wild. Rodale Press. Emmaus, Pennsylvania.
103. Rogers, L. L. 1993. Bear navigation . Page 78 In Ian Stirling, ed. Bears: majestic creatures of the wild. Rodale Press. Emmaus, Pennsylvania.
102. Hellgren, E. C., L. L. Rogers, and U. S. Seal. 1993. Serum chemistry and hematology of black bears: physiological indices of habitat quality or seasonal patterns? J. Mamm. 74(2):304-315.
101. Rogers, L. L. 1993. The role of habitat quality in the natural regulation of black bear populations . Proc. 4th Western Black Bear Workshop: 95-102. Yosemite National Park, California. Technical Report NPS/NRWR/NRTR-93/12.
100. Rogers, L. L., and Edward L. Lindquist. 1992. Supercanopy white pine and wildlife . Pages 39-43 in Robert A. Stine and Melvin J. Baughman (eds.) White Pine Symposium Proceedings NR-BU-6044. Symposium held September 16-18, 1992, at the Duluth Entertainment Convention Center. Available from Minnesota Extension Service, Distribution Center, Room 3, Coffey Hall, 1420 Eckles Avenue, University of Minnesota, St Paul, MN 55108-6064 (612) 625-8173 or FAX (612) 625-2207.
99. Rogers, L. L., P. A. Beringer, D. A. Trauba, and G. A. Wilker. 1992. Fawn predation by black bears in northeastern Minnesota . Ninth International Conference on Bear Research and Management. Missoula, Montana. February 24-28, 1992. (Abstract)
98. Baldridge, A., and L. L. Rogers. 1992. Harbor seal predation on a wolf-eel . California Fish and Game J. 77(4)Fall 1991:210-211.
97. Rogers, L. L. 1992. Watchable wildlife: the black bear. USDA, Forest Service, North Central Forest Experiment Station. 15p.
96. Rogers, L. L., and R. McRoberts. 1992. Estimation of shrub leaf biomass available to white-tailed deer. Gen. Tech. Rep. NC-307. 16p.
95. Yarmark, D., and L. L. Rogers. 1991. The three bears of Kawishiwi River: researcher learns lots about Minnesota black bears, GBF helps . Bear News vol 9(1)Spring 1991. Great Bear Foundation.
94. Rogers, L. L. 1991. Foster families found for deserted cubs. Pages 98-102 in John Henricksson (ed) North Writers: a strong woods collection. University of Minnesota Press. Minneapolis. 292 pp. (Reprinted from Minnesota Volunteer magazine, July-August 1989)
93. Johnson, L. B., G. E. Host, J. K. Jordan, and L. L. Rogers. 1991. Pages 507-517 in Use of GIS for landscape design in natural resource management: habitat assessment and management for the female black bear. Proc. GIS/LIS '91 Conf. Atlanta, Georgia. October 28-November 1, 1991.
92. Host, G. E., L. B. Johnson, J. Jordan, and L. L. Rogers. 1991. Geographic information systems for spatial analysis and landscape design in natural resource management . Page 34 in Abstracts of papers: Emerging issues in northern hardwood management: air pollution, climate change, and biodiversity. May 21-23, 1991. Mackinac Island, Michigan. (Abstract)
91. DelGiudice, G. D., L. L. Rogers, A. W. Allen, and U. S. Seal. 1991. Weights and hematology of wild black bears during hibernation . J. Wildl. Dis. 27(4):637-642.
90. Rogers, L. L., G. A. Wilker, and S. S. Scott. 1991. Reactions of black bears to human menstrual odors . J. Wildl. Manage. 55(4):632-634.
89. Rogers, L. L. 1991. Bears and other carnivores . Pages 184-193 in R. Robin Baker, ed. Fantastic journeys: the marvels of animal migration. Weldon Owen Publishing, Sidney, Australia.
88. Rogers, L. L. 1991. Are white pines too valuable to cut? The Minnesota Volunteer: Department of Natural Resources. Sept-Oct. 1991:8-21.
87. Rogers, L. L., and E. L. Lindquist. 1991. Black bears and the oak resource in northeastern Minnesota . Pages 107-114 in S. B. Laursen and J. F. DeBoe (eds.), The oak resource in the upper midwest--implications for management. Minnesota Extension Serv. Publ. NR-BU-5663-S, Univ. Minnesota, St. Paul.
86. Rogers, L. L. 1991. The black bear in a changing environment . Page 7 in Views of a changing planet: a strategy for the 1990s. March 9, 1991. Harvard University. Cambridge, Massachusetts. (Abstract)
85. Rogers, L. L. 1990. Preface. Pages 6-7 in Jeff Fair (author). The Great American Bear . NorthWord Press, Inc. Minocqua, Wisconsin. 192pp.
84. Rogers, L. L., P. S. Beringer, R. E. Kennedy, and G. A. Wilker. 1990. Fawn predation by black bears . Page 261 in Abstracts: 52nd Midwest Fish and Wildlife Conf. December 2-5, 1990. Minneapolis, Minnesota. 406 pp. (Abstract)
83. Rogers, L. L., and G. W. Wilker. 1990. How to obtain behavioral and ecological information from free-ranging, researcher-habituated black bears . Bear Res. and Manage. 8:321-328.
82. Rogers, L. L. 1990. Bear fact: black bear not as harmful as it looks or acts . Outdoor News 23(31):8. August 3, 1990.
81. Rogers, L. L. 1990. Black bears are known to roam--but their den is always home. Outdoor News 23(31):7. August 3, 1990.
80. Rogers, L. L., G. A. Wilker, and S. S. Scott. 1990. Managing natural populations of black bears in wilderness . Pages 363-366 In D. W. Lime (ed). Managing America's enduring wilderness resource. University of Minnesota Press, Minneapolis. 706pp.
79. Ream, R. R., L. L. Rogers, and J. M. Peek. 1990. Managing natural populations of wildlife in wilderness. Pages 357-362 In D. W. Lime (ed). Managing America's enduring wilderness resource. University of Minnesota Press, Minneapolis. 706pp.
78. Rogers, L. L., and G. A. Wilker. 1989. Habitat use, diet, activity patterns, and communication methods of wild, researcher-habituated black bears (abstract). Pages 111-112 in Abstracts of the 51st Midwest Fish and Wildlife Conf. December 3-6, 1989. Springfield, Illinois. 264 pp.
77 1/2. Rogers, L. L., 1989. 1989 Great Lakes Bear Habitat Study , 1989 progress report. 56 pages. USDA, Forest Service, progress report to forest supervisors, Lakes States Forests, December 30, 1989.
77. Rogers, L. L. 1989. A year in the life of a black bear in northern Minnesota . Page 1 In 1990 Minnesota Weatherguide Calendar. Publ. by Science Museum of Minnesota, Freshwater Foundation, and WCCO Weather Center. Mpls., Minn.
76. Rogers, L. L. 1989. Home, sweet-smelling home . Natural History 9/89:60-67, 96.
75. Rogers, L. L., G. A. Wilker, and S. S. Scott. 1989. What is important to black bears in the Lakes States? Proc. Aspen Symp. '89. July 25-27, 1989. Duluth, Minnesota. (Abstract)
74. Rogers, L. L., G. A. Wilker, and V. D. O'Connor. 1989. Continuous observation reveals habitat use, diet, activity patterns, and communication methods of black bears in northeastern Minnesota . Eighth International Conference on Bear Research and Management. Victoria, British Columbia, Canada. February 20-25, 1989. (Abstract)
73. Rogers, L. L. 1989. Foster families found for deserted cubs . The Minnesota Volunteer 52(305):26-31. Reprinted in: L. L. Rogers. 1991. Foster families found for deserted cubs. Pages 98-102 In John Henricksson (ed.) North Writers: A Strong Woods Collection. University of Minnesota Press, Minneapolis. 292p.
72. Rogers, L. L. 1989. Black bears, people, and garbage dumps in Minnesota . Pages 48-51 In: M. Bromley and P. A. Gray, eds. Proc. Symp. Bear-people conflicts. Yellowknife, N.W.T. Canada.
71. Rogers, L. L., and S. S. Scott. 1989. Bear diet and habitat in the Great Lakes Region, 1988 progress report . 15 pages. USDA, Forest Service, progress report to forest supervisors, Lakes States Forests, January 1, 1989.
70. Rogers, L. L., D. L. Garshelis, and John R. Chell. 1988. Hunt for the rogue bear . Minnesota Volunteer 51(299):57-62.
69. Rogers, L. L., and D. L. Garshelis. 1988. The BWCA bear attacks of 1987 . Boundary Waters Journal 2(1):48-50, 56.
68. Rogers, L. L., G. A. Wilker, and A. W. Allen. 1988. Managing northern forests for black bears . Pages 140-146 In: Economic and social development: a role for forests and forestry professionals. Proc. of the Society of American Foresters National Convention. October 18-21, 1987. Minneapolis, Minnesota. Reprinted as L. L. Rogers, G. A. Wilker, and A. W. Allen. 1988. Managing northern forests for black bears. Pages 36-42 In: T. W. Hoekstra and J. Capp, Compilers. Integrating forest management for wildlife and fish. U.S. Forest Service General Technical Report NC-122.
67. Maxwell, R., J. Thorkelson, L. L. Rogers, and R. B. Brander. 1988. The field energetics of winter-dormant black bear (Ursus americanus) in northeastern Minnesota . Can. J. Zool. 66:2095-2103.
66. Rogers, L. L. 1988. Homing tendencies of large mammals: a review . Pages 76-92 In: L. Nielsen and R. Brown, eds. Translocation of wild animals. Wisconsin Humane Society, Milwaukee. 335 pp.
65. Rogers, L. L. 1988. How to live with black bears . U.S. For. Serv., N. Central Forest Exp. Station. HT-66. Updated and renamed "How to camp in bear country" on 28-Sept-01.
64. Rogers, L. L., and G. A. Wilker. 1987. Researcher-conditioned black bears provide detailed habitat use data (abstract) . Pages 177-178 in Abstracts of the 49th Midwest Fish and Wildlife Conf. December 5-9, 1987. Milwaukee, Wisconsin. 282 pp. (Abstract)
63. Chepko-Sade, B. D., W. H. Shields, J. Berger, Z. T. Halpin, W. T. Jones, L. L. Rogers, J. Rood, and A. Smith. 1987. The effects of dispersal and social structure on effective population size. Pages 287-321 In: B. D. Chepko-Sade and Z. T. Halpin, eds. Mammalian dispersal patterns: the effects of social structure on population genetics. University of Chicago Press. 342 pp. (Peer reviewed)
62. Rogers, L. L. 1987. Factors influencing dispersal in black bears . Pages 75-84 In: B. D. Chepko-Sade and Z. T. Halpin, eds. Mammalian dispersal patterns: the effects of social structure on population genetics. University of Chicago Press. 342 pp. (Peer reviewed)
61. Rogers, L. L., and Arthur W. Allen. 1987. Habitat suitability index models: black bear, Upper Great Lakes Region . National Ecology Center, U.S. Fish Wildl. Service, Biol. Rep. 82(10.144). 54 pp. (Peer reviewed)
60. Rogers, L. L. 1987. Boundary waters bears . BWCA Wilderness News (Spring-Summer):10-11.
59. Rogers, L. L. 1987. Spring beckons mother and cubs from birth den. Minnesota Volunteer 50(291):32-35.
58. Rogers, L. L. 1987. Habits of black bears: Bears and people in the Superior National Forest. f Boundary Waters Journal 1(1):44-47.
57. Rogers, L. L., and S. C. Durst. 1987. Evidence that black bears reduce peripheral blood flow during hibernation . J. Mammal. 68(4):872-875.
56. Rogers, L. L. 1987. Portrait of a black bear . Encounters 10(2):10-13.
55. Rogers, L. L. 1987. Effects of food supply and kinship on social behavior, movements, and population dynamics of black bears in northeastern Minnesota . Wildlife Monograph 97. 72 pp.
54. Rogers, L. L., A. N. Moen, and M. L. Shedd. 1987. Rectal temperatures of 2 free-ranging white-tailed deer fawns. J. Wildl. Manage. 51(1):59-62.
53. Rogers, L. L. 1987. Seasonal changes in defecation rates of free-ranging white-tailed deer. J. Wildl. Manage.51(2):328-331.
52. Rogers, L. L. 1987. Navigation by adult black bears . J. Mammal. 68(1):185-188.
51. Rogers, L. L., and A. Taylor. 1986. Why black bears are masters of winter survival . (Woods Wise column published in Minnesota Out of Doors, Woodland Management, Minnesota Forests, National Woodlands, Illinois Wildlife, Pennsylvania Forests, Missouri Wildlife, Michigan Out of Doors, Hoosier Conservation, Forestry Forum, and Northbound Magazine)
50. Rogers, L. L. 1986. Habits of black bears in Minnesota . Minnesota Forests 1986 (Fall):8-9.
49. Ensrud, E. R., R. A. Nelson, G. Alt, T. Beck, G. Matula, and L. Rogers. 1986. Seasonal variation in the ratio of serum urea to creatinine (U/C) in black bears . The Physiologist 29(4):116 (Abstract).
48. Rogers, L. L. 1986. Homing by black bears in Minnesota . Canadian Field-Naturalist 100(3):350-353.
47. Rogers, L. L. 1986. Long-term survival of adopted black bear cubs in suboptimal habitat . Wildl. Soc. Bull. 14(1):81-83.
46. Rogers, L. L. 1986. Effects of translocation distance on frequency of returns by black bears . Wildl. Soc. Bull. 14(1):76-80.
45. Rogers, L. L. 1985. Aiding the wild survival of orphaned bear cubs . Wildlife Rehabilitation 4:104-111.
44. Moen, A. N., and L. L. Rogers. 1985. Radiant surface temperatures and hair depths of a black bear, Ursus americanus . Canadian Field-Naturalist 99(1):47-50.
43. Rogers, L. L. 1984. Black bear homing panel summary . Proc. East. Workshop Black Bear Manage. Res. 7:78.
42. Rogers, L. L. 1984. Homing by black bears and other large mammals . Proc. East. Workshop Black Bear Manage. Res. 7:76-77.
41. Rogers, L. L. 1984. Recent advances in studies of homing mechanisms . Proc. East. Workshop Black Bear Manage. Res.7:75-76.
40. Rogers, L. L. 1984. Reactions of free-ranging black bears to capsaicin spray repellent . Wildl. Soc. Bull. 12(1):59-61.
39. Rogers, L. L. 1983. Effects of food supply, predation, cannibalism, parasites, and other health problems on black bear populations . pp. 194-211. In: F. Bunnell, D. S. Eastman, and J. M. Peek (eds.). Symposium in Natural Regulation of Wildlife Populations. Forest, Wildlife, and Range Experiment Station Proceedings 14. Univ. of Idaho, Moscow. 225 pp. (Peer reviewed)
38. Rogers, L. L., and R. Applegate. 1983. Dispersal of fruit seeds by black bears . J. Mamm. 64(2):310-311.
37. Wolfe, R. R., R. A. Nelson, M. H. Wolfe, and L. L. Rogers. 1982. Nitrogen cycling in hibernating bears. Proc. Amer. Soc. Mass Spectrometry 30:426. (Abstract)
36. Wolfe, R. R., R. A. Nelson, T. P. Stein, L. L. Rogers, and M. H. Wolfe. 1982. Urea nitrogen reutilization in hibernating bears. Federation Proceedings 41:1623. (Abstract)
35. Rogers, L. L. 1982. Deer, wolf decline prompts intensive deer study in northeastern Minnesota . Restoration and Management Notes 1(2):22. (Abstract)
34. Rogers, L. L. 1981. A bear in its lair . Natural History 90(10):64-70. Translated and reprinted as: L. L. Rogers. 1981. Die Gefahr Vershlafen (Sleeping through hard times). Weltwoche 9(52):34. Also translated and reprinted as: L. L. Rogers. 1984. Sieben monate auf der Barenhaut (Seven months of laziness). Das Tier 1/84:4-9.
33. Rogers, L. L., J. J. Mooty, and D. K. Dawson. 1981. Foods of white-tailed deer in the Upper Great Lakes Region: A review. U.S. Dep. Agric., For. Serv., GTR NC-65. 24 pp. (Peer reviewed)
32. Hamer, D., S. Herrero, and L. L. Rogers. 1981. Differentiating black and grizzly bear feces. Wildl. Soc. Bull. 9(3):210-212.
31. Rogers, L. L. 1981. Walking with deer . pp. 29-34. In: W. H. Nesbitt and P. L. Wright (eds.). Records of North American Big Game. Boone and Crockett Club, Publisher. Alexandria, VA.
30. Ohmann, L. F., D. F. Grigal, and L. L. Rogers. 1981. Estimating plant biomass for undergrowth species of northeastern Minnesota . U.S. Dep. Agric., For. Serv., GTR NC-61. 10 pp. (Peer reviewed)
29. Rogers, L. L., and L. D. Mech. 1981. Interactions of wolves and black bears in northeastern Minnesota . J. Mamm. 62(2):434-436.
28. Buech, R. R., L. L. Rogers, and L. D. Mech. 1980. People and wildlife--bringing them together. Naturalist 31(4):12-20.
27. Rogers, L. L., L. D. Mech, D. K. Dawson, M. Korb, and J. Peek. 1980. Deer distribution in relation to wolf pack territory edges . J. Wildl. Manage. 44(1):253-258.
26. Kramer, G., and L. L. Rogers. 1980. Bear-ly visible. National Geographic World Magazine No. 64 (December 1980):4-9.
25. Folk, G. E., P. S. Cooper, L. L. Rogers, C. V. Gisolfi, and V. J. Morinello. 1980. Studies on hibernation-inducing factor. Report No. 22 from the Laboratory of Environmental Physiology, The University of Iowa, Iowa City, Iowa. 30 pp.
24. Barnes, D., and L. L. Rogers. 1980. Clostridial myonecrosis associated with drug administration. J. Wildl. Diseases 16(3):315-317.
23. Rogers, L. L. 1980. Inheritance of coat color and changes in pelage coloration in black bears in northeastern Minnesota . J. Mamm. 61(2):324-327.
22. Rogers, L. L. 1980. The use of tame, free-ranging deer in studies of food habits . Abstracts of papers presented at the 42nd Midwest Fish and Wildlife Conference, St. Paul, Minnesota. Dec. 7-10, 1980. (Abstract)
21. Buech, R. R., L. L. Rogers, and L. D. Mech. 1980. People and wildlife--bringing them together. Naturalist 31(4):12-20.
20. Rogers, L. L. 1979. Interpretation of cementum annuli in first premolars of bear. Proc. 4th Eastern Black Bear Workshop: 102-112.
19. Rogers, L. L. 1979. Shorter bear season needed to prevent overkill . The Minnesota Arrow 7(2):37-39. April 1979.
18. Applegate, R., L. L. Rogers, D. Casteel, and J. Novack. 1979. Germination of cow parsnip seeds from grizzly bear feces . J. Mamm. 60:655.
17. Rogers, Lynn Leroy. 1978. Social relationships, movements, and population dynamics of black bears in northeastern Minnesota . Dissertation Abstracts International, Volume XXXIX, Number 1, 1978. 2p
16. Rogers, L. L. 1977. The ubiquitous American black bear . pp. 28-33. In: Wm. H. Nesbitt and S. S. Parker (eds.) North American Big Game. Boone and Crockett Club and National Rifle association of America. Washington, D.C. 367 pp. Reprinted in The Minnesota Arrow 6(5):28-32, October 1978.
15. Rogers, L. L. 1977. Fourth International Conference on Bear Research and Management, a review . Wildl. Soc. Bull. 5(4):196:197.
14. Mech, L. D., and L. L. Rogers. 1977. Status, distribution, and movements of martens in northeastern Minnesota . USDA Forest Service Research Paper NC-143. 7 pp.
13. Rogers, L. L. 1976. Effects of mast and berry crop failures on survival, growth, and reproductive success of black bears . Trans. North Amer. Wildl. and Natural Resour. Conf. 41:431-438.
12. McMillin, J. M., U. S. Seal, L. L. Rogers, and A. W. Erickson. 1976. Annual testosterone rhythm in the black bear (Ursus americanus) . Biology of Reproduction 15:163-167.
11. Rogers, L. L., D. W. Kuehn, A. W. Erickson, E. M. Harger, L. J. Verme, and J. J. Ozoga. 1976. Characteristics and management of black bears that feed in garbage dumps, campgrounds, or residential areas . Bear Research and Management 3:169-175.
10. Rogers, L. L., C. M. Stowe, and A. W. Erickson, 1976. Succinylcholine chloride immobilization of black bear. Bear Research and Manage. 3:431-436.
9. Rogers, L. L., and S. M. Rogers. 1976. Parasites of bears: a review . Bear Research and Manage. 3:411-430.
8. Rogers, L. L. 1975. The use of dental annuli as an index to reproductive success . p. 62 In: Abstracts of technical papers presented at the 55th annual meeting of the American Society of Mammalogists. Univ. Montana, Missoula. 121 pp. (Abstract)
7. Rogers, L. L. 1975. Black bears of the Lake Superior Region . North Land 3(1):1-6.
6. Rogers, L. L. 1975. Parasites of black bears ( Ursus americanus Pallas ) of the Lake Superior Region . J. Wildl. Diseases 11:189-192.
5. Rogers, L. L. 1974. Trail of a big black bear. National Rifle Assoc. Hunting Annual. 1974:20-21, 167-169.
4. Rogers, L. L. 1974. Shedding of foot pads by black bears during denning . J. Mamm. 55(3):672-674.
3. Rogers, L. L. 1972. Movement patterns and social organization of black bears in northeastern Minnesota. Bull. Ecol. Soc. Amer. 53(2)21. (Abstract)
2. Maxwell, R., J. Thorkelson, and L. L. Rogers. 1972. The energetics of wintering bears (Ursus americanus) in northeastern Minnesota. Bull. Ecol. Soc. Amer. 53(2):21. (Abstract)
1. Rogers, L. L. 1970. Black bear of Minnesota . Naturalist 21(4):42-47.
Thank you for visiting nature.com. You are using a browser version with limited support for CSS. To obtain the best experience, we recommend you use a more up to date browser (or turn off compatibility mode in Internet Explorer). In the meantime, to ensure continued support, we are displaying the site without styles and JavaScript.
- View all journals
- My Account Login
- Explore content
- About the journal
- Publish with us
- Sign up for alerts
- Open access
- Published: 28 October 2021
The giant panda is cryptic
- Ossi Nokelainen 1 ,
- Nicholas E. Scott-Samuel 2 ,
- Yonggang Nie 3 , 4 ,
- Fuwen Wei 3 , 4 &
- Tim Caro 5 , 6
Scientific Reports volume 11 , Article number: 21287 ( 2021 ) Cite this article
18k Accesses
11 Citations
624 Altmetric
Metrics details
- Behavioural ecology
The giant panda ( Ailuropoda melanoleuca ) is an iconic mammal, but the function of its black-and-white coloration is mysterious. Using photographs of giant pandas taken in the wild and state-of-the-art image analysis, we confirm the counterintuitive hypothesis that their coloration provides camouflage in their natural environment. The black fur blends into dark shades and tree trunks, whereas white fur matches foliage and snow when present, and intermediate pelage tones match rocks and ground. At longer viewing distances giant pandas show high edge disruption that breaks up their outline, and up close they rely more on background matching. The results are consistent across acuity-corrected canine, feline, and human vision models. We also show quantitatively that the species animal-to-background colour matching falls within the range of other species that are widely recognised as cryptic. Thus, their coloration is an adaptation to provide background matching in the visual environment in which they live and simultaneously to afford distance-dependent disruptive coloration, the latter of which constitutes the first computational evidence of this form of protective coloration in mammals.
Similar content being viewed by others
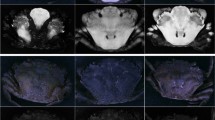
Background matching and disruptive coloration as habitat-specific strategies for camouflage
Natasha Price, Samuel Green, … Martin Stevens
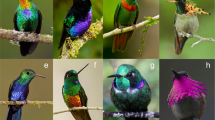
Hummingbird plumage color diversity exceeds the known gamut of all other birds
Gabriela X. Venable, Kaija Gahm & Richard O. Prum
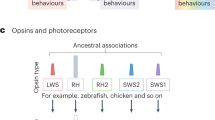
Ancestral photoreceptor diversity as the basis of visual behaviour
Introduction.
Most mammals have drab coloration, generally showing brown tones, but there are a small number of well-known exceptions that demand evolutionary explanation 1 , 2 . One is the giant panda ( Ailuropoda melanoleuca ), an iconic flagship species of conservation biology which is familiar to a great many people, but the function of its black-and-white coloration has proven puzzling. Several hypotheses for its strange appearance have been suggested. These include intraspecific signalling 3 , 4 , heat management 3 , aposematism 5 , 6 , and background matching 5 , 7 , the latter two to avoid predation by tigers ( Panthera tigris ), leopards ( Panthera pardus ) and dholes ( Cuon alpinus ), all of which are suggested to prey on giant pandas, especially young ones 3 , 4 . To date however, the adaptive significance of its seemingly conspicuous black-and-white coloration remains unresolved.
With respect to camouflage 8 , coloration may facilitate crypsis (i.e., preventing detection), for example through both background matching 9 , whereby an animal’s appearance matches the colour, lightness and pattern of its visual background, and disruptive coloration, whereby an animal’s appearance creates false edges or boundaries hindering recognition of its shape 10 . Caro and colleagues 11 used a comparative phylogenetic analysis of carnivores to propose that the giant panda has cryptic body pelage (while the black markings around the eyes and on the ears could be used in communication). Using comparative methods to score coloration, they showed that lighter fur colour correlates with the presence of snow across terrestrial carnivore species, making it plausible that white areas of the giant panda’s body are cryptic against the seasonal snowy background characteristic of the species’ habitat 11 . In contrast, dark areas are associated with shade in carnivores and ursids, suggesting these parts of the giant panda’s pelage might keep the animal camouflaged in shaded forested areas 12 . This dual function coloration could be driven by the need of this species to traverse two very different visual backgrounds (winter and summer habitat) during the course of the year, because their diet prevents them from hibernating as do some other Ursidae 11 . While background matching was implicated in these analyses 11 , disruptive coloration 13 , 14 was poorly supported. However, the pelage displays two critical features of disruptive coloration: highly contrasting colour patches intersecting the animal’s outline, and coloration that matches at least some visual elements of the background—maximum disruptive contrast and differential blending, respectively 8 , 15 , 16 .
We explore the hypothesis that the giant panda is cryptic in its natural mountain forest habitat, despite the fact that the species’ black-and-white coloration appears conspicuous when viewed close up (Fig. 1 ). To achieve this, we examined difficult-to-obtain photographs of 15 giant pandas taken in the wild using the Multispectral Image Calibration and Analysis (MICA) toolbox 17 and the Quantitative Colour and Pattern Analysis (QCPA) framework 18 , which together provide state-of-the-art image analysis 17 , 18 , 19 , 20 , to establish whether or not giant pandas are camouflaged either through background matching or disruptive coloration, or both. We note that the sample size is not large, but fifteen replicates are adequate to address some of the basic questions related to their mysterious appearance from a visual ecology perspective.
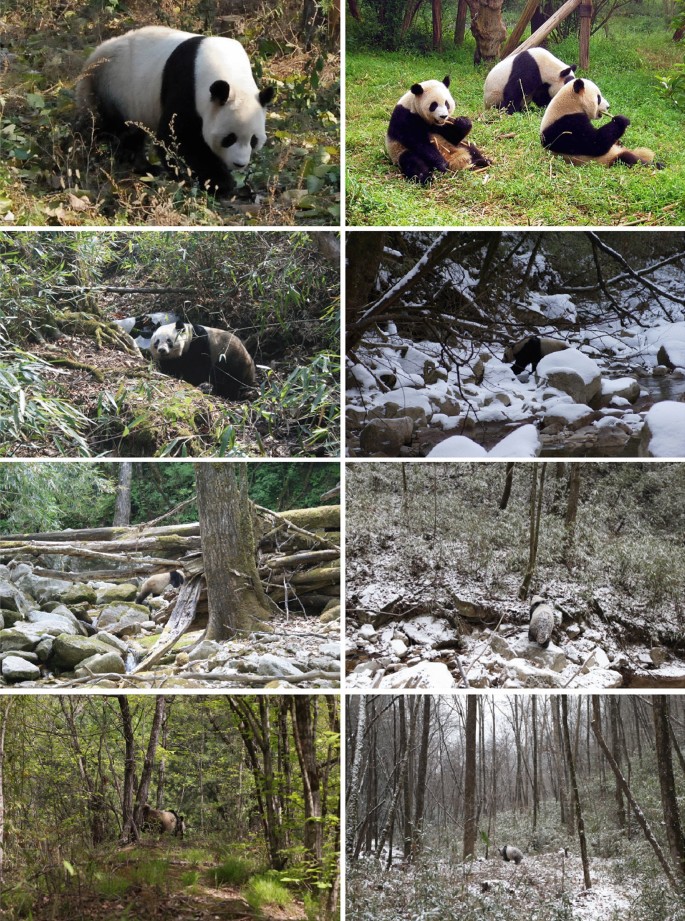
Giant panda coloration and its visual background. First row: animals photographed in a zoo (left Beijing zoo, right Chengdu zoo, Wikimedia commons, the rest in nature by FW), exemplifying how black-and-white coloration stands out when viewed close up and/or against an artificial background. Second row: In its natural habitat, the coloration is less conspicuous even at close range. Third row: the camouflage is more evident at intermediate distances and from various angles, and helps the giant panda to blend into its natural environment. Bottom row: from further away, the black-and-white coloration breaks up the outline of the animal due to increased edge disruption. Note that these figures are meant illustrative—the images are not normalised data.
First, using the QCPA framework and its acuity-corrected cluster analysis (RNL clustering), we studied how closely the black-and-white pelage of the giant panda clusters with the visual patchiness of the background. This approach uses spatial filtering to determine which colour and/or luminance elements cluster together in the image (Fig. 2 ). We assumed that the pelage would have counterparts (i.e., similar brightness elements) present in the visual background if they were to serve a background matching function. We modelled the image data with dichromatic (canine and felid—predator surrogates) and trichromatic (human—a point of reference) vision systems and their corresponding visual acuities. Second, using Gabor filtering to quantify the ratio of false edges that run orthogonal to an animal’s true outline 19 , we probed whether the giant panda demonstrates disruptive coloration operating as a function of distance. We hypothesised that edge disruption, if it occurs, would operate more easily on animals further away because, as the distance between predator and prey increases, the latter’s outline will become less visible than the highly salient disruptive markings 15 , 21 , 22 . At the limit of object detection (e.g., at the maximum distance at which any part of the panda can still be seen by a potential predator), both disruptive markings and the animal’s outline should become undetectable. Finally, to debunk the myth of conspicuousness of giant pandas in their natural environment, a common misconception, we asked whether giant panda coloration matches the background colour variation as assessed by a colour map comparison technique (a component of QCPA) using human vision as a proxy 18 . We compared this similarity-to-background metric in the giant panda to a variety of other species examined objectively along the “crypsis-conspicuousness spectrum”. Our results provide information on how conspicuous black-and-white coloration in animals may provide concealment in the visual environment in which they live and simultaneously afford distance-dependent disruptive coloration.
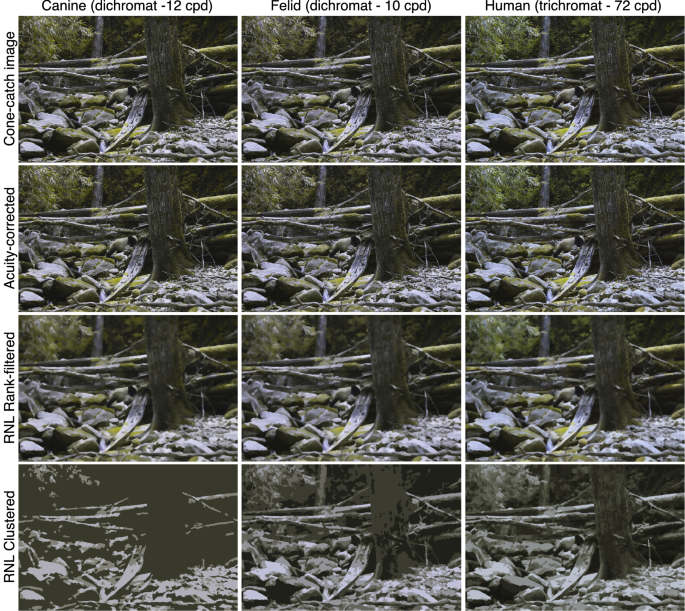
Quantitative colour and pattern analysis (QCPA) was used to investigate the giant panda coloration in its natural habitat. We studied how closely the black-and-white pelages of the giant panda clusters with the visual patchiness of the background. The RNL clustering method uses spatial filtering to determine which visual elements group together in the image. Here, one example of the giant panda images is shown and modelled using dichromatic canine (domestic dog) and felid (cat) vision, and trichromatic human vision. The first row shows images converted to receiver dependent cone catch images, the second row shows acuity-corrected (canine—dog 12 cpd, felid—cat 10 cpd, human 72 cpd,) images, the third row shows RNL rank-filtered images and the fourth row shows RNL-clustered view. These examples were gamma corrected for better image screening.
Quantification of background matching: how does the patchiness of the black-and-white coloration blend into the visual environment?
The giant panda pelage patches were significantly associated with visual elements found in their natural habitat (Chi-Square test for independence, χ 2 = 89.01, df = 10, p < 0.001). There were no patches that were unique to the giant panda and not found in the background in terms of luminance. The black patches clustered with shadows and naturally dark tree trunks, white patches clustered with foliage (due to specular light reflecting from the leaves under high irradiance) and snow when present, and midtones clustered with ground and rocks. Although people tend to consider the giant panda as dichotomously black-and-white, we detected an additional visual cluster of off-white fur, which characterizes midtones in terms of luminance (i.e., not being dark or light).
More specifically, background visual elements (i.e., shade, trees, ground, snow, foliage, rocks) clustered between the black, midtones and white colours of giant pandas’ pelage as follows (Fig. 3 ). On average across the three visual models, the black patches were associated with dark tree trunks (57.6%) and shadows (30.7%), but also with ground (3.8%), rocks (3.8%) and foliage (3.8%). The white patches were grouped with snow (on average 60.0% of the images, but always when snow was present), waxy bright foliage (30.0%) and rocks (10.0%). The midtones were clustered with ground (35.8%), foliage (28.2%), shady background areas (17.9%), and also with rocks (17.9%).
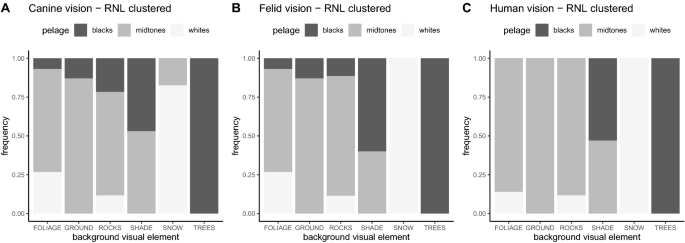
Background matching of the giant panda. The clustering method uses spatial filtering to test which visual elements cluster together in the image. The vision modelling was performed using acuity-corrected cone-catch images. We used three vision models: ( A ) canine vision (dog 12 cpd) and ( B ) felid vision (cat 10 cpd), as the dichromatic predator surrogates, and ( C ) the trichromatic human vision (72 cpd) for the sake of comparison and to provide a point of reference. The clustering results show that the dark elements (e.g., shadows, tree trunks) in the background are grouped with black pelage patches, brightest elements (e.g., snow, bright leaves in foliage and rocks) are grouped together with white pelage patches, whereas elements with midtones (e.g., ground, but also rocks, leaves and shades) are grouped with intermediate off-white pelage patches. The frequency of each subgroup is represented allowing a comparison of the contribution of their proportion to the whole. The frequencies (0–1) are additive to 100% within the visual background comparison group.
QCPA showed similar clustering results of background matching for ROI comparisons (i.e., regions of interest: the panda, the background, the entire image) as regards number of visual clusters formed, cluster luminance and relative patch coverage (Fig. 4 , Tables S3 – S7 ). Also, the majority of Visual Contrast Analysis (VCA) metrics overlapped when comparing values across the giant panda, the background and the entire image (Fig. S3 ). However, two variables from dichromatic felid (cat) and canine (dog) vision models showed a marked difference from higher human vision model values: weighted mean of RNL pattern contrast (VCA:MSL) and weighted standard deviation of RNL luminance pattern contrast (VCA:sSL). The comparison of clustering results between seasons (summer, winter) did not show marked differences (Fig. S4 ).
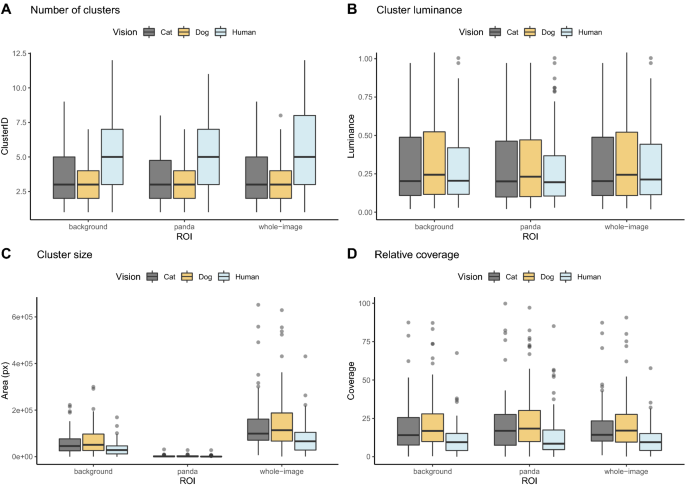
Background matching of the giant panda. The figure shows the relationship between the measured region of interest (ROI) and vision model used. Clustering results are presented for ( A ) number of clusters formed, ( B ) cluster luminance distribution, ( C ) cluster size measured in pixels (thus, de facto area is arbitrary and represents between group differences), and ( D ) the relative coverage of cluster size within the measured ROI (background, panda, whole image). The clustering method uses spatial filtering to test which visual elements cluster together in the image. The vision modelling was performed using acuity-corrected cone-catch images (canine vision—dog 12 cpd and felid vision—cat 10 cpd, the dichromatic predator surrogates, and the trichromatic human vision 72 cpd).
Quantification of disruptive coloration
We found that giant panda edge disruption was poor at close proximity (Figs. 5 and S5 ), compared to edge disruption that was measured further away. The minimum (or poorest) edge disruption was observed at approximately 12 m (at 8 relative body lengths distance). We speculate that maximal edge disruption should be close to 50 percent, because beyond this the ‘false edges’ become predominant, leading to the same ‘problem’ of having an outline. At a distance of approximately 50 m (approximated as 34 relative giant panda body lengths), however, edge disruption increased steeply for dichromats, after which it levelled out at even longer distances (edge disruption, distance by vision model interaction, F 2,430 = 39.77, p < 0.001, Fig. 4 ). Significantly, canine and felid vision systems had steeper edge disruption by distance dependency than human vision, which may suggest that edge disruption works particularly well against visual systems with lower acuity.
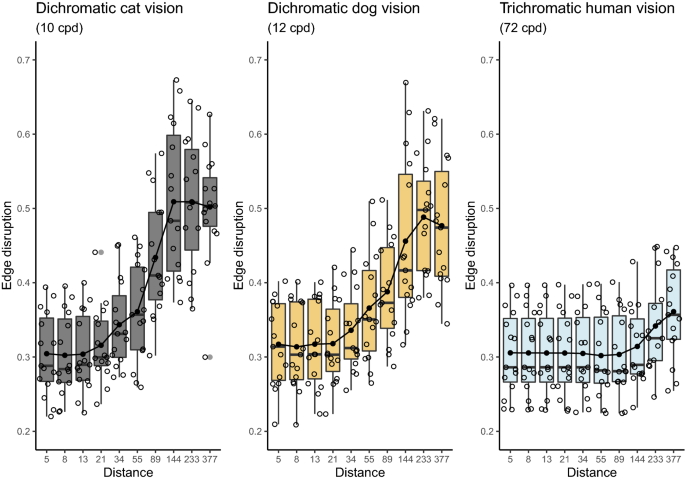
Giant panda edge disruption. The method uses Gabor filtering to quantify the ratio of false edges that run orthogonal to the animal’s true outline. Edge disruption was modelled through three visual models and their respective acuities: dichromatic felid (cat—10 cpd) and canine (dog—12 cpd), as well as trichromatic human vision (72 cpd) for comparison. The edge disruption increases as a function of distance before levelling out at longer distances. The boxplot shows minimum and maximum (whiskers), median horizontal line and the interquartile range of the simulated data.
Debunking the myth of conspicuousness: how similar is the colour of the giant panda to its natural habitat and in comparison to other species?
The giant panda is apparently conspicuous to the human eye when seen at short viewing distances. In its natural habitat, however, on average 50% of all colours within an image are perceptually similar to human vision between a giant panda and its visual background (i.e., similarity-to-background chromatic overlap, n = 15 images, s.d. = 0.1) as judged from colour space histograms (Figs. 6 and S2 ). Colour space overlap comparisons of 15 species, whose adaptive significance has been demonstrated quantitatively, confirmed the expectation that the highest degree of average chromatic similarity-to-background was found among well-camouflaged desert rodents, whereas the lowest degree of average chromatic similarity was found among aposematic poison frogs (similarity index by species, F 14,134 = 18.99, p < 0.001). Giant pandas fall between jerboas and shore crabs, species classically assumed to be cryptic in the field (Fig. 6 ). These results debunk the common misconception that the giant panda would be conspicuous to human vision in their natural habitat.
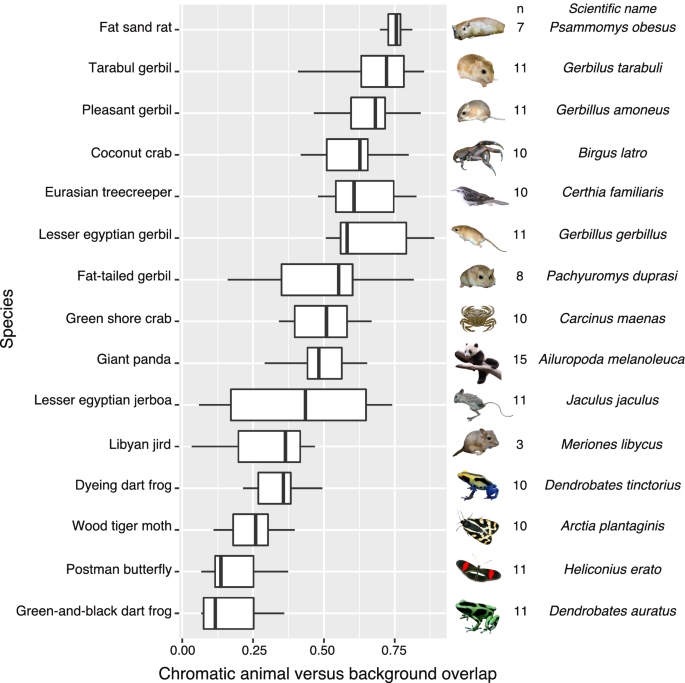
Similarity to background measured as overlap in colour space. The chromaticity diagram using human vision shows that 50% of all colours within images were perceptually similar between the giant panda and its background. The least similar species to their respective backgrounds are those usually considered as being aposematic, whereas the other end of the spectrum consists of the camouflaged animals. The giant panda falls at the middle of this ‘conspicuity spectrum’, and among other species that are considered as camouflaged, suggesting that giant panda pelage coloration does not stand out against the background more than a concealed species would. All inset pictures: Wikimedia commons open access license (CC BY-SA 3.0).
We have shown that the iconic black-and-white coloration of the giant panda is cryptic in its natural environment. Furthermore, the giant panda shows distance-dependent disruptive coloration 21 , 23 . Although disruptive coloration has been discussed in relation to other mammalian taxa before 24 , 25 , this is the first time to our knowledge that disruptive coloration has been shown to occur in mammals using a quantitative set of computational image analysis techniques.
First, we demonstrated that giant panda fur pelage shares luminance appearance (i.e., has similar counterparts) with its natural visual environment. The black pelage patches blend with dark shadows and tree trunks, thus providing concealment against darker elements in the visual scene whereas white patches match foliage and snow (when present). The brightness variation of the background highlights explains why white is associated with foliage, which are snow-covered during winter and have a waxy outer cuticle (causing specular lighting) during summer. Thus, it seems plausible that white patches can provide concealment in well illuminated forest gaps even without snow, when small gaps in the canopy allow bright light to penetrate the forest. In addition, infrequent pelage midtones match the ground and rocks. We argue that these midtones enhance background matching in the giant panda by providing an intermediate coloration, which bridges the gap between the very dark and very light visual elements in its habitat. Plausibly, the midtones allow more effective background matching than if the panda were just black-and-white. This provides adaptive insight into the brown-and-white coloration of Sichuan and Qinling giant panda individuals, because similar (brown) midtones replace black pelage on individuals in these populations. In the natural visual environment, which mostly consists of bright illuminant small gaps, larger gaps and partial woodland shade 12 , the contrasting black-and-white coloration thus facilitates background matching. The results obtained from both dichromatic and trichromatic vision models were consistent, although two metrics (weighted mean of RNL pattern contrast and weighted standard deviation of RNL luminance pattern contrast) suggest that dichromats may perceive the giant panda’s coloration more uniformly than trichromats. Overall, our data confirm previously reported findings (based on an independent and different comparative methodology) that giant panda coloration matches its background 11 .
Second, using Gabor filtering 19 and three vision models, we have shown that the giant panda demonstrates disruptive coloration operating as a function of distance: edge disruption is greater at longer distances in comparison with shorter viewing distances, where there may be more reliance on background matching. The latter would always be expected to operate as a function of distance: when an animal is far enough away both it and its background will be blurred, at which point background matching will obtain regardless of the spatial structure of individual colours. In this context, disruptive coloration is particularly interesting because there appears to be an intermediate stage when the spatial structure of the disruptive elements can be resolved even when the target shape cannot. Interestingly, we found that dichromatic visual systems with lower visual acuity than human vision showed steeper edge disruption by distance. Disruptive coloration may therefore work particularly well against visual systems with low acuity, especially when viewed over longer distances. Our results support the idea that edge disruption would be easier to detect on animals further away and with low visual acuity, because as the distance between predator and prey increases, the latter’s outline becomes less visible than the disruptive markings. Plausibly, disruption of the giant panda’s outline can no longer be resolved by the visual system of a potential predator at some ecologically relevant viewing distances, because spatial visual acuity is often poor in large carnivores 26 , and leads to distance dependent pattern blending 21 , 23 . For example, spotted hyaenas ( Crocuta crocuta ) cannot resolve the widest stripes on a plains zebra ( Equus burchelli ) beyond 50 m distance under lighting conditions when they hunt 26 . At large viewing distances, we believe that both the disruptive markings and the animal’s outline will be undetectable 21 , 27 , 28 . We suspect that the giant panda’s coloration shows features of both maximum disruptive coloration and differential blending depending on who views them and from which distance 8 , 15 , 16 .
Third, our data busted the myth of conspicuousness of giant pandas. Although at close distances, as in captive settings, the species appears conspicuous to humans, giant pandas share more than half of their colours with their natural habitat. In terms of a similarity-to-background index (i.e., chromatic animal versus background overlap) using the colour map technique, the giant panda groups together with several species widely considered as camouflaged.
Any appearance in nature is dependent on viewing conditions and perceptual sensitivities, as well as the visual acuity of the observer. In light environments which mostly consist of bright illuminant small gaps, larger gaps and partial woodland shade 12 , contrasting black-and-white coloration can serve as both background matching and disruptive coloration, and this possibility should be explored further in other animals with conspicuous black-and-white markings. It is plausible though that the black-and-white coloration is conspicuous close up, and we cannot therefore discount the possibility that visual signalling, whether for conspecifics or heterospecifics, would be important for the giant panda 29 , a conclusion reached by Caro and colleagues 11 for the black eye and ear markings of this species. In summary, the giant panda uses black-and-white pelage as a form of crypsis to avoid detection in its natural habitat and its apparent conspicuousness to the human eye is an artefact of the short viewing distances found in photography and zoos.
Photographs of the giant pandas in their natural habitat
The giant panda lives in fragmented, humid and remote mountain forests in Sichuan, Shaanxi and Gansu Province in south-central China, which makes it difficult to obtain data on this endangered animal in its natural habitat 11 . Fifteen encounters (assumed to be of different individuals) were photographed from 2007 to 2014 by FW in Sanguanmiao Field Station, Foping Nature Reserve, Shaanxi Province. The photographic data were collected from a narrow geographic range, but we have no reason to expect that our results would be limited to only within our sampling region.
Animals were photographed from various distances (estimated to be approximately 5–150 m) as they were encountered in their environment. The timing of the photographs varied across seasons: some (7) were photographed when there was snow on the ground whereas others (8) were photographed in summer conditions. The environment in these areas comprises several distinctive visual elements such as rocks, ground substrate, small streams, primary forest tree trunks, secondary growth shrubs (including bamboo), foliage and a mixture of alternating bright sunlit spots and dark shady areas. The forest geometry plausibly corresponds to light profile of forest shade with small light gaps 12 . As it was not feasible to explore the irradiance profiles of these different forest patches here, we used standard D65 illumination in the photographs as a proxy. For details of photography and using the micaToolbox as well as QCPA see appendices (Table S1 ).
Image analysis techniques
We examined photographs of 15 giant pandas using the Multispectral Image Calibration and Analysis (MICA) toolbox in Image J (version 1.52 k/Java 1.8.0_172 (64-bit) 17 and its extension, the Quantitative Colour and Pattern Analysis (QCPA) framework 18 —micaToolbox version 15.3.2019. Photographs were processed in digital negative format (.dng) and we used a Canon EOS400D with kit specifications (18–55 mm lens) in quantitative colour and pattern analysis framework (for details see Table S1 ). Each image was converted into multispectral image stacks as follows. The photographs’ white balance was adjusted through the MICA toolbox plugin with ImageJ software and was normalised to 7 percent black using the giant panda black (dark tones) and 63 percent white reflectance using the white (light tones) pelage patches and 97 percent snow reflectance as proxies (Table S1 ). The reflectance values of the pelages were confirmed prior to analyses. For this, two captive giant pandas were photographed in Ähtäri Zoo, Finland, next to calibrated photographic standards (Fig. S1 ). Note that the exact values of fur reflectance are not crucial, but it is important to normalise the dynamic light range across the photographs 30 . We used panda size to standardise scale across the photographs: length was used as ‘a measuring stick’ to approximate relative distance in the edge disruption analysis (see below). As regions of interest (ROIs), the entire animal outline (animal) and the remaining surrounding visual environment excluding the animal (background) were selected.
Quantification of background matching
We tested how closely the pelage patches of the giant panda clusters with the visual patchiness of the background. The RNL clustering method uses spatial filtering to determine which luminance elements group together in the image (i.e., the method clusters pixels according to their perceived colour or luminance contrast—our approach for this question takes account of luminance only, Table S1 —as measured in Euclidian distance between pixels in the RNL colour space). For details see 18 . Acuity-corrected cone catch images were used without distance scaling for three vision models: domestic dog and cat, and human vision. Details are reported in Table S1 . The obtained clusters were counted as dummy variables (i.e., dichotomous: true or false) with respect to whether or not each pelage grouped with specified background visual features; associations between giant panda pelage patches and the ground, leaves, rocks, shades, snow and tree trunks were determined using relative frequencies (i.e., number of associations divided by the total number of samples). We also used the QCPA’s Visual Contrast Analysis (VCA) for detailed luminance analysis (Table S2 ), and to confirm the validity of human vision modelled data in comparison to dichromatic vision systems (domestic dog and cat) with the following visual acuities: dog 12 cpd 31 , cat 10 cpd 32 , 33 , and human 72 cycles per degree—cpd 27 .
We tested whether free-living giant pandas show disruptive coloration operating as a function of distance, using Gabor filtering of achromatic edge disruption which quantifies the ratio of false edges that run orthogonal to an animal’s true outline 19 . This approach allowed us to test edge disruption using only the luminance channel. The pictures were first standardised to fixed pixels per distance ratio. The distance was modelled as relative body lengths (the panda body length was set as 1, which corresponds to approximately 1.5 m). We modelled the distance to target using the acuity view tool in the toolbox 18 with Fibonacci sequence increments to obtain exponential growth for longer distances (starting at 5). A GabRat Sigma value of 3 was used 19 . We modelled edge disruption through all three vision systems explored here (cat, dog, human) and used these as a proxy for the giant panda’s would-be predators 26 , 34 , because the latter’s exact spatial acuities remain uncertain.
Exposing the myth of conspicuousness
We tested whether giant panda coloration matches natural background colour variation as judged by a colour map comparison technique 18 . These colour maps can be considered as a non-parametric histogram representation of the spectral variation captured in any image region using receptor noise limited colour space and acuity correction. The colour map tool in QCPA quantifies chromatic contrast as ΔS in the chromatic Receptor Noise Limited (RNL) space 18 . As such, it categorically ignores luminance 35 and exclusively quantifies chromatic similarity. The method allows the user to estimate the overlap of estimated colour perception between parts of images. As we wanted to question and demystify the idea of wild giant pandas being conspicuousness to us, we used human vision as a proxy. Images were first converted to normalised multispectral images, as above, and then converted to human cone catch images in D65 standard illuminant. The colour space overlap value (range 0–1, i.e. 0 to 100% similarity) was determined by opposing the human mw:lw channel against the (lw + mw):sw channel, and thus represents the view through a trichromatic visual system. The overlap was considered as a similarity-to-background index where higher values indicate higher similarity between the animal and its background (Fig. S2 ).
As the QCPA’s colour map method is relatively new and has not been widely used yet, we performed additional comparisons with other species to cross-reference where this similarity-to-background index fell in comparison to the colour patterns of species whose adaptive significance has been demonstrated quantitatively. For this, we used the following species for which we had access to multispectral images (all these are broadly considered as camouflaged): seven species of Sahara-Sahel desert rodents 36 : ( Gerbillus amoenus , G. gerbillus , G. tarabuli , Jaculus hirtipes , Meriones libycus , Pachyuromys duprasi , and Psammomys obesus ), the coconut crab ( Birgus latro ) 37 , Eurasian treecreeper ( Certhia familiaris ; Nokelainen et al. n.d.) and green shore crab ( Carcinus maenas ) 38 . In addition, ON photographed four ‘vividly-coloured’ species (all considered as aposematic): the postman butterfly ( Heliconius erato ), the green-and-black dart frog ( Dendrobates auratus ), a dyeing dart frog ( Dendrobates tinctorius ) and the wood tiger moth ( Arctia plantaginis ). The same QCPA colour map procedure as above was applied to all of these species in order to create colour maps for the reference species in the same way as for giant pandas (Table S1 ).
Statistical analysis
First, we used acuity-corrected cluster analysis to determine how well the visual patchiness of the pandas and backgrounds match. Following the cluster analysis, the number of shared visual features were counted: the relative frequencies were collated according to whether the clusters were unique to the giant panda and not found in the background, specific to the background only, or shared between the animal and the background. In addition, we determined which visual elements of the background (i.e., rocks, foliage, trunks, ground, snow, shadows) cluster with differently coloured body parts of giant pandas. The non-independency of background elements and giant panda colour patches was tested with Pearson’s Chi-squared test. The number of visual clusters formed, cluster luminance, cluster size and their relative coverage were tested in a lmer-model (Tables S3 – S7 ). Second, we used Gabor filtering 19 to quantify the ratio of false edges that run orthogonal to an animal’s true outline. The edge disruption index was used as a dependent variable and the modelled distance and its interaction with the vision model were used as explanatory variables in a lmer-model (lme4-package in r) with ID set as a random factor to control data structure (Table S8 ). Third, to test whether giant pandas are camouflaged either through background matching, disruptive coloration or both, we used colour space overlap values as a similarity-to-background index and applied this as the dependent variable in a linear model with reference species as the independent variable. Analyses were performed in R studio (R version 3.6.1/2019-07-05).
Caro, T. The adaptive significance of coloration in mammals. Bioscience 55 , 125 (2005).
Article Google Scholar
Caro, T. The colours of extant mammals. Semin. Cell Dev. Biol. 24 , 542–552 (2013).
Schaller, G. B., Jinchu, H., Wenshi, P. & Jing, Z. The Giant Pandas of Wolong (University of Chicago Press, 1985). https://doi.org/10.1086/414647 .
Book Google Scholar
Schaller, G. B. The Last Panda (University of Chicago Press, 1994).
Google Scholar
Morris, R. & Morris, D. Men and Pandas (McGraw-Hill Book Company, 1966).
Morris, R. & Morris, D. The Giant Panda (Penguin Books, 1982).
Lazell, J. D. J. Color Patterns of the ‘Giant’ Bear (Ailuropoda melanoleuca) and the True Panda (Ailurus fulgens) (Mississippi Wildlife Federation, 1974).
Cott, H. B. Adaptive Coloration in Animals (Methuen & Co., Ltd., 1940).
Endler, J. A. On the measurement and classification of colour in studies of animal colour patterns. Biol. J. Linn. Soc. 41 , 315–352 (1990).
Stevens, M. & Merilaita, S. Animal camouflage: Current issues and new perspectives. Philos. Trans. R. Soc. B Biol. Sci. 364 , 423–427 (2009).
Caro, T., Walker, H., Rossman, Z., Hendrix, M. & Stankowich, T. Why is the giant panda black and white?. Behav. Ecol. 28 , 657–667 (2017).
Endler, J. A. The color of light in forests and its implications. Ecol. Monogr. 63 , 1–27 (1993).
Merilaita, S. Crypsis through disruptive coloration in an isopod. Proc. R. Soc. B Biol. Sci. 265 , 1059–1064 (1998).
Cuthill, I. C. et al. Disruptive coloration and background pattern matching. Nature 434 , 72–74 (2005).
Article ADS CAS Google Scholar
Stevens, M. & Merilaita, S. Defining disruptive coloration and distinguishing its functions. Philos. Trans. R. Soc. B Biol. Sci. 364 , 481–488 (2009).
Ruxton, G., Allen, W., Sherratt, T. & Speed, M. Avoiding Attack: The Evolutionary Ecology of Crypsis, Aposematism, and Mimicry (Oxford University Press, 2019).
Troscianko, J. & Stevens, M. Image calibration and analysis toolbox—A free software suite for objectively measuring reflectance, colour and pattern. Methods Ecol. Evol. 6 , 1320–1331 (2015).
van den Berg, C. P., Troscianko, J., Endler, J. A., Marshall, N. J. & Cheney, K. L. Quantitative colour pattern analysis (QCPA): A comprehensive framework for the analysis of colour patterns in nature. Methods Ecol. Evol. 11 , 316–332 (2020).
Troscianko, J., Skelhorn, J. & Stevens, M. Quantifying camouflage: How to predict detectability from appearance. BMC Evol. Biol. 17 , 7 (2017).
Caves, E. M. & Johnsen, S. AcuityView: An r package for portraying the effects of visual acuity on scenes observed by an animal. Methods Ecol. Evol. 9 , 793–797 (2018).
Marshall, N. J. Communication and camouflage with the same ‘bright’ colours in reef fishes. Philos. Trans. R. Soc. B Biol. Sci. 355 , 1243–1248 (2000).
Article CAS Google Scholar
Barnett, J. B., Cuthill, I. C. & Scott-Samuel, N. E. Distance-dependent aposematism and camouflage in the cinnabar moth caterpillar ( Tyria jacobaeae , erebidae). R. Soc. Open Sci. 5 , 171396 (2018).
Article ADS Google Scholar
Barnett, J. B., Cuthill, I. C. & Scott-Samuel, N. E. Distance-dependent pattern blending can camouflage salient aposematic signals. Proc. R. Soc. B Biol. Sci. 284 , 20170128 (2017).
Stoner, C. J., Caro, T. M. & Graham, C. M. Ecological and behavioral correlates of coloration in artiodactyls: Systematic analyses of conventional hypotheses. Behav. Ecol. 14 , 823–840 (2003).
Caro, T., Walker, H., Santana, S. E. & Stankowich, T. The evolution of anterior coloration in carnivorans. Behav. Ecol. Sociobiol. 71 , 177 (2017).
Melin, A. D., Kline, D. W., Hiramatsu, C. & Caro, T. Zebra stripes through the eyes of their predators, zebras, and humans. PLoS ONE 11 , e0145679 (2016).
Land, M. F. & Nilsson, D.-E. Animal Eyes (Oxford University Press, 2012).
Phillips, G. A. C., How, M. J., Lange, J. E., Marshall, N. J. & Cheney, K. L. Disruptive colouration in reef fish: Does matching the background reduce predation risk?. J. Exp. Biol. 220 , 1962–1974 (2017).
Li, Y. et al. Giant pandas can discriminate the emotions of human facial pictures. Sci. Rep. 7 , 1–8 (2017).
ADS Google Scholar
Stevens, M., Párraga, C. A., Cuthill, I. C., Partridge, J. C. & Troscianko, T. S. Using digital photography to study animal coloration. Biol. J. Linn. Soc. 90 , 211–237 (2007).
Lind, O., Milton, I., Andersson, E., Jensen, P. & Roth, L. S. V. High visual acuity revealed in dogs. PLoS ONE 12 , 1–12 (2017).
Pasternak, T. & Merigan, W. H. The luminance dependence of spatial vision in the cat. Vis. Res. 21 , 1333–1339 (1981).
Clark, D. L. & Clark, R. A. Neutral point testing of color vision in the domestic cat. Exp. Eye Res. 153 , 23–26 (2016).
Caves, E. M., Brandley, N. C. & Johnsen, S. Visual acuity and the evolution of signals. Trends Ecol. Evol. 33 , 1–15 (2018).
Vorobyev, M. & Osorio, D. Receptor noise as a determinant of colour thresholds. Proc. R. Soc. B Biol. Sci. 265 , 351–358 (1998).
Nokelainen, O., Brito, J. C., Scott-Samuel, N. E., Valkonen, J. K. & Boratyński, Z. Camouflage accuracy in Sahara-Sahel desert rodents. J. Anim. Ecol. https://doi.org/10.1111/1365-2656.13225 (2020).
Article PubMed Google Scholar
Nokelainen, O., Stevens, M. & Caro, T. Colour polymorphism in the coconut crab ( Birgus latro ). Evol. Ecol. 32 , 75–88 (2018).
Nokelainen, O., Maynes, R., Mynott, S., Price, N. & Stevens, M. Improved camouflage through ontogenetic colour change confers reduced detection risk in shore crabs. Funct. Ecol. https://doi.org/10.1111/1365-2435.13280 (2019).
Article PubMed PubMed Central Google Scholar
Download references
Acknowledgements
We thank one anonymous reviewer, Olivier Penacchio, Martin Stevens, Cedric van den Berg and Jolyon Troscianko for constructive feedback on a previous version of this manuscript. Sandra Winters kindly helped with the cat vision model. We thank Ähtäri Zoo and its staff for helping to take the calibration photos. We thank Ron Swaisgood for introducing FW and YN to TC. This research was funded by the Academy of Finland Postdoctoral Research Fellow grant (#21000038821) and the Strategic Priority Research Program of Chinese Academy of Sciences (XDB31000000).
Author information
Authors and affiliations.
Department of Biological and Environmental Science, University of Jyväskylä, P.O. Box 35, 40014, Jyväskylä, Finland
- Ossi Nokelainen
School of Psychological Science, University of Bristol, Bristol, UK
Nicholas E. Scott-Samuel
Key Laboratory of Animal Ecology and Conservation Biology, Chinese Academy of Sciences, Beijing, China
Yonggang Nie & Fuwen Wei
Center for Excellence in Animal Evolution and Genetics, Chinese Academy of Sciences, Kunming, China
School of Biological Sciences, University of Bristol, Bristol, BS8 1TQ, UK
Center for Population Biology, University of California, 1 Shields Avenue, Davis, CA, 95616, USA
You can also search for this author in PubMed Google Scholar
Contributions
O.N. analysed the data and wrote the first version of the manuscript. N.E.S.-S. helped to conceptualise the manuscript. Y.N. and F.W. provided the original images and contributed on writing the manuscript. T.C. edited the manuscript and brought people together for this project.
Corresponding authors
Correspondence to Ossi Nokelainen or Tim Caro .
Ethics declarations
Competing interests.
The authors declare no competing interests.
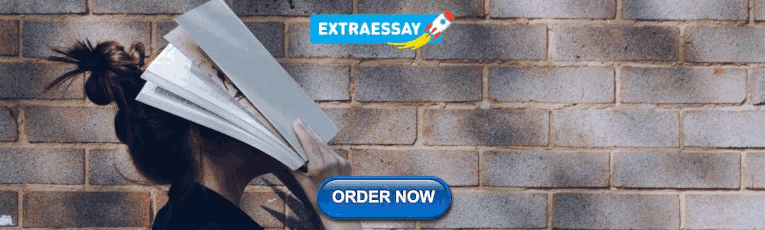
Additional information
Publisher's note.
Springer Nature remains neutral with regard to jurisdictional claims in published maps and institutional affiliations.
Supplementary Information
Supplementary information 1., rights and permissions.
Open Access This article is licensed under a Creative Commons Attribution 4.0 International License, which permits use, sharing, adaptation, distribution and reproduction in any medium or format, as long as you give appropriate credit to the original author(s) and the source, provide a link to the Creative Commons licence, and indicate if changes were made. The images or other third party material in this article are included in the article's Creative Commons licence, unless indicated otherwise in a credit line to the material. If material is not included in the article's Creative Commons licence and your intended use is not permitted by statutory regulation or exceeds the permitted use, you will need to obtain permission directly from the copyright holder. To view a copy of this licence, visit http://creativecommons.org/licenses/by/4.0/ .
Reprints and permissions
About this article
Cite this article.
Nokelainen, O., Scott-Samuel, N.E., Nie, Y. et al. The giant panda is cryptic. Sci Rep 11 , 21287 (2021). https://doi.org/10.1038/s41598-021-00742-4
Download citation
Received : 09 July 2021
Accepted : 15 October 2021
Published : 28 October 2021
DOI : https://doi.org/10.1038/s41598-021-00742-4
Share this article
Anyone you share the following link with will be able to read this content:
Sorry, a shareable link is not currently available for this article.
Provided by the Springer Nature SharedIt content-sharing initiative
This article is cited by
Predator selection on phenotypic variability of cryptic and aposematic moths.
- Sanni A. Silvasti
- Johanna Mappes
Nature Communications (2024)
Black-and-white pelage as visually protective coloration in colobus monkeys
- Sandra Winters
Behavioral Ecology and Sociobiology (2024)
Automated workflows using Quantitative Colour Pattern Analysis (QCPA): a guide to batch processing and downstream data analysis
- Cedric P. van den Berg
- Nicholas D. Condon
- Karen L. Cheney
Evolutionary Ecology (2024)
By submitting a comment you agree to abide by our Terms and Community Guidelines . If you find something abusive or that does not comply with our terms or guidelines please flag it as inappropriate.
Quick links
- Explore articles by subject
- Guide to authors
- Editorial policies
Sign up for the Nature Briefing: Anthropocene newsletter — what matters in anthropocene research, free to your inbox weekly.

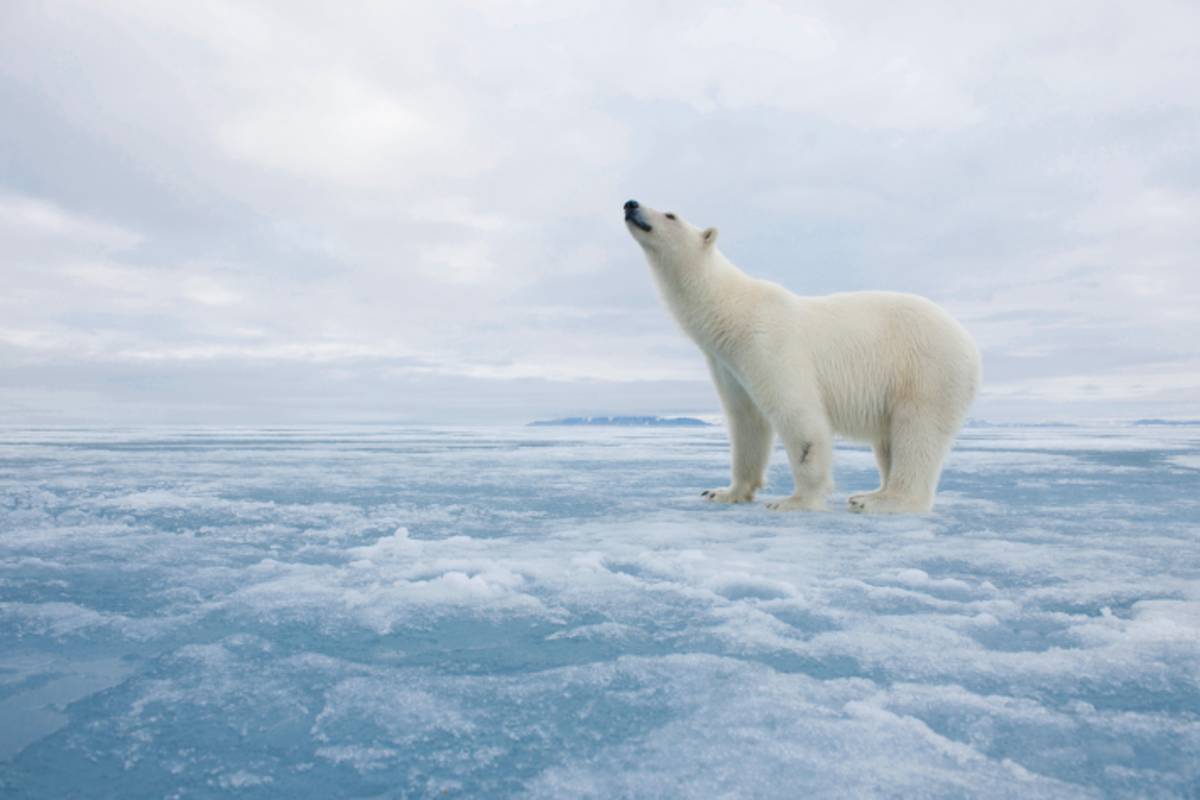
Photo: Daniel J. Cox
Polar Bear Research in a Changing Arctic
By Todd. C. Atwood
11 May 2023
Share on social
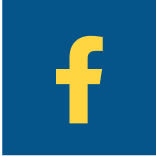
In January of 1992, a lone female cub was born in a den located on the lee side of a pressure ridge on the pack ice of the Beaufort Sea. In late March, the mother bear popped her nose through the den’s ceiling to let in the first rays of daylight for her cub, and over the next week slowly acclimated the cub to life outside the den. Eventually, the mom felt confident in her cub’s ability to tolerate the challenging environment and left the den for good to find her first meal in roughly five months. That April, researchers from the U.S. Geological Survey’s Polar Bear Research Program captured the family on the sea ice north of Prudhoe Bay, Alaska—giving the mom and her cub small ear tags with unique identification numbers and adding them to the database.
Twenty years later, I was part of a research team flying in a helicopter over the snow-covered sea ice of the southern Beaufort Sea in search of polar bears to sample for the program’s long-term population dynamics study, research that helps us understand how the bears are faring. We had been slowly flying over a network of intertwined leads (narrow slivers of open water between ice floes) when someone shouted, “There’s a bear!” It took me a moment to isolate the small flash of pale yellow within the flat light of the vast and snowy-white background. It was my first time seeing a polar bear in the wild and it was majestic. Once we were on the ice with the bear, we noted the ear tag indicating a previous capture. The grizzled 20-year-old, 200-kilogram female was the same individual captured as a 13-kilogram cub in 1992.
I have since seen hundreds of polar bears and the excitement has never waned. My colleagues and I are privileged to study the bears and feel the weight on our shoulders to continue to produce rigorous and timely science that can be used to ensure their long-term persistence. But over the past two decades, a looming question has emerged: How do you study an ice-dependent species when your study area is melting away?
Photo: Kt Miller / Polar Bears International
The sea ice in Svalbard, Norway, where Polar Bears International conducts our annual maternal den study, has become less predictable in recent years, impacting research in the area.
A changing Arctic and the loss of “bear-able” days
The loss of sea ice habitat from human-caused climate warming is the primary threat to polar bears—and to the ability of researchers to study them. In 1992, sea ice was present in the southern Beaufort Sea throughout the year. In 2012, it was functionally ice-free in August and September. More recently, it has been ice-free, on average, for upwards of three months each year. The ice-free period is expected to continue to increase in duration unless greenhouse gas emissions are meaningfully reduced.
Changes to summer and fall sea ice conditions have been dramatic and garnered a lot of media attention. But winter and spring conditions have also deteriorated, which has increased the challenges and risks to field crews working on the sea ice. The thinner first-year ice that now dominates the southern Beaufort Sea is more mobile in winter and spring. It has become harder to judge ice stability and thickness—critical factors when deciding whether it’s safe to capture a bear.
Moreover, the frequency of wind-storm events in winter and spring has increased. Strong winds can crack and shear the sea ice, further fragmenting habitat and creating expanses of open water. Water vapor from the warmer exposed ocean mixes with the much colder air and condenses into fog. The fog can arrive with little warning, grounding air crews or leading to “boomerang flights” where conditions are suitable for take-off but deteriorate offshore, necessitating a hasty retreat to avoid getting stuck on the ice. Since 2001, our program has experienced a substantial decline in “bear-able” days (i.e., days we are able to fly and search for bears) due to poor weather conditions like extensive fog or high winds that create dangerous flight conditions. From 2001-05, we were grounded due to weather an average of 23% of the field season. From 2017-21, we were grounded an average of 56% of the field season.
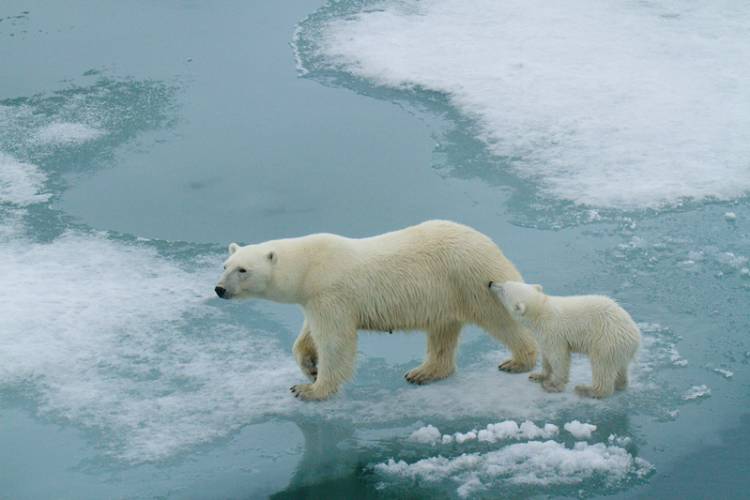
Coping with uncertainty
If you are familiar with the television series Breaking Bad, then you probably recognize the name Heisenberg. Werner Heisenberg was a theoretical physicist best known for his uncertainty principle, which states (paraphrasing) there will always be a fundamental limit to the accuracy we can achieve for an estimated or predicted value. That said, a primary goal for scientists is to strive to reduce the uncertainty of results.
Consider wildlife abundance estimates. Accurate and precise population abundance estimates are “the coin of the realm” in wildlife biology. When accuracy and precision are high, uncertainty is low, and managers feel confident using those abundance estimates to inform essential management actions.
One of the ways to reduce uncertainty is to increase sample size—i.e., the number of observations analyzed. You can probably see the conundrum here for polar bear research: How do you increase sample size when the number of bear-able days is declining?
Our solution has been to invest in diversifying the methods for collecting data. Capture and handling bears (collecting measurements, taking samples for health assessments, and attaching tracking devices) yields the most diverse and highest quality data. Yet, deteriorating ice conditions are already impacting our work and will make capture less feasible in the years to come. Looking ahead, it will be important to adapt data collection methods to the sea ice conditions encountered during a given field season. In years when capture is infeasible, field crews will need to seamlessly switch to remote sampling methods like biopsy darting, passive genetic sampling (e.g., hair and scat collection), and observational surveys, even though they yield less data. We are also investing in developing statistical methods that can integrate multiple data sources, including Indigenous Knowledge.
A final thought
I often think about that first bear I saw in 2012. I wonder if she’s still alive (if so, she’d be 30 years old) and how she coped with the dramatic changes to sea ice habitat that occurred over her lifetime. Polar bear researchers also face challenges—not existential like the bears, but in continuing to provide rigorous science to guide conservation efforts. The U.S. Geological Survey’s Polar Bear Research Program is working to meet those challenges head-on. But time is running short to reduce greenhouse gas emissions, conserve Arctic sea ice, and ensure the long-term persistence of polar bears.
Dr. Todd Atwood is a research biologist and project leader with the U.S. Geological Survey’s Polar Bear Research Program. He has studied polar bears since 2012.
Related Articles
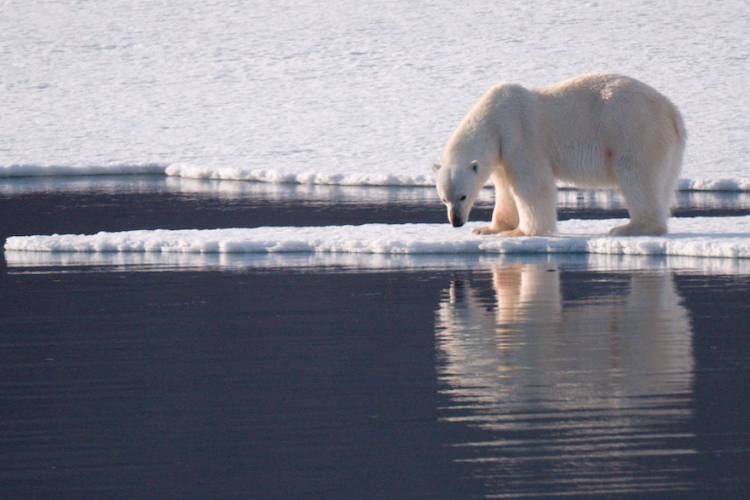
Climate Warming
Shaping Policy for the Planet and Polar Bears
What exactly is policy and what does it have to do with polar bears? And how can I, as a citizen, help influence leaders and bring about change? Alex Shahbazi breaks it down for us in an approachable way.
04 Apr 2024
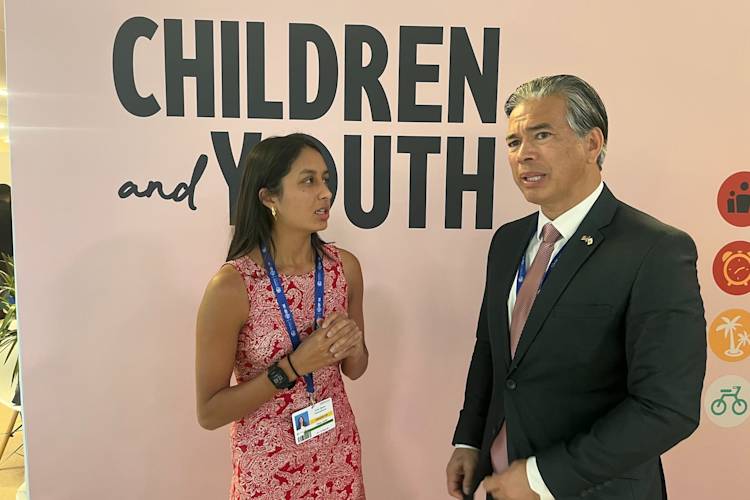
What the U.N. Climate Talks Can Teach Us About Local Action
Youth climate activist and leader, Elise Joshi, reflects on her experience at COP28 and finding comfort in her realization that the biggest impact one can make may be in your own community.
13 Feb 2024
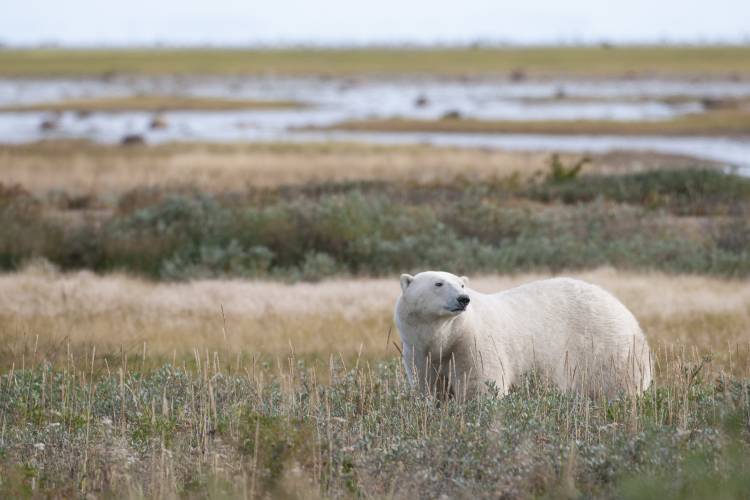
Can Polar Bears Adapt to Longer Summers on Land?
A new study from the USGS and WSU monitored polar bears on land during summer near the Hudson Bay to determine energetic loss while on land.
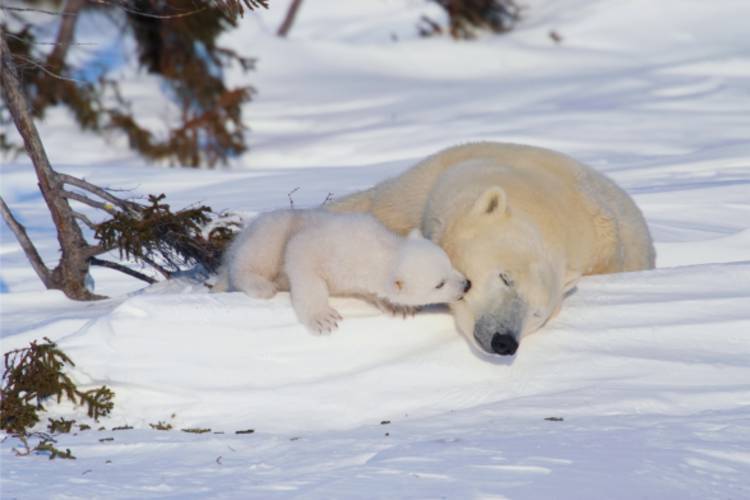
Join this conservation journey.
Become part of a community committed to protecting polar bears with our free e-news.

Help ensure the polar bear's future.
The actions we take today give polar bears a chance at an even better tomorrow.
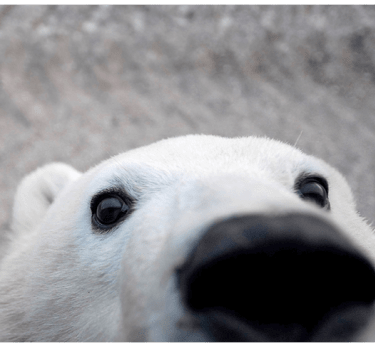
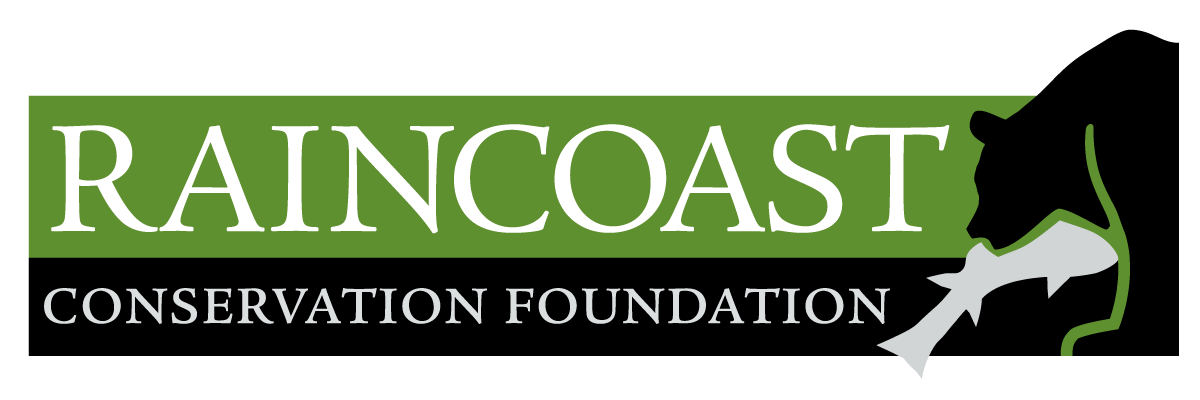
Study: Spatial patterns and rarity of the white‐phased ‘Spirit bear’ allele reveal gaps in habitat protection
These findings provide new insight into hypotheses related to the maintenance of this rare polymorphism, and directly relevant information to support evidence‐based opportunities for Indigenous Nations of the area to attend to gaps in conservation planning.
Spirit bears are rarer and less protected than we thought.
New research has identified that the small genetic change responsible for Spirit bears – a rare, white-coated form of black bears – is up to 50% rarer in the Great Bear Rainforest than previously estimated. The study also indicates that geographic hotspots, where the Spirit bear version of the gene was especially prominent lack adequate protection from resource extraction.
This research paper, published by British Ecological Society , will inform contemporary land use planning, which include the potential for increased protection in the form of Indigenous Protected Areas. Lead author Christina Service published these findings with team members from the Kitasoo Xai’xais Stewardship Authority, Gitga’at Oceans and Lands Department, University of British Columbia, University of Victoria, and Raincoast Conservation Foundation.
“We found that the frequency of the white version of the gene was as much as 50% lower compared with previous estimates. While ongoing work is estimating the number of Spirit bears in the area, such a finding questions previous estimates.” – Christina Service, Wildlife Biologist for Kitasoo/Xai’xais First Nation Stewardship Authority, Raincoast Applied Conservation Science Lab
Service CN, Bourbonnais M, Adams MS, et al. Spatial patterns and rarity of the white-phased ‘Spirit bear’ allele reveal gaps in habitat protection. Ecol SolutEvidence . 2020;e12014. https://doi.org/10.1002/2688-8319.12014
1. Preserving genetic and phenotypic diversity can help safeguard not only biodiversity but also cultural and economic values.
2. Here, we present data that emerged from Indigenous‐led research at the intersection of evolution and ecology to support conservation planning of a culturally salient, economically valuable, and rare phenotypic variant. We addressed three conservation objectives for the white‐phased ‘Spirit bear’ polymorphism, a rare and endemic white‐coated phenotype of black bear ( Ursus americanus ) in Kitasoo/Xai’xais and Gitga’at Territories and beyond in coastal British Columbia, Canada. First, we used non‐invasively collected hair samples ( n = 385 bears over ∼18,000 km 2 ) to assess the spatial variation in the frequency of the allele that controls the white‐coloured morph (mc1r). Second, we compared our observed allele frequencies at mc1r with those expected under Hardy–Weinberg equilibrium. Finally, we examined how well current protected areas in the region aligned with spatial hotspots of Spirit bear alleles.
3. We found that landscape‐level allele frequency was lower than previously reported. For example our systematic sampling estimated a frequency of 0.25 (95% CI {0.13, 0.41}) on Gribbell Island compared with the previously reported estimate of 0.56. Also, in contrast with previous reports, we failed to detect a statistically significant departure from Hardy–Weinberg equilibrium at mc1r, which calls into question the previously posited role of homozygote gene flow, heterozygote disadvantage, and positive assortative mating in the maintenance of this polymorphism. Finally, we found a discrepancy between the placement of protected areas and the 90th percentile hotspots (upper 10% of all estimated values) of Spirit bear alleles, with ∼50% of hotspots falling outside of protected areas.
4. These results provide new insight into hypotheses related to the maintenance of this rare polymorphism, and directly relevant information to support evidence‐based opportunities for Indigenous Nations of the area to attend to gaps in conservation planning.
Select figures and assets

Affiliations
Gitga’at Oceans and Lands Department, Gitga’at First Nation, Hartley Bay, British Columbia, Canada
Kitasoo Xai’xais Stewardship Authority, Kitasoo/Xai’xais First Nation, Klemtu, British Columbia, Canada
Department of Geography, University of Victoria, Victoria, British Columbia, Canada
Department of Earth, Environmental and Geographic Sciences, Irving K. Barber School of Arts and Sciences, University of British Columbia Okanagan, Kelowna, British Columbia, Canada
Raincoast Conservation Foundation, Sidney, British Columbia, Canada
Support our mobile lab, Tracker !
Our new mobile lab will enable the Healthy Waters Program to deliver capacity, learning, and training to watershed-based communities. We need your support to convert the vehicle and equip it with lab instrumentation. This will allow us to deliver insight into pollutants of concern in local watersheds, and contribute to solution-oriented practices that protect and restore fish habitat.

2020 British Ecological Society Christina Service legacy 2 Science 2020 spirit bear
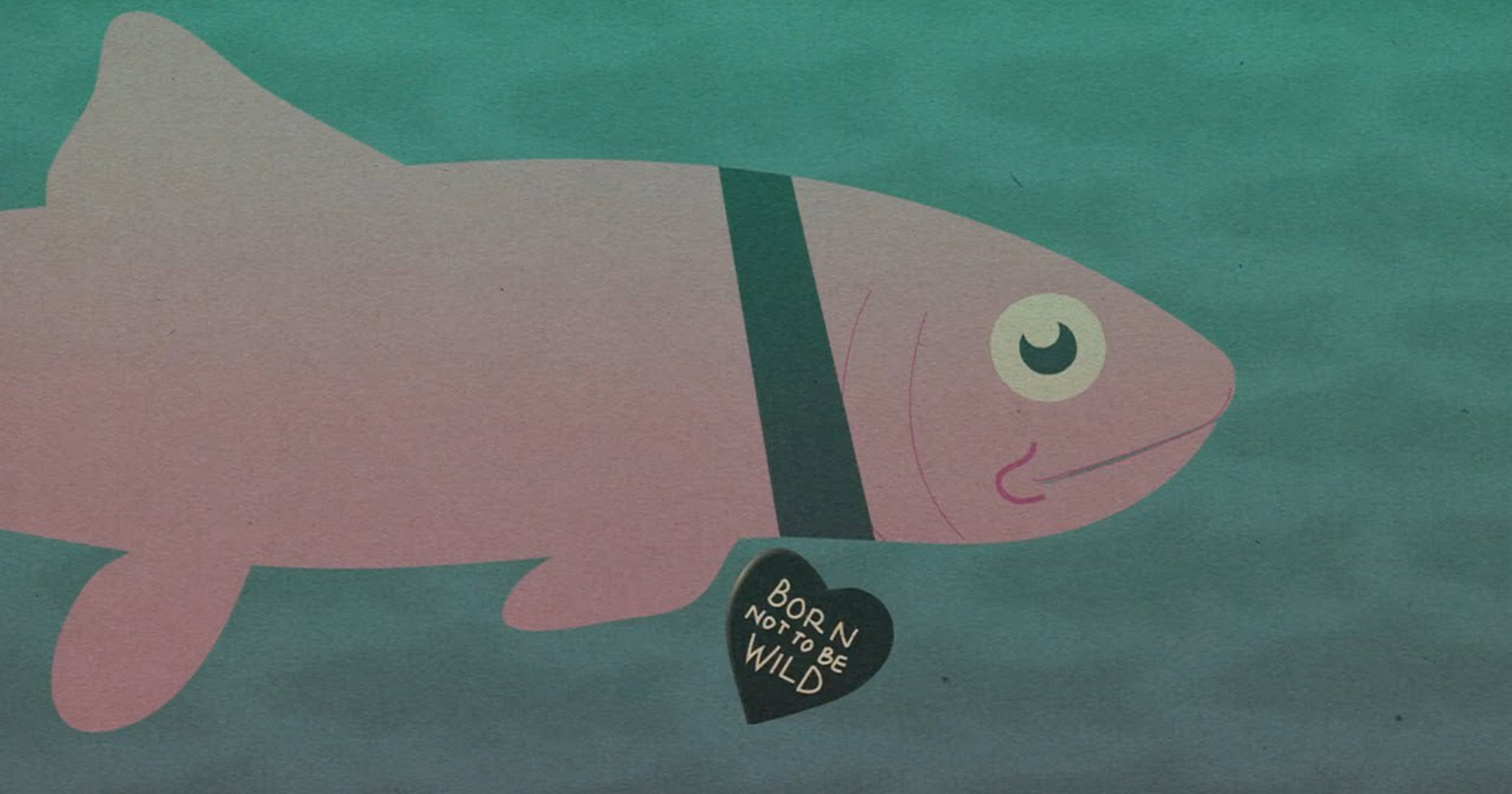
Hatcheries: good intentions, bad outcomes
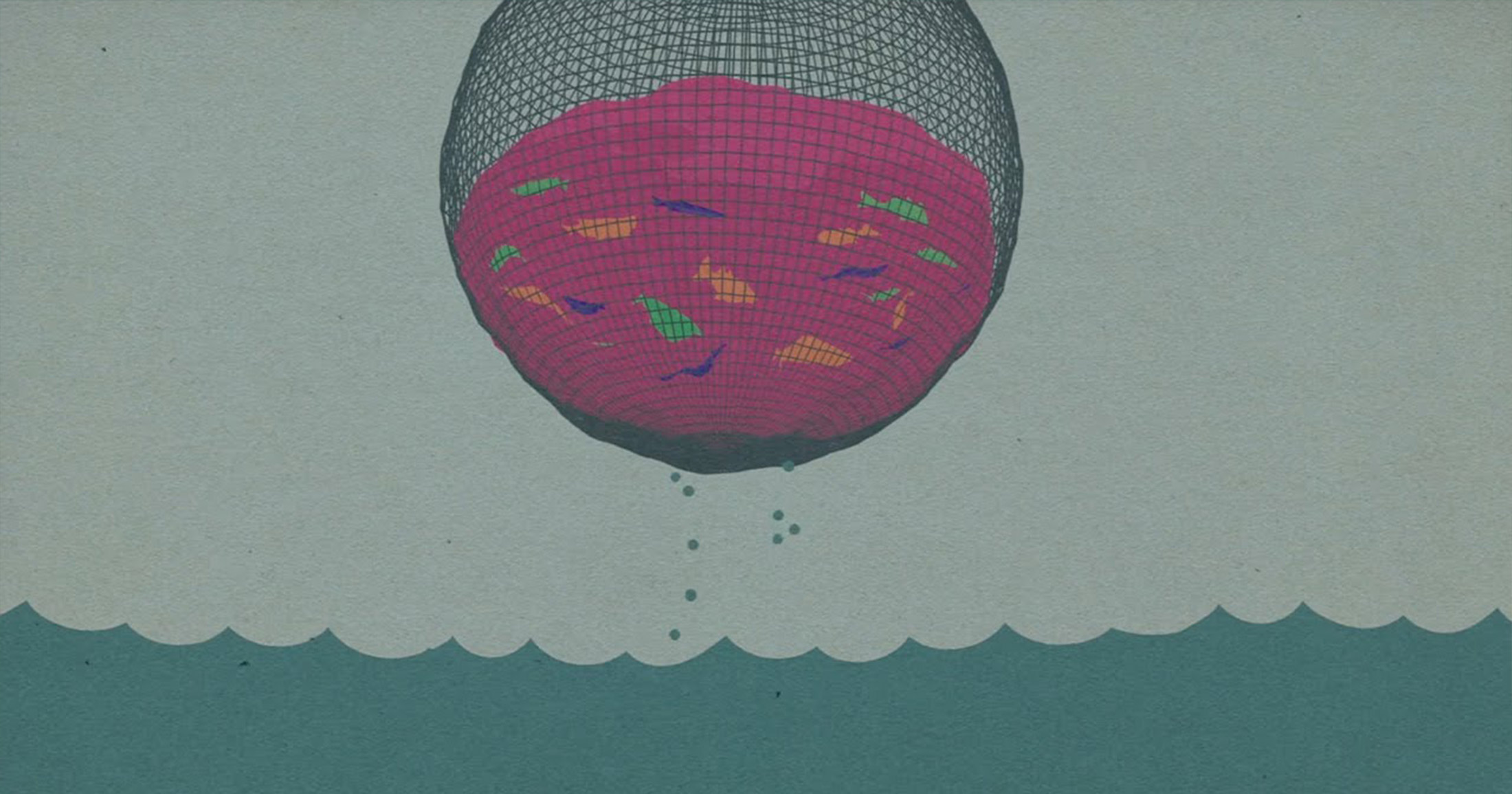
A better, older way to fish for salmon
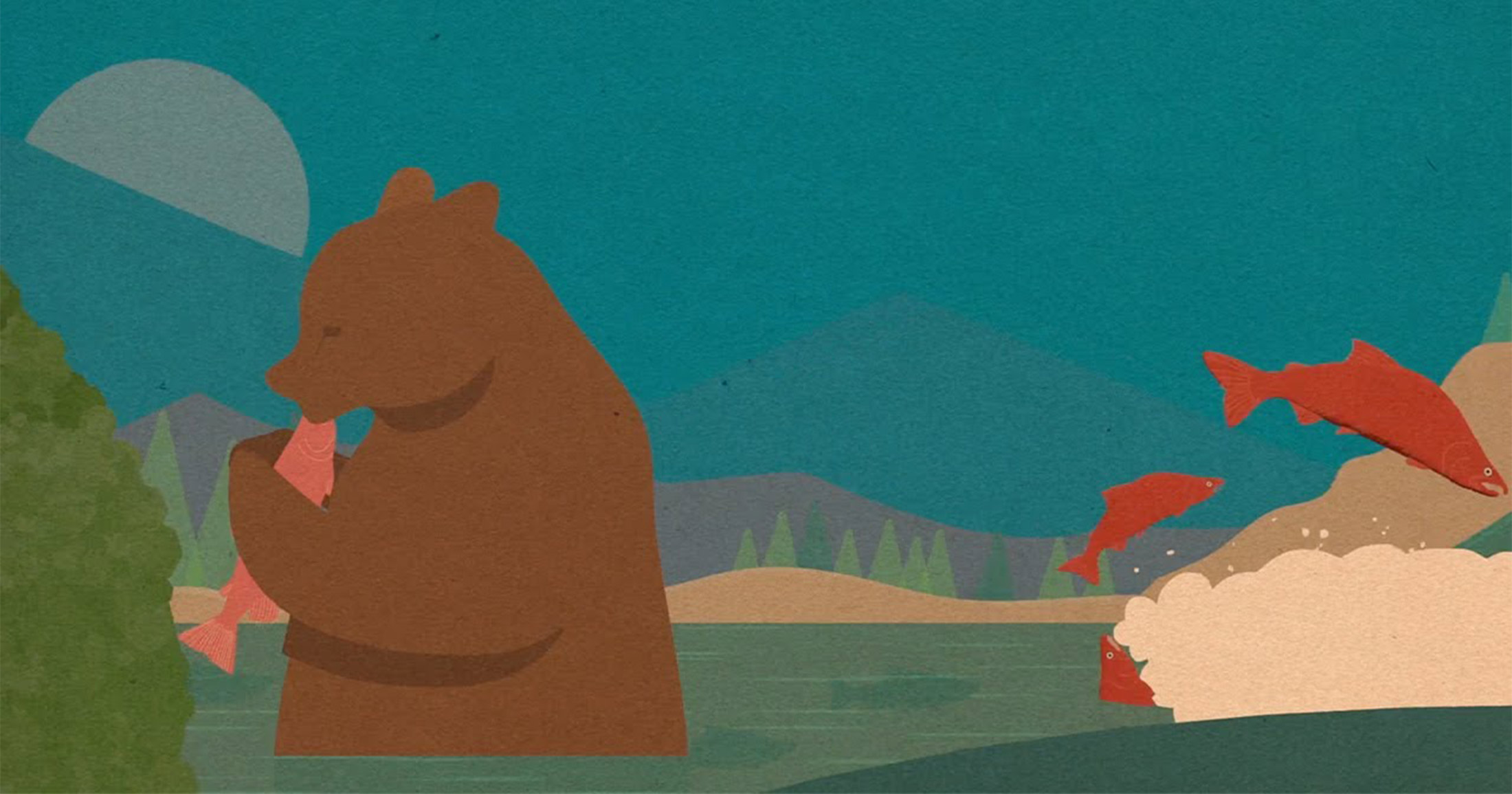
Seeing wild salmon thrive: Our wild dream
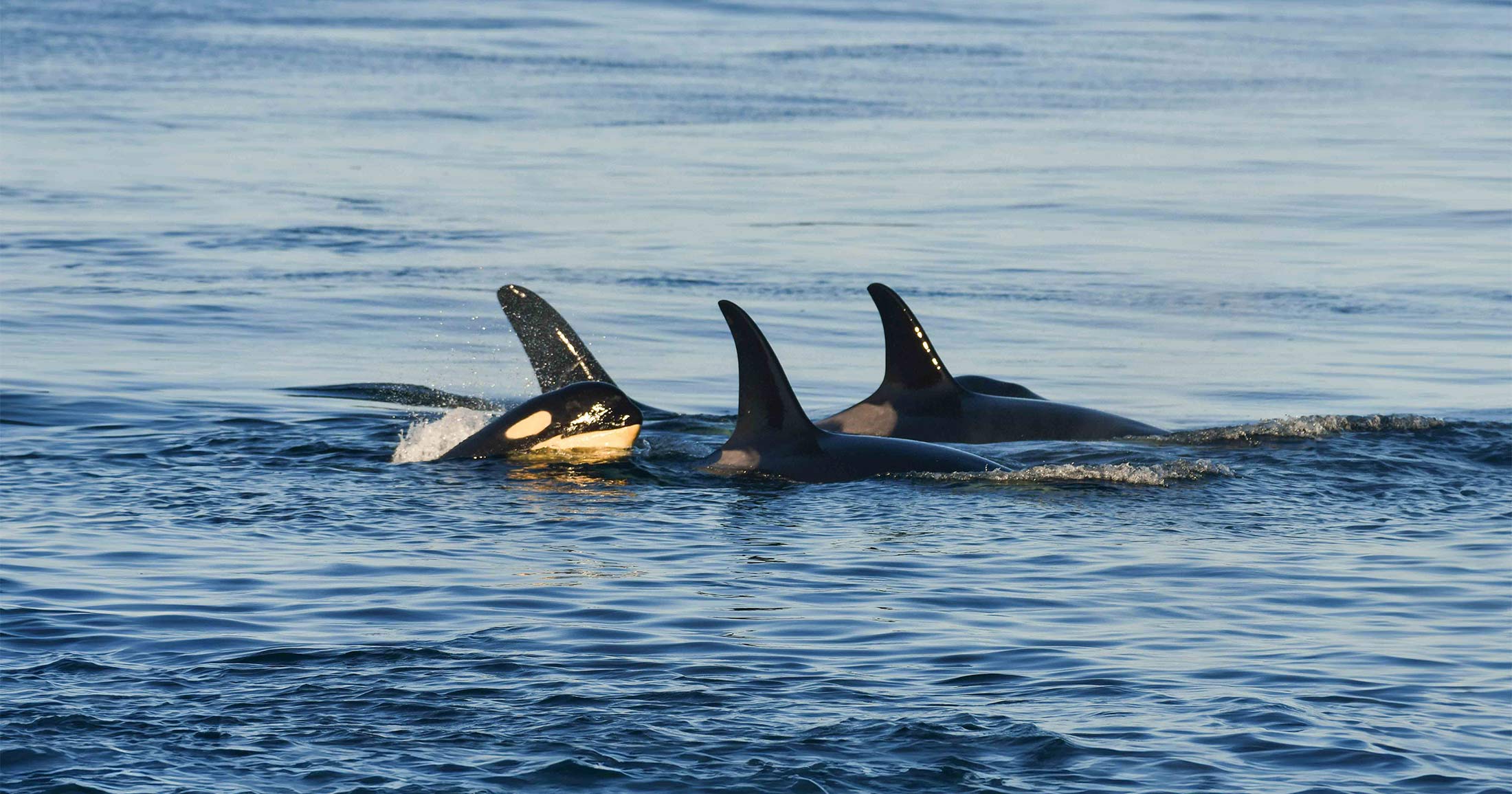
New study coins the term ‘bright extinction’ to describe the failure of Southern Resident killer whales to recover

Learning from the two lives of grad school

Fishy business: the technicalities of fisheries management planning
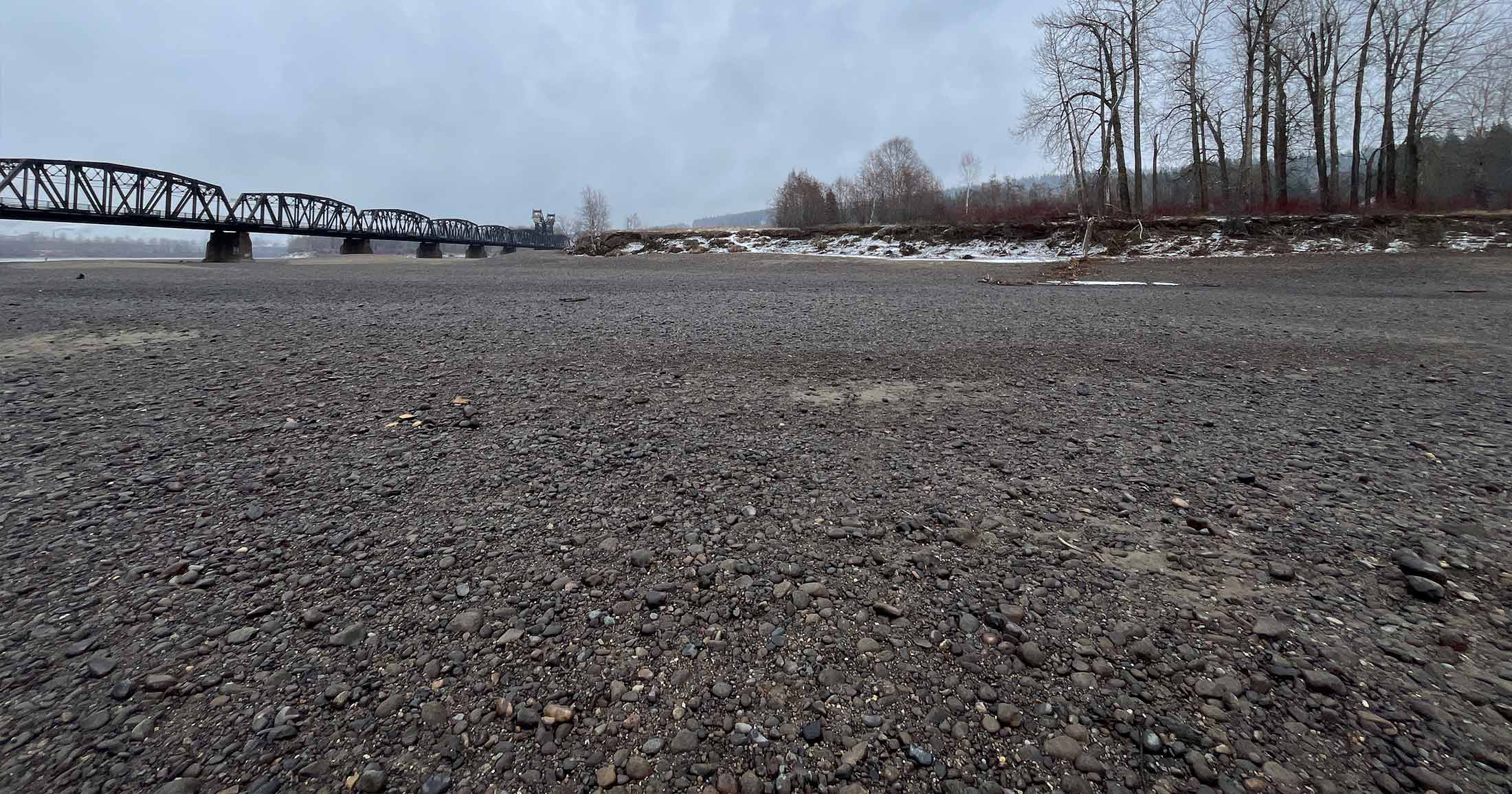
Drought resilience for Nicola River salmon

The federal 2030 National Biodiversity Strategy: another vision document or a legally-binding action plan?
Get connected.

An official website of the United States government
The .gov means it’s official. Federal government websites often end in .gov or .mil. Before sharing sensitive information, make sure you’re on a federal government site.
The site is secure. The https:// ensures that you are connecting to the official website and that any information you provide is encrypted and transmitted securely.
- Publications
- Account settings
Preview improvements coming to the PMC website in October 2024. Learn More or Try it out now .
- Advanced Search
- Journal List

Coexistence or conflict: Black bear habitat use along an urban-wildland gradient
Joanna klees van bommel.
1 Department of Forest Resources Management, University of British Columbia, Vancouver, British Columbia, Canada
Catherine Sun
Adam t. ford.
2 Department of Biology, University of British Columbia Okanagan, Kelowna, British Columbia, Canada
Melissa Todd
3 British Columbia Ministry of Forests, Coast Area Research Section, Nanaimo, British Columbia, Canada
A. Cole Burton
4 Biodiversity Research Centre, University of British Columbia, Vancouver, British Columbia, Canada
Associated Data
The data are available from the Dryad database ( https://doi.org/10.5061/dryad.nvx0k6dvf ).
The urban-wildland interface is expanding and increasing the risk of human-wildlife conflict. Some wildlife species adapt to or avoid living near people, while others select for anthropogenic resources and are thus more prone to conflict. To promote human-wildlife coexistence, wildlife and land managers need to understand how conflict relates to habitat and resource use in the urban-wildland interface. We investigated black bear ( Ursus americanus ) habitat use across a gradient of human disturbance in a North American hotspot of human-black bear conflict. We used camera traps to monitor bear activity from July 2018 to July 2019, and compared bear habitat use to environmental and anthropogenic variables and spatiotemporal probabilities of conflict. Bears predominantly used areas of high vegetation productivity and increased their nocturnality near people. Still, bears used more high-conflict areas in summer and autumn, specifically rural lands with ripe crops. Our results suggest that bears are generally modifying their behaviours in the urban-wildland interface through spatial and temporal avoidance of humans, which may facilitate coexistence. However, conflict still occurs, especially in autumn when hyperphagia and peak crop availability attract bears to abundant rural food resources. To improve conflict mitigation practices, we recommend targeting seasonal rural attractants with pre-emptive fruit picking, bear-proof compost containment, and other forms of behavioural deterrence. By combining camera-trap monitoring of a large carnivore along an anthropogenic gradient with conflict mapping, we provide a framework for evidence-based improvements in human-wildlife coexistence.
Introduction
The urban-wildland interface, where human development borders natural habitat, is increasing with an expanding human footprint [ 1 , 2 ]. This has important consequences for wildlife; some animals are displaced by habitat loss due to direct and adjacent human development, while others are able to adjust their behaviours to use human areas [ 3 ]. For instance, some species have been found to reduce movement [ 4 ] and increase nocturnal activity [ 5 ] when using areas dominated by people. These behavioural changes may function to reduce direct contact with diurnal human activity [ 6 ]. For example, tigers ( Panthera tigris ) and grizzly bears ( Ursus arctos ) have been shown to shift to nocturnal behaviour near people to minimize the risk of conflict and mortality [ 6 , 7 ]. Such behavioural changes that decrease negative interactions may lead to coexistence, where humans and wildlife share landscapes with sustainable risks of death, injury, or significant cost to either party [ 8 ].
For human communities adjacent to natural (hereafter “wild”) habitats, it is important to know whether local wildlife are adjusting to a range of human influences in a manner that can promote coexistence. Behavioural changes to avoid direct conflict may actually increase indirect conflicts due to attempts by animals to access calorie-rich human food attractants (e.g., accessing garbage, fruit, or crops at night when interaction with people is less likely). For example, increased nocturnality of cougars ( Puma concolor ) in human-dominated landscapes has been shown to increase their movement and caloric expenditure, and ultimately their potential to pursue anthropogenic attractants such as livestock [ 9 ]. Consequently, behavioural changes such as increased nocturnality could lead to either coexistence or conflict depending on context and conditions at the urban-wildlife interface.
Anthropogenic food attractants are a common source of human-wildlife conflict [ 1 ]. Attractants like garbage can appear beneficial as they are predictable, consistent, and spatially aggregated sources of possibly high-caloric food, thus requiring less foraging effort [ 10 ]. For potentially dangerous animals like large carnivores, their use of such attractants can provoke management responses that typically involve relocating or destroying the animal [ 11 , 12 ]. Higher mortality in areas of greater conflict compared to wild areas with low or no conflict creates an ecological trap, whereby an animal selects the area based on its resources but experiences unexpectedly high mortality and lowered fitness [ 13 ]. These ecological traps function as population sinks which have negative impacts on long-term population persistence [ 14 ], and may become more common as the urban-wildland interface increases.
Coexistence with carnivores in the expanding urban-wildlife interface requires human mitigation to reduce wildlife visitation rates and mortality risks associated with human-dominated areas. Strategies include protecting anthropogenic food attractants or creating a hostile environment to signal the mortality risks to wildlife in human-dominated areas. For instance, if securing livestock indoors is not possible, setting up an electric fence to deter carnivores can reduce conflict [ 15 ]. Such actions may promote behavioural changes in carnivores to reduce conflict or maintain behaviours consistent with coexistence. Understanding the effectiveness of mitigation efforts and whether new strategies are needed requires knowledge about how carnivores use habitats and resources within the urban-wildland interface, and how that relates to the probability of conflict.
Black bears ( Ursus americanus ) often occupy urban-wildland interfaces in North America and have been found to change their natural behaviours as a result of human disturbance (including human presence and development) [ 10 , 16 ]. They are a forest-adapted species with generalist diets and can alter their foraging patterns to access new food sources [ 10 , 12 , 17 ]. In the urban-wildland interface, omnivorous bears may select for anthropogenic over wild food sources [ 12 ], preying on livestock and consuming garbage, compost, bird feed, and fruit. The high caloric value and availability of anthropogenic food is especially attractive in the summer and autumn when bears need to store energy for hibernation, and can result in seasonal increases in human-bear conflicts [ 11 , 18 ]. If such attractants remain constant and available, they can draw animals from surrounding wild areas and create a reliance on anthropogenic food, which may change bear behaviour in the urban-wildland interface. In Nevada, urban black bears are less active each day (as they satisfied their caloric needs faster), more nocturnal, and hibernate less [ 10 ]. Evidence suggests that some bears do not hibernate at all in moderate climates with continuous food sources like garbage in the winter [ 19 ].
Previous research on black bear habitat use has found that bears typically avoid people, selecting forested habitat close to edges and at low elevations, where they can access vegetation in meadows and riparian areas [ 20 , 21 ]. But by preferring edges, black bears are primed to thrive in the urban-wildland interface, as rural and suburban development creates more edge habitat which may increase natural foods like berries [ 22 ] and bring in new anthropogenic food attractants. On southern Vancouver Island in British Columbia, Klees van Bommel et al. [ 18 ] found that human-black bear conflict probability increased where suburban development adjoined wild areas, and in places with intermediate human density; but in autumn, high-conflict probability expanded into rural/agricultural areas, when reports of human-bear conflict shifted from being driven primarily by garbage attractants to include fruit, compost, and other rural attractants. It is thus likely that seasonal conditions affect the attractiveness of different types of human areas for bears, as is seen with the increase of rural conflicts during summer and autumn when crops ripen. Therefore, it is important to study bear habitat use both spatially and temporally to understand the implications for conflict.
Study objectives
We aimed to understand the spatial and temporal dynamics of black bear habitat use along a gradient of human disturbance, from urban to wild, in the Capital Regional District of Vancouver Island, British Columbia (BC), Canada—a region reporting hundreds of human-black bear conflicts per year [ 18 ]. We conducted a camera trap study, and estimated habitat use by bears in urban, rural, and wild areas, as defined by human density and land use type. We measured and modelled the spatial distribution of black bear camera trap detections as a function of environmental and anthropogenic variables over a full year. To assess the relationship between habitat use and conflict, we subsequently modeled detections using previously estimated seasonal conflict probabilities from the same region [ 18 ]. Finally, we tested if bears were more active at night in human-dominated urban and rural landscapes compared to wild areas.
We tested two contrasting hypotheses to explain patterns of bear habitat use relative to reported human-bear conflict. Our “conflict hypothesis” posited that bear habitat use was driven primarily by available anthropogenic foods, such that bears would be most active in rural areas (i.e., the interface between urban and wild) where they had access to a broader spectrum of human attractants, such as garbage and bird feeders, but also unique attractants like livestock and food crops in addition to wild resources. Under this hypothesis, bears would not be expected to modify their behaviour to avoid conflict, such that habitat use would have a positive relationship with conflict probability and the degree of nocturnality would be similar in urban versus wild areas. An alternative “coexistence hypothesis” posited that bears changed their behaviour in the interface to avoid humans and thus avoid areas of high conflict probability, due to perceived costs (e.g. increased mortality risk) outweighing the potential benefits of anthropogenic resources [ 5 , 10 ]. Under this hypothesis, we predicted that the areas of greatest habitat use by bears would be those with a low conflict probability, and that bears would be more nocturnal in urban and rural areas compared to wild areas.
Vancouver Island, BC, Canada, is home to black bears living at high densities near urban areas. Recent bear population estimates for Vancouver Island are not available, however high bear abundance is indicated by some of the highest average annual harvest densities across BC during the past ten years (up to 25 bears/100 km 2 ) [ 23 ]. The municipality of Sooke, on the southern tip of Vancouver Island, has a current human census of approximately 13,000 that forms part of Victoria’s Capital Regional District (CRD) population of 383,360 [ 24 ]. Sooke is the second fastest growing of 13 municipalities in the CRD, with growth rapidly converting forest into housing developments. The forested landscape around Sooke is comprised of second growth dry coastal western hemlock forests with Douglas-fir as the leading tree species, berry-producing shrub understories, and productive salmon rivers [ 25 ]. Between 2011 and 2017, Sooke had 60% of the reported human-bear conflicts in the CRD, and more than twice the number of calls to conservation officers than other municipalities. In that same timeframe, Sooke also had the highest conflict-related mortality for black bears, accounting for 38 of the 60 bear deaths reported in the CRD [British Columbia Conservation Officer Service, unpublished data ].
Camera traps
We set 54 camera traps within an 80 km 2 area in and adjacent to Sooke to assess spatial and temporal variation in bear distribution and habitat use along a gradient of human disturbance from urban to wild ( Fig 1 ). Camera traps are an established non-invasive tool for monitoring large terrestrial animals with minimal human influence on behaviour, and are effective with clear research objectives [ 26 , 27 ]. Based on estimated female black bear home ranges on Vancouver Island (7.83 km 2 ) [ 22 ], we expected the study area to include multiple overlapping home ranges, capturing a representative sample of the local bear population.

Location of 54 camera traps used to sample black bear habitat use as measured by camera trap detection rates in and around the municipality of Sooke on Vancouver Island, BC, Canada. Other vegetation includes shrubs, herbs, wetlands, and shorelines outside of urban or agricultural land uses.
We deployed cameras following a stratified random design [ 28 ] to representatively allocate cameras based on the proportion of the survey area falling within each of three strata: urban (n = 11 cameras), rural (n = 19), or wild (n = 24). We aimed for >200 m between neighboring camera sites (mean = 446 m, range = 147–1467 m) to increase spatial independence [ 29 ]. Within strata, sampling distribution was randomized where possible. Due to the abundance of private land, urban and rural camera sites were selected from a candidate list of participating landowners provided by the local environmental non-governmental organization, Wild Wise Sooke. Rural sites were either within agricultural land cover or low development areas, while urban sites were in town and close to other homes. Wild sites were in forested areas with minimal disturbance from human development, consisting of 21 in Sea to Sea Regional Park and three on undeveloped T’Sou-ke Nation lands. Work was completed under a CRD Regional Parks park use permit (#15/19) and signed consent to entry forms from all private landowners and T’Sou-ke Nation. To randomize sampling locations within the main accessible block of the regional park, a 500 by 500 m grid was overlaid on park trail maps and cameras were placed in 10 random cells that contained a trail. The T’Sou-ke Nation forest sites and regional park sites on the northwest edge were only accessible by a single hiking trail, so cameras were set a minimum of 200 m apart. To avoid excessive human photos and privacy concerns, we avoided setting cameras directly on the main hiking trails in the park and T’Sou-ke Nation lands, and either targeted adjacent game and low-use human trails within the selected cell or set cameras off the main trail. Deployment occurred between July 18—August 20, 2018. To detect any seasonal variation in black bear habitat use [ 22 ], all cameras remained deployed for approximately one year, and were retrieved between July 16–19, 2019. We used a combination of three camera trap models (Reconyx PC900, Reconyx HC600, and Browning Strike Force HD Pro) randomly allocated across strata to reduce potential effects of different detectability between camera models.
We set cameras at locations to maximize the probability of detecting bears that occurred there, using local knowledge of where bears moved across urban or rural properties, or the presence of animal trails and sign. Per site, one camera was set on a tree, approximately one metre above the ground, at high sensitivity, with a one second delay between triggers (one image per trigger as bears are large enough to be captured without a sequence and this saves battery and memory card space) [ 30 ], and facing open spaces such as meadows, lawns, or trails. Black bears have shown a preference for using low-use human paths because of the ease of movement and increased shrub vegetation containing berries [ 22 , 31 ]. Where possible, cameras faced an intersection of multiple animal and/or low-use human trails.
We visited camera traps every 2–3 months to download images, check functionality and replace batteries as needed. We used Timelapse Image Analyzer 2.0 [ 32 ] to classify all camera trap images of black bears. We defined independent detection events as those separated by ≥30 minutes to minimize correlation among consecutive detections as individual bears were not uniquely identifiable [ 27 ]. We counted sows with cubs as single individuals because sows determined the habitat use. We summed the number of detection events at each camera site for each month to calculate the monthly camera trap detection rate as a measure of habitat use (13 months x 54 cameras).
Habitat use modelling framework
We used zero-inflated generalized linear mixed modelling (GLMM) to model bear habitat use. Our dependent variable was habitat use as measured by the monthly, camera-level detection rate of bears (i.e, count of independent detections). We chose to directly model detection rates instead of commonly proposed occupancy models as estimates from the latter may not be reliable for species with relatively large home ranges compared to the spacing of sampling points [ 33 ]. We used a zero-inflated approach in order to accommodate the high proportion of zeros (80%) due to some cameras not detecting bears in each month or at all [ 34 ]. To control for non-independence from repeat bear detections at a site across months, we included a random intercept for camera site in all models. All models also included the number of active camera days as an offset to account for variable sampling effort (i.e. not all cameras were active for all study days). Models were run with the ‘ glmmTMB’ package [ 35 ] in Program R [ 36 ]. We used the negative binomial distribution “Nbinom2” which treats the variance quadratically because all candidate models had a lower AICc compared to the “Nbinom1” distribution (where variance was treated linearly) [ 35 ].
Habitat use predictor variables
To relate bear detections to environmental and anthropogenic features associated with conflict probability, we considered a suite of camera-specific independent variables extracted from spatial datasets. We included human density, trail density, road density, elevation, and distances to agriculture and urban land cover ( S1 and S2 Tables), averaged within a 150 m radius weighted buffer centred on camera locations in order to avoid overlapping buffers. These are the same predictors and buffers used in previous research to model human-black bear conflict in the CRD [ 18 ] to allow for direct comparison of their importance in explaining reported conflicts at the regional scale (CRD) versus bear habitat use at the local scale (Sooke). For variables derived from GIS raster layers with cells that extended beyond the buffer boundary, values were proportionally weighted to the cell areas within the buffer. Additionally, we used the Enhanced Vegetation Index (EVI) as a measure of vegetation productivity to indicate forage availability, rather than distance-to-forest because all camera locations were set within treed areas. We extracted EVI from MODIS 250m 16-day layers [ 37 ]. Unlike other common vegetation indices, EVI does not saturate in high biomass areas like forests, remaining sensitive to variation [ 38 ] and therefore informative across the forested sites. EVI has been used as a proxy for fruit abundance (grapes, Vitis spp .) in rural areas as ripeness peaks at the same time as greenness [ 39 ]. EVI may also serve as a proxy for season as greenness is highest in productive spring and summer months. We used a weighted average based on number of days the 16-day MODIS window had within our focal calendar month of analysis and the amount each raster cell fell into a 150m buffer around each camera site.
Additional predictors captured local-scale variation in natural food occurrence and recent conflict reports. We used distance-to-freshwater as a proxy for the documented importance of riparian vegetation and fish for black bears [ 40 ]; presence/absence of salmon at camera sites near (within 150 m buffer radius) salmon-bearing water by month [Charters Creek Hatchery, unpublished data ], given their importance as a seasonal food resource for bears [ 22 ]; and the number of reported conflicts within a 500 m buffer of a camera site within the study year [ 41 ]. The buffer size for the latter two additional variables were tested at 150 and 500 m as in Klees van Bommel et al. [ 18 ].
We prepared continuous predictor variables by standardizing them, and ensuring noncollinearity (r < 0.7) [ 42 ]. Standardization by subtracting the mean and dividing by 1 standard deviation allowed for direct comparison of estimated effect sizes on bear habitat use. We computed the correlation matrix using Pearson correlation. We also tested for non-independence in terms of spatial autocorrelation in the residuals from best-supported models (see below) using Moran’s I test [ 43 ].
Habitat use candidate models
We specified a set of candidate models as competing hypotheses to explain spatial and temporal variation in black bear habitat use ( Table 1 ). We expected if our overall “conflict hypothesis” was supported, the best fit model would show selection for human-dominated areas with high values for variables associated with high conflict probability; selection for wild areas with high values for low conflict probability variables, would support our “coexistence hypothesis”. We tested four sets of candidate models to see which types of predictor variables best explained bear habitat use. The first model (“conflict”) tested if bears were selecting areas with conditions that increased conflict probability, using the same predictors as Klees van Bommel et al. [ 18 ] used to model regional-scale on human-black bear conflict in the same study area, but applied to the local camera scale, with EVI to represent forest cover and vegetation productivity as a proxy for food and cover. The second model (“anthropogenic”) tested if bears selected areas based primarily on human attractants and disturbance, by considering only anthropogenic variables and the recent conflict reports. A third model (“environmental”) tested if bears were using areas based on natural food occurrence and security cover, by considering only environmental variables, including the distance-to-freshwater and presence of salmon variables. A fourth model (“full”) was a full model with all conflict, anthropogenic and environmental predictor variables. The final model was a null model. We also included a quadratic term for human density in all candidate models with anthropogenic variables, to allow for bear selection of areas of intermediate density (i.e. rural areas), as well as an interaction between human density and trail density to test if bears used (or avoided) trails to navigate through human-dominated areas.
Candidate models for black bear habitat use as measured by monthly camera trap detection rates, from 54 camera traps sampled in and around Sooke, BC, Canada from July 2018 –July 2019 using zero-inflated GLMMs. Models are grouped by candidate set, with sub-models representing variations in variables (quadratic terms or interactions) included if they are within 2 ΔAICc of the best model in the set. Evaluated predictor variables extracted from a 150 m buffer around camera locations include HD = human density, RD = road density, EVI = enhanced vegetation index, DUrb = distance-to-urban, DAg = distance-to-agriculture, Con = conflict (500 m buffer), DW = distance-to-freshwater, Sal = salmon, Ele = elevation, and TD = trail density. All models also have site as a random effect and number of active days as an offset. Df is the degrees of freedom of the model, within ΔAICc is the difference in AICc scores from the top model within a set, between ΔAICc is the difference in top models between sets, Akaike weight is the relative likelihood of a model divided by the sum of those values across all models.
We used Akaike’s Information Criterion corrected for small sample sizes (AICc) to assess statistical support among candidate models and selected the model with the lowest AICc. We also calculated the Akaike weight, which is the relative likelihood of a model divided by the sum of those values across all models [ 44 ], using “ICtab” from R package bbmle [ 45 ].
Habitat use vs. seasonal conflict probability
To test if black bear habitat use had a positive relationship with conflict probability, and thus test predictions from a regional model of conflict at a local scale, we also modeled camera trap detections using the predicted spatially explicit probabilities of human-black bear conflict estimated from Klees van Bommel et al. [ 18 ]. Given that black bear habitat use is expected to vary seasonally following food availability, we used seasonal conflict probability and modelled an interaction with season (spring: February-April, summer: May-July, autumn: August-October, and winter: November-January). The months covered by each season have been adjusted to allow autumn to include the period of greatest berry abundance [ 22 ] and the timing of hyperphagia. Other predictor variables included season and strata (urban, wild, and rural).
We considered a set of 8 candidate models, which included each predictor modelled individually, 4 models in which season and strata were either additive or interaction terms with seasonal conflict probability, and a final null model ( Table 2 ). Seasons were modelled as a factor, with spring used as the intercept. All models included site as a random effect and the number of active camera days as an offset to account for variable sampling effort. Each model was run using zero-inflation and used either the “Nbinom1” and “Nbinom2” variance structure–whichever resulted in a lower AICc. We used AICc to compare all models due to small sample sizes.
Candidate models for the relationship of seasonal black bear conflict probabilities with black bear habitat use as measured by monthly camera trap detection rates, from 54 camera traps sampled in and around Sooke, BC, Canada from July 2018 –July 2019 using zero-inflated GLMMs. Evaluated predictor variables include seasonal conflict probability extracted from 150 m buffer around camera locations from Klees van Bommel et al. [ 18 ]; seasons defined as spring: February-April, summer: May-July, autumn: August-October, and winter: November-January; and strata depending on where camera traps were set: urban, rural, or wild sites. All models also have site as a random effect and number of active days as an offset. Each model was run twice, using negative binomial 1 or 2 (nb1, nb2), and the model with the lower AICc is included below. Df is the degrees of freedom of the model, ΔAICc is the difference in AICc scores from the top model.
Nocturnality
To test if Sooke black bears exhibited increased nocturnality in human areas as a potential indicator of coexistence behaviour, we compared the proportion of nocturnal bear activity between areas of high (urban and rural sites) vs. low disturbance (wild sites) [ 5 ]. We first classified all independent bear detections as either diurnal (between sunrise and sunset) or nocturnal (between sunset and sunrise), using the R package suncalc [ 46 ]. We then calculated risk ratios (RR) and associated variance for the urban and rural categories as
where X h is percent nocturnal activity (i.e., the proportion of night detections out of all detections) at high disturbance (e.g, urban or rural), X 1 is percent nocturnal activity at low disturbance (e.g., wild), and O is the number of observations [ 5 ]. RR > 0 would suggest a greater degree of nocturnality in response to a greater human presence, with larger numbers representing greater nocturnality, whereas a RR < 0 would indicate lower nocturnality in response to increased human presence. We assessed support for changes in nocturnality by comparing the observed RR values to a bootstrapped distribution of RR values, which was created by randomly assigning detections into urban/rural/wild categories 1000 times and calculating RR values. We considered changes in nocturnality to be significant if the 95% confidence intervals (CIs) of observed RRs did not overlap with the 95% highest posterior density intervals (HPDIs) of the bootstrapped RR distributions. We used the “HDInterval” [ 47 ] package in R.
Camera traps detected black bears in all 13 months of the survey (N = 548 independent detections over 16,546 camera trap days), averaging 42 monthly detections across all sites, with the most detections in September 2018 (n = 148) and the least in February and March of 2019 (n = 1 each; Fig 2 providing evidence that not all bears hibernate). Rural sites had the most detections (n = 368), followed by wild and urban sites with 103 and 77 detections respectively ( S4 Table ) [ 48 ]. While individual bears are not reliably distinguishable from camera trap images, we did photograph multiple bears of different sizes at the same sites, sows with cubs of different sizes taken around the same date, and multiple photos taken close in time at different sites that were unlikely to be the same bear.

Monthly black bear detection rate from 54 camera traps sampled July 2018–2019 in Sooke, BC, Canada grouped seasonally. The detection rate accounts for time when camera traps were not active, with rate = (number of detections / number of active camera days) * number of days in the month. Seasons are spring: February-April, summer: May-July, autumn: August-October, and winter: November-January.
Patterns of habitat use by black bears in Sooke were best explained by the “conflict model”, which included anthropogenic and environmental variables that explained broader patterns of conflict in the CRD (Akaike weight: 0.41; Table 1 , full model set S3 Table ). However, the direction of effects did not fully match the predictions of either our “conflict” or “coexistence” hypotheses. Bears were more active in areas with greater EVI and higher vegetation productivity (0.34 ± 0.13, p < 0.01; 95% confidence intervals do not overlap zero, Fig 3 ), which was low-conflict parks and crown land forest in winter and spring, but high-conflict rural areas in summer and autumn ( S1 Fig ). We failed to detect an effect of human density (effect size: -2.37 ± 1.85 standard error, p = 0.20; quadratic term: -0.49 ± 0.25, p = 0.05), road density (-0.22 ± 0.24, p = 0.34), elevation (-0.52 ± 0.27, p = 0.06), distance-to-urban (0.17 ± 0.21, p = 0.41) and -agriculture (-0.12 ± 0.30, p = 0.68), or trail density (-2.31 ± 1.24, p = 0.06). While the “conflict model” was > 2 ΔAICc from the next hypothesis model set (the full model), a version within the “conflict model” set that did not include the interaction between trail and human density was only 0.6 ΔAICc away, suggesting this interaction did not add much to the explanatory power of the top model (-3.54 ± 2.25, p = 0.12). There was no evidence of spatial autocorrelation in the model residuals (Moran I = -0.052, p-value = 0.999).

Estimated effects of human and environmental variables on black bear habitat use as measured by camera trap detection rate in Sooke, BC, Canada. Variables are human, road, and trail density (including a quadratic variable for human density and interaction between human and trail density), distance-to-agriculture and -urban, enhanced vegetation index, elevation, and camera trap active days. Coefficients from best-supported zero-inflated negative binomial generalized linear mixed model of monthly detections from 54 camera traps sampled July 2018–2019 illustrated as mean and 95% confidence intervals. Predictor variables have been standardized to a mean of zero and standard deviation of one to allow for direct comparison.
Bear habitat use in the seasonal conflict probability model set was best explained by the model with an interaction between seasonal conflict probability and season ( Table 2 ). Monthly bear detection rates ( Fig 2 ) were significantly higher in summer (effect size: 1.37 ± 0.52 standard error, S.E., p < 0.01) and autumn (1.12 ± 0.47, p = 0.02) compared to spring, while winter detections were similar to spring (-0.27 ± 0.52, p = 0.60; Fig 4 ). Bear detections were generally lower in areas with increasing conflict probability (-0.22 ± 0.11, p = 0.05), but less so in summer (0.18 ± 0.10, p = 0.09) and winter (0.22 ± 0.11, p = 0.04) compared to spring due to the interaction between conflict probability and season. By contrast, detections of bears in autumn were higher in areas with higher conflict probability (0.22 ± 0.11, p = 0.04; Figs Figs4 4 and and5 5 ).

Estimated effects of seasonal conflict probability and season on black bear habitat use as measured by camera trap detection rate in Sooke, BC, Canada. Coefficients from best-supported zero-inflated negative binomial generalized linear mixed model of monthly detections from 54 camera traps sampled July 2018–2019 illustrated as mean and 95% confidence intervals. Seasons are spring: February-April, summer: May-July, autumn: August-October, and winter: November-January. Predictor variables have been standardized to a mean of zero and standard deviation of one to allow for direct comparison.

Plot of relationship between monthly black bear camera trap detections and the interaction between seasonal conflict probability and season (spring: February-April, summer: May-July, autumn: August-October, and winter: November-January) represented by mean and 95% confidence intervals.
Consistent with the prediction of our “coexistence hypothesis”, the risk ratios for nocturnal activity in urban versus wild areas (RR urban = 0.84; 95% CI: 0.42–1.26) and rural versus wild areas (RR rural = 0.74; 95% CI: 0.37–1.12) were both positive and did not overlap the bootstrapped 95% HPDIs (urban: -0.36–0.32; rural: -0.21–0.27); Fig 6 , S4 Table ), suggesting greater nocturnality in areas of higher human disturbance. Small sample sizes prevented estimation of season-specific risk ratios.

Expected null distribution versus observed risk ratios for black bears nocturnality from urban and rural camera trap sites relative to wild sites. The risk ratio (RR) compares nighttime activity for bears detected in areas of high human disturbance (X h , urban = blue, rural = yellow) with those in low disturbance (X l, wild) areas using the equation Risk Ratio = ln(X h /X l ), where 0 would mean no difference. Expected distribution was calculated using 1000 bootstrap iterations from data. Horizontal lines show the 95% Highest Posterior Density Intervals for the expected (null) distribution (solid lines) and 95% confidence intervals for the observed values (dashed lines).
Our results suggest that black bears in Sooke change their behaviours in response to human presence. The municipality of Sooke has the highest reported rates of human-bear conflict and conflict-related bear mortality on Vancouver Island, which is already known for having one of the highest rates of human-bear interactions in North America [ 49 ]. Therefore, the Sooke area represents an opportunity to investigate whether bears are changing their behaviours in response to humans in ways that either facilitate coexistence or result in conflict with people. Our results showed that the conflict model best fit the camera detection data, with vegetative condition significantly influencing bear habitat use. Furthermore, the relationship between camera trap detections and previously modelled probabilities of conflict shows that bears are shifting their habitat use spatially by season and increasing their nocturnal activity in human spaces.
Our results suggest, however, that bear responses to conditions in the urban-wildland interface are not consistent year-round and do not support simple interpretations of conflict versus coexistence. Increased nocturnality in urban and rural spaces compared to wild areas and preference for vegetatively productive habitats (high EVI) suggest coexistence for much of the year, but increased use of high-conflict rural areas still occurs in autumn. This is similar to observations of seasonal shifts in natural diets before hyperphagia, where bears select swamp habitat with an abundance of berries, grasses, and willows [ 50 ]. We suggest that autumn conflict reflects the bears’ hyperphagia to prepare for winter denning, coupled with the peak availability (ripeness) of anthropogenic crops, led to riskier bear behaviour.
Spatially, bears used habitats with higher EVI, suggesting they were selecting coniferous forest for shelter and natural foods in winter and spring [ 20 , 21 ]. This could be a result of bears avoiding people as predicted by our “coexistence hypothesis”, which may thereby minimize conflicts. While, our model results did not find a significant effect of human density on habitat use, previous studies in the North Cascades in Washington (USA), and Michigan (USA), found that black bears avoided people based on metrics of developed lands and roads [ 20 , 51 ]. Interestingly, road density and distances to urban and agricultural areas were also not significant predictors in our study, while edge habitats (i.e., those close to disturbed areas) have been found to be selected by bears in the Cascade Mountains, USA [ 21 ]. The lack of a similar effect in our study may have resulted from the 150 m buffer size we extracted our variables at, or suggest that bears in Sooke select habitat based on food availability and avoidance of direct human encounters rather than general landscape condition. Future research should further evaluate the importance of avoiding direct human encounters relative to responding to habitat changes such as landscape disturbance and supplemental human food resources for bears in Sooke.
Bear activity was significantly more nocturnal in urban and rural areas than at wild sites, further suggesting they were avoiding direct interactions with humans and possibly facilitating human-wildlife coexistence [ 5 ]. However, nocturnal activity could increase a bear’s ability to access food attractants like garbage, compost, or livestock, which may be less guarded by people at night. This is supported by research from Colorado which found that bears became more nocturnal in years of poor natural food availability, as they used areas of higher human density to access anthropogenic food sources [ 52 ]. Furthermore, nighttime activity in bears has been found to peak in spring, when food is scarce, and in autumn, when energetic requirements are high, further suggesting that increased nocturnality is an adjustment to facilitate access to human resources [ 53 ]. Therefore, despite the change to nocturnal behaviour, conflicts could still be detrimental to bear fitness if they cause property damage property that results in reactive management of conflict bears.
Overall, bear avoidance of humans in the Sooke study area fosters coexistence in the urban-wildland interface for most of the year, with notable exceptions in the late summer and fall when a combination of hyperphagia and increased availability of high-quality anthropogenic food resources likely increase bear tolerance of human presence and attract bears into rural conflict. Consistent with our “conflict hypothesis” bears avoided high conflict areas less in summer (May-July) and even used them more in autumn (August-October). At these times of year, high conflict areas are largely human-dominated rural areas, which have the broadest spectrum of anthropogenic food attractants available. If our assumption that higher EVI indicates forage availability and crop ripeness is correct, as has been shown in other studies [ 12 , 38 , 39 ], Sooke bears may be selecting for crops, orchards, and berries along forest edges that provide important, abundant, and aggregated foods during hyperphagia. Fruit especially has been found to be selected over natural and other anthropogenic attractants by black bears in Nevada [ 12 ] and grizzly bears in Canada [ 13 ]. Bears may then be drawn into human areas as they are attracted to fruits and crops coming into season. Indeed, the conflict probability model showed that bear detections increased in autumn despite increasing conflict probability. Therefore, bear habitat use appears to reflect a spatiotemporal shift made to balance increased caloric needs with risk of conflict.
We do note that our second set of models comparing habitat use to seasonal conflict probability did not propagate the uncertainty from the models used to estimate the conflict probabilities. Future research could further integrate reports of human-black bear conflict with camera trap surveys to more accurately predict and target where and when conflicts may happen, as in Fidino et al. [ 54 ]. Conflict reports themselves are a sample of all the conflict that occurs, and thus contain error as some conflict goes unreported. However, community demographics have been found to have limited influence on the chance of reporting conflicts, and conflicts relating to safety or property damage (which encompasses many human-black bear conflicts) are more likely to be reported [ 18 ]. We therefore assumed that sampling of conflicts across Sooke was not systematically biased.
Current mitigation efforts may not be enough to promote coexistence by reducing attractants or increasing bears’ perceived risk of human areas. While the spike of rural conflicts in autumn may result from fruit and other rural attractants, garbage conflicts are the focus of current mitigation priorities because they are the most common type of human-bear conflict year-round in Sooke [ 55 ]. We recommend that conflict mitigation strategies in Sooke should extend beyond urban and rural garbage management to include managing autumn attractants, such as by picking fruit in a timely manner and keeping attractants such as compost or crops within electric fences or other bear proof containers.
In addition to mitigation, land managers should also prioritize conserving or restoring natural food sources. Black bears have been found to use human food attractants more when there was a natural food shortage, and then revert back in subsequent years as natural foods recovered [ 52 ]. Protecting natural resources may gain importance as climate change affects the range, abundance, and timing of foods like berries [ 56 ] and salmon [ 57 ].
Our study provides a foundation for future research on human-bear interactions in this and similar hotspots of conflict. Useful next steps would be to estimate bear densities in the urban-wildland interface [ 58 ], and monitor trends over time in comparison to wild regions. Furthermore, to determine if Sooke is an ecological trap for bears, tracking individual movements could determine if bears are drawn to urban spaces from wilder areas, and whether conflict-related deaths are affecting the broader population, or if a few “problem” individuals are responsible for the majority of conflicts [ 13 ]. If bears are attracted to urban areas, determining the sexes of those individuals would be important as females of reproductive age have a greater impact on population demography. In general, male black bears have been found to use more developed areas and thus be involved in more conflict [ 12 , 16 ], however in poor natural food years, females may select areas of higher development than males [ 59 ]. Extending the sampling period of the current design while adding finer scale data on anthropogenic and natural bear foods–particularly salmon and berries–could also provide information on how dependent these bear conflict behaviours are on natural food availability, and whether natural or anthropogenic foods are supporting bears who do not stay in dens over winter.
Coexistence, as defined by Carter and Linnell [ 8 ], occurs when humans and wildlife share landscapes without unsustainable risk of death, injury, or significant cost to either party. As the urban-wildland interface expands, coexistence will require both humans and wildlife to adapt to avoid conflict. The current combination of black bear use of low-conflict, forested habitats for most of the year, and their increased nocturnal behaviour in areas of higher human density, represents behavioural plasticity via spatial and temporal avoidance of humans, thereby reducing the chance of dangerous conflicts and contributing to human-bear coexistence [ 6 ]. However, bear use of urban areas and their attraction to rural areas in summer and autumn suggest that bears are not entirely avoiding risks associated with food-related conflicts. As long as seasonal rural conflicts continue, bears may be targeted for lethal mitigation or become food-conditioned and desensitized to humans, and thus be at risk of escalating dangerous conflicts. Thus, coexistence with bears and other large carnivores necessitates some human tolerance of conflict risk, with an aim of reducing that risk to an acceptable level through mitigation strategies and practices [ 8 ]. Mitigations will vary by species and socio-ecological context; our study shows how research can determine the patterns of space use by wildlife in human-dominated areas and relate them to conflict outcomes to generate context-specific management recommendations.
Supporting information
Predictor variables used to model black bear habitat use in Sooke, Vancouver Island, Canada, between 2018–2019. Variables all derived within 150 m radius buffers around camera trap locations unless otherwise noted. Weighted buffers reduce the contribution of raster layer cells not fully within the circular buffer by the percent excluded.
Mean values of predictor variables by strata used to model black bear habitat use in Sooke, Vancouver Island, Canada, between 2018–2019.
All candidate for black bear habitat use as measured by monthly camera trap detection rates, from 54 camera traps sampled in and around Sooke, BC, Canada from July 2018 –July 2019 using zero-inflated GLMMs. Evaluated predictor variables extracted from a 150m buffer around camera locations include HD = human density, RD = road density, EVI = enhanced vegetation index, DUrb = distance-to-urban, DAg = distance-to-agriculture, Con = conflict (500 m buffer), DW = distance-to-freshwater, Sal = salmon, Ele = elevation, and TD = trail density. All models also have site as a random effect and number of active days as an offset. Df is the degrees of freedom of the model, within ΔAICc is the difference in AICc scores from the top model within a set, between ΔAICc is the difference in top models between sets, Akaike weight is the relative likelihood of a model divided by the sum of those values across all models.
Number of independent black bear detections in the day versus night at urban, rural, and wild camera trap sites (n = 548).
Enhanced Vegetation Index (EVI) averaged within sampling strata (urban, rural, or wild) across 54 camera-trap sites in Sooke, BC, Canada sampled from July 2018–2019.
Acknowledgments
We thank Peter Arcese and Garth Mowat for feedback on earlier drafts, Wild Wise Sooke for assistance finding land owners to work with, T’Sou-ke Nation for insight and allowing us to set cameras on their land, the District of Sooke for access to GIS data, and Mike Badry and the British Columbia Conservation Officer Service for access to their conflict reporting data. We thank Todd Golumbia, Joanna Burgar, Jacqui Sunderland-Groves, Erin Tattersall, Aisha Uduman, Alexia Constantinou, Taylor Justason, Meghna Bandyopadhyay, and Paige Monteiro for field assistance, and Emily Siemens, Lauren Kasper, Zach Brunton, Avril Hann, Micaela Anguita, HyunGu Kang and Isla Francis for help processing photos. We also thank our reviewers Stijn Verschueren and Mason Fidino for their constructive suggestions.
Funding Statement
Funding was received from a National Geographic Early Career Grant (EC-336R-18; https://www.nationalgeographic.org/society/grants-and-investments/ ) awarded to JKvB, the University of British Columbia’s Faculty of Forestry (no grant number; https://forestry.ubc.ca/ ), and the Natural Sciences and Engineering Research Council of Canada (NSERC; https://www.nserc-crsng.gc.ca/index_eng.asp ), through a Canada Graduate Scholarship to JKvB (no grant number) and NSERC Discovery Grant (DGECR-2018-00413) to ACB. The funders had no role in study design, data collection and analysis, decision to publish, or preparation of the manuscript.
Data Availability
- PLoS One. 2022; 17(11): e0276448.
Decision Letter 0
PONE-D-22-12898Coexistence or conflict: black bear habitat use along an urban-wildland gradientPLOS ONE
Dear Dr. Klees van Bommel,
Thank you for submitting your manuscript to PLOS ONE. After careful consideration, we feel that it has merit but does not fully meet PLOS ONE’s publication criteria as it currently stands. Therefore, we invite you to submit a revised version of the manuscript that addresses the points raised during the review process.
Please submit your revised manuscript by Aug 18 2022 11:59PM. If you will need more time than this to complete your revisions, please reply to this message or contact the journal office at gro.solp@enosolp . When you're ready to submit your revision, log on to https://www.editorialmanager.com/pone/ and select the 'Submissions Needing Revision' folder to locate your manuscript file.
Please include the following items when submitting your revised manuscript:
- A rebuttal letter that responds to each point raised by the academic editor and reviewer(s). You should upload this letter as a separate file labeled 'Response to Reviewers'.
- A marked-up copy of your manuscript that highlights changes made to the original version. You should upload this as a separate file labeled 'Revised Manuscript with Track Changes'.
- An unmarked version of your revised paper without tracked changes. You should upload this as a separate file labeled 'Manuscript'.
If you would like to make changes to your financial disclosure, please include your updated statement in your cover letter. Guidelines for resubmitting your figure files are available below the reviewer comments at the end of this letter.
If applicable, we recommend that you deposit your laboratory protocols in protocols.io to enhance the reproducibility of your results. Protocols.io assigns your protocol its own identifier (DOI) so that it can be cited independently in the future. For instructions see: https://journals.plos.org/plosone/s/submission-guidelines#loc-laboratory-protocols . Additionally, PLOS ONE offers an option for publishing peer-reviewed Lab Protocol articles, which describe protocols hosted on protocols.io. Read more information on sharing protocols at https://plos.org/protocols?utm_medium=editorial-email&utm_source=authorletters&utm_campaign=protocols .
We look forward to receiving your revised manuscript.
Kind regards,
Bogdan Cristescu
Academic Editor
Academic Editor's (Bogdan Cristescu) comments:
Two reviewers provided excellent comments and suggestions, with the feedback from one of them resembling more of a major than minor revision. Please address their points in the revised manuscript and response to reviewers document.
It will be important to justify why an occupancy modeling approach was not used. One reviewer provided suggestions on some ways to achieve that.
Please include metrics of model fit throughout, for example in Tables 1 and 2.
Looking forward to seeing the revision.
Journal Requirements:
When submitting your revision, we need you to address these additional requirements.
1. Please ensure that your manuscript meets PLOS ONE's style requirements, including those for file naming. The PLOS ONE style templates can be found at
https://journals.plos.org/plosone/s/file?id=wjVg/PLOSOne_formatting_sample_main_body.pdf and
https://journals.plos.org/plosone/s/file?id=ba62/PLOSOne_formatting_sample_title_authors_affiliations.pdf
2. In your Methods section, please provide additional information regarding the permits you obtained for the work. Please ensure you have included the full name of the authority that approved the field site access and, if no permits were required, a brief statement explaining why.
3. In your Data Availability statement, you have not specified where the minimal data set underlying the results described in your manuscript can be found. PLOS defines a study's minimal data set as the underlying data used to reach the conclusions drawn in the manuscript and any additional data required to replicate the reported study findings in their entirety. All PLOS journals require that the minimal data set be made fully available. For more information about our data policy, please see http://journals.plos.org/plosone/s/data-availability .
"Upon re-submitting your revised manuscript, please upload your study’s minimal underlying data set as either Supporting Information files or to a stable, public repository and include the relevant URLs, DOIs, or accession numbers within your revised cover letter. For a list of acceptable repositories, please see http://journals.plos.org/plosone/s/data-availability#loc-recommended-repositories . Any potentially identifying patient information must be fully anonymized.
Important: If there are ethical or legal restrictions to sharing your data publicly, please explain these restrictions in detail. Please see our guidelines for more information on what we consider unacceptable restrictions to publicly sharing data: http://journals.plos.org/plosone/s/data-availability#loc-unacceptable-data-access-restrictions . Note that it is not acceptable for the authors to be the sole named individuals responsible for ensuring data access.
We will update your Data Availability statement to reflect the information you provide in your cover letter.
4. We note that you have stated that you will provide repository information for your data at acceptance. Should your manuscript be accepted for publication, we will hold it until you provide the relevant accession numbers or DOIs necessary to access your data. If you wish to make changes to your Data Availability statement, please describe these changes in your cover letter and we will update your Data Availability statement to reflect the information you provide.
5. We note that Figure 1 in your submission contain [map/satellite] images which may be copyrighted. All PLOS content is published under the Creative Commons Attribution License (CC BY 4.0), which means that the manuscript, images, and Supporting Information files will be freely available online, and any third party is permitted to access, download, copy, distribute, and use these materials in any way, even commercially, with proper attribution. For these reasons, we cannot publish previously copyrighted maps or satellite images created using proprietary data, such as Google software (Google Maps, Street View, and Earth). For more information, see our copyright guidelines: http://journals.plos.org/plosone/s/licenses-and-copyright .
We require you to either (1) present written permission from the copyright holder to publish these figures specifically under the CC BY 4.0 license, or (2) remove the figures from your submission:
a. You may seek permission from the original copyright holder of Figure 1 to publish the content specifically under the CC BY 4.0 license.
We recommend that you contact the original copyright holder with the Content Permission Form ( http://journals.plos.org/plosone/s/file?id=7c09/content-permission-form.pdf ) and the following text:
“I request permission for the open-access journal PLOS ONE to publish XXX under the Creative Commons Attribution License (CCAL) CC BY 4.0 ( http://creativecommons.org/licenses/by/4.0/ ). Please be aware that this license allows unrestricted use and distribution, even commercially, by third parties. Please reply and provide explicit written permission to publish XXX under a CC BY license and complete the attached form.”
Please upload the completed Content Permission Form or other proof of granted permissions as an "Other" file with your submission.
In the figure caption of the copyrighted figure, please include the following text: “Reprinted from [ref] under a CC BY license, with permission from [name of publisher], original copyright [original copyright year].”
b. If you are unable to obtain permission from the original copyright holder to publish these figures under the CC BY 4.0 license or if the copyright holder’s requirements are incompatible with the CC BY 4.0 license, please either i) remove the figure or ii) supply a replacement figure that complies with the CC BY 4.0 license. Please check copyright information on all replacement figures and update the figure caption with source information. If applicable, please specify in the figure caption text when a figure is similar but not identical to the original image and is therefore for illustrative purposes only.
The following resources for replacing copyrighted map figures may be helpful:
USGS National Map Viewer (public domain): http://viewer.nationalmap.gov/viewer/
The Gateway to Astronaut Photography of Earth (public domain): http://eol.jsc.nasa.gov/sseop/clickmap/
Maps at the CIA (public domain): https://www.cia.gov/library/publications/the-world-factbook/index.html and https://www.cia.gov/library/publications/cia-maps-publications/index.html
NASA Earth Observatory (public domain): http://earthobservatory.nasa.gov/
Landsat: http://landsat.visibleearth.nasa.gov/
USGS EROS (Earth Resources Observatory and Science (EROS) Center) (public domain): http://eros.usgs.gov/#
Natural Earth (public domain): http://www.naturalearthdata.com
6. Please review your reference list to ensure that it is complete and correct. If you have cited papers that have been retracted, please include the rationale for doing so in the manuscript text, or remove these references and replace them with relevant current references. Any changes to the reference list should be mentioned in the rebuttal letter that accompanies your revised manuscript. If you need to cite a retracted article, indicate the article’s retracted status in the References list and also include a citation and full reference for the retraction notice.
[Note: HTML markup is below. Please do not edit.]
Reviewers' comments:
Reviewer's Responses to Questions
Comments to the Author
1. Is the manuscript technically sound, and do the data support the conclusions?
The manuscript must describe a technically sound piece of scientific research with data that supports the conclusions. Experiments must have been conducted rigorously, with appropriate controls, replication, and sample sizes. The conclusions must be drawn appropriately based on the data presented.
Reviewer #1: Yes
Reviewer #2: Yes
2. Has the statistical analysis been performed appropriately and rigorously?
Reviewer #2: No
3. Have the authors made all data underlying the findings in their manuscript fully available?
The PLOS Data policy requires authors to make all data underlying the findings described in their manuscript fully available without restriction, with rare exception (please refer to the Data Availability Statement in the manuscript PDF file). The data should be provided as part of the manuscript or its supporting information, or deposited to a public repository. For example, in addition to summary statistics, the data points behind means, medians and variance measures should be available. If there are restrictions on publicly sharing data—e.g. participant privacy or use of data from a third party—those must be specified.
Reviewer #1: No
4. Is the manuscript presented in an intelligible fashion and written in standard English?
PLOS ONE does not copyedit accepted manuscripts, so the language in submitted articles must be clear, correct, and unambiguous. Any typographical or grammatical errors should be corrected at revision, so please note any specific errors here.
5. Review Comments to the Author
Please use the space provided to explain your answers to the questions above. You may also include additional comments for the author, including concerns about dual publication, research ethics, or publication ethics. (Please upload your review as an attachment if it exceeds 20,000 characters)
Reviewer #1: The authors analyze camera detection rates of black bears in and around a conflict hotspot. Urban-wildlife interfaces are expanding rapidly and understanding habitat use of key species in human environments is critical for their management. The manuscript is well-written, the objectives are clearly defined and addressed, and the findings are discussed within a relevant context. I have few comments:
The authors acknowledge complexities with individual bear identification and discuss future directions of research that include density estimates and individual movements. Is there however any indication that multiple and enough individuals have been captured during your study to be representative for the broader population. In particular because generalist species typically display a great amount of behavioral plasticity, and the extent of the study area is rather small (~80km2).
Related to above comment and a potential avenue for future research, would there be any (expected) differences in habitat use between adult males, adult females, and females with cubs? And how would this influence conflict or coexistence?
How did you deal with the fact that not detecting a bear at a camera trap location may be a false absence? And consequently the zero’s in the zero inflated model may not all be true absences, which may induce bias in inferences on habitat use?
L388-393: It is not clear to me on what base you make these inferences. You report, for example, a negative association between bear habitat use and human density, but no effects of trail density are reported. From looking at Fig3, it seems however that both variables have similar coefficient estimates, standard errors (not overlapping zero) and confidence intervals (overlapping zero). The same applies for the quadratic term of human density and elevation. I would therefore think that only ‘Active Days’ and ‘EVI’ are informative predictors of bear habitat use.
L414-418: Please be consistent in reporting p-values and could you include these in the previous results section?
L416: maybe add ‘compared to spring’.
L460-463 & L469-471: Is there any previous research in natural systems that shows seasonal shifts in habitat use as a consequence of hyperphagia?
Reviewer #2: # Review for:
*Coexistence or conflict: black bear habitat use along an urban-wildland gradient*
Note: This was written in markdown format. I've also included a PDF if that is easier for you to read.
In this paper the authors look at spatio-temporal variation in the distribution of black bears in an area where conflict between black bears and humans is high. Additionally, they also look at the correlation between black bear detections and the probability of black-bear conflict that was estimated from previous research. In general, I think this is an interesting and well written piece of research. I often found myself in agreement with the logical flow of the research, the modeling choices made, and the interpretation of said models. Great work!
Perhaps my biggest concern is the secondary modeling approach, which treats the estimated conflict probability as known, when in fact it is something that is estimated with error. In my review below I have a number of hopefully helpful citations about this specific issue for the authors.
I also suggest the authors look at a recent article of mine (Fidino et al. 2022), which shows how integrated models could be use to combine human-wildlife conflict data with camera trap data. I'm not on the hunt for a citation here, but since it is relevant to this piece of research I am sharing in case you were interested in how to do this in the future.
Fidino, M., Lehrer, E. W., Kay, C. A., Yarmey, N. T., Murray, M. H., Fake, K., ... & Magle, S. B. (2022). Integrated species distribution models reveal spatiotemporal patterns of human–wildlife conflict. Ecological Applications, e2647.
If you have any specific questions about any of my comments, feel free to email me at gro.oozpl@onidifm . Again, great work on this!
- Mason Fidino
## Abstract
### Line by line comments
Line 46: The 'such as' breaks up this sentence in a weird way. I think you can remove it and the examples become a little more clear.
## Introduction
### Top-level thoughts
1. Well written introduction, I only have some minor comments on some of the wording / sentence structure.
Line 64: Is sustainable the correct word here? I think it could be, but also it tends to have a bit of a positive connotation to it, so it reads a little off to say sustainable risks of death. Maybe 'acceptable' could be used instead? Just a minor point, feel free to ignore if you disagree.
Line 69: This sentence is a little circular. Changes to avoid direct conflict can increase direct conflict when risk of direct conflict is lower. I think it's the second use of direct conflict, which makes it feel a little redundant. Could that bit right after the first (e.g.,) just get removed? I think the point still comes across.
Line 76-77: Is 'year round' needed when you already state they are available consistently? "...as they are predictable, consistent, and spatially aggregated sources of..."
Line 82-84: Does it help here to remind the reader that the urban-wildlife interface is increases, and therefore increased risk of ecological traps for such species?
Line 88: ...to signal wildlife mortality risks...
Line 98: ...and can alter their foraging patterns to...
Line 103: ..., and can result in seasonal...
Line 103-105: Sentence starting with "If such attractants" reads a little weird. Maybe it's the 'attractants can attract animals' part or that the '...and food-conditioning' bit at the end feels like a different thought tacked on.
Line 105 - 108: This sentence could be made more active (and therefore a little more succinct). "In Nevada, urban black bears are less active each day (as they satisfied their caloric needs faster), more nocturnal, and hibernate less."
Line 116-120: Conflict probability of what? Do you mean human-wildlife conflict probability in general? Human-bear conflict in particular?
Line 123-125: Great point, and the introduction sets a really strong foundation for the need to study this.
1. I would imagine that the distance between camera trapping locations would be species specific to maintain spatial independence. For black bear, multiple camera traps can be contained within the home range of a single bear, and therefore I would assume that spatial independence is not met. This citation is also a pre-print, though it's been around for 12 years. Maybe just soften the language here (e.g,. say to increase spatial independence rather than maintain spatial independence).
2. Was the sampling effort term included as an offset to the model or did you just include it as a covariate? If the latter was done, I would encourage adding a log offset term instead, as that is a more standard approach when there is variation in sampling effort. If I had to guess, you used a log-offset, so just be specific about that here in the methods.
3. I could see some readers get their hackles up about not using an occupancy model here. I leave it to the authors, but it may help to just get ahead of it here (by using a zero-inflated model you may be estimating habitat use conditional on presence anyways, depending on the class of zero-inflated model you fit). Likewise, occupancy models are literally just zero-inflated logistic regression, so your approach has substantial overlap. For example, the old MacKenzie et al. occupancy modeling book talks about these similarities on about page 135 (i.e., the use of the zero-inflated binomial to model occupancy).
MacKenzie, D. I., Nichols, J. D., Royle, J. A., Pollock, K. H., Bailey, L. L., & Hines, J. E. (2017). Occupancy estimation and modeling: inferring patterns and dynamics of species occurrence. Elsevier.
4. There are a few different kinds of zero-inflated glmms, can you be a little more specific? For example, what is the error distribution that was used (negative binomial, Poisson, etc.). Is this a two part model and therefore the conditional model cannot include zeroes or is it a mixture model and therefore the conditional models can include zero? A little more explanation here would be helpful. note: I see now that the distributional information is shared much lower, around line 297. I'd move that little bit of info upwards so it's not separated from when you introduce the modeling framework.
5. Thank you for using a set of candidate models to assess your different hypotheses. Solid approach. Given the two set hypotheses brought up in the introduction, in may help to add a little more connection between those hypotheses and the set of candidate models given that there are two hypotheses but four candidate models. You should also fit a fifth null model as well (just the active days term plus the site random effect). Given the results I suspect the null will provide the worst fit (highest delta AIC), but it's nice to demonstrate this.
6. Based on the introduction (lines 133-134) I thought that modeled conflict probabilities would be incorporated into your glmms, but instead it looks like counts of conflict are included instead. I suspect other readers will also be confused about this. What makes this more confusing, is that there is a second batch of models done that uses the estimated conflict probabilities.
7. Why conflicts over the year instead of conflicts per month?
8. Using the output from one model as a predictor in another is okay, but the uncertainty of those estimates should also be propagated into the secondary model. From my reading of this secondary model set, I'm guessing that these spatially explicit probabilities are treated as known (i.e., measured without error). If this is the case, using such predictions in secondary analysis leads to anticonservative tests because this error is excluded from further tests (i.e., estimates are too precise). Some papers about this topic that the authors may find useful include:
Hadfield, J. D., Wilson, A. J., Garant, D., Sheldon, B. C., & Kruuk, L. E. (2010). The
misuse of BLUP in ecology and evolution. The American Naturalist, 175(1), 116-125.
Houslay, T. M., & Wilson, A. J. (2017). Avoiding the misuse of BLUP in behavioural
ecology. Behavioral Ecology, 28(4), 948-952.
Link, W. A. (1999). Modeling pattern in collections of parameters. The Journal of
wildlife management, 1017-1027.
The Houslay & Wilson paper is open access, so that is where I'd start. I've personally found this easiest to account for in a Bayesian framework (e.g., if you have the mean and SE of each prediction you can set a prior for each data point to propagate that uncertainty), but there are likely ways to deal with this in a frequentist framework as well (e.g., bootstrapping, but resampling the predicted covariate instead of the response variable).
9. What is a reported conflict in these data? Do they vary in severity?
1. Failing to detect an effect does not mean that there was no effect (e.g., line 391 - 392). I'd just reword to "We failed to detect an effect of road density" so that you avoid confirming the null (which these tests do not do). Other than that, great breakdown of the results.
## Discussion
1. Given the conflicting hypotheses, do the authors feel that one hypothesis was supported more than the other?
2. Any caveats worth bringing up here? For example, there was the assumption that EVI indicates forage availability. Is it possible for there to be human-bear conflicts that go unreported and so the conflicts / year metric used may have some error?
Line 530: You used ecological trap earlier.
## Tables & figures
1. The axis text on many of the figures is a very light gray, I'd suggest replacing with black to make it easier to read.
2. You could increase the line width for the 95% CI's on figure 3&4, plus the mean estimate on figure 5.
6. PLOS authors have the option to publish the peer review history of their article ( what does this mean? ). If published, this will include your full peer review and any attached files.
If you choose “no”, your identity will remain anonymous but your review may still be made public.
Do you want your identity to be public for this peer review? For information about this choice, including consent withdrawal, please see our Privacy Policy .
Reviewer #1: Yes: Stijn Verschueren
Reviewer #2: Yes: Mason Fidino
[NOTE: If reviewer comments were submitted as an attachment file, they will be attached to this email and accessible via the submission site. Please log into your account, locate the manuscript record, and check for the action link "View Attachments". If this link does not appear, there are no attachment files.]
While revising your submission, please upload your figure files to the Preflight Analysis and Conversion Engine (PACE) digital diagnostic tool, https://pacev2.apexcovantage.com/ . PACE helps ensure that figures meet PLOS requirements. To use PACE, you must first register as a user. Registration is free. Then, login and navigate to the UPLOAD tab, where you will find detailed instructions on how to use the tool. If you encounter any issues or have any questions when using PACE, please email PLOS at gro.solp@serugif . Please note that Supporting Information files do not need this step.
Submitted filename: PONE-D-22-12898_review.pdf
Author response to Decision Letter 0
14 Sep 2022
Academic Editor's comments:
Two reviewers provided excellent comments and suggestions, with the feedback from one of
them resembling more of a major than minor revision. Please address their points in the
revised manuscript and response to reviewers document.
It will be important to justify why an occupancy modeling approach was not used. One
reviewer provided suggestions on some ways to achieve that.
We appreciate the comments and suggestions from the reviewers and have added their names to our Acknowledgement section [Line 623-624]. We have provided point-by-point responses to the reviewer comments below.
With respect to our reasons for not using occupancy modelling, please see our response to Reviewer #2, Methods question 3 (page 9 below). We have also added metrics of model fit to the Results section to better link the table information to the text.
1. Please ensure that your manuscript meets PLOS ONE's style requirements, including those
for file naming.
We have reviewed the PLOS ONE style requirements and made the necessary changes to the file names.
2. In your Methods section, please provide additional information regarding the permits you
obtained for the work. Please ensure you have included the full name of the authority that
approved the field site access and, if no permits were required, a brief statement explaining
We have added “Work was completed under a CRD Regional Parks park use permit (#15/19) and signed consent to entry forms from all private landowners and T’Sou-ke Nation.” [Lines 198-200]
3. In your Data Availability statement, you have not specified where the minimal data set
underlying the results described in your manuscript can be found. PLOS defines a study's
minimal data set as the underlying data used to reach the conclusions drawn in the manuscript
and any additional data required to replicate the reported study findings in their entirety. All
PLOS journals require that the minimal data set be made fully available. For more information
about our data policy, please see http://journals.plos.org/plosone/s/data-availability .
We have now stored the minimal dataset on Dryad and included the citation “Klees van Bommel J, Sun CC, Ford AT, Todd M, Burton CA. Coexistence or conflict: black bear habitat use along an urban-wildland gradient; 2022 [cited 2022 Aug 18]. Database: Dryad [internet]. doi: 10.5061/dryad.nvx0k6dvf” [48] in our reference list. The dataset is currently listed as private for peer review and can be viewed using the following link: https://datadryad.org/stash/share/LX_zkxl9UuNjMggVpsaA2ukTn7j0646vs3KiBtF45IA
4. We note that you have stated that you will provide repository information for your data at
acceptance. Should your manuscript be accepted for publication, we will hold it until you
provide the relevant accession numbers or DOIs necessary to access your data. If you wish to
make changes to your Data Availability statement, please describe these changes in your cover
letter and we will update your Data Availability statement to reflect the information you
Please refer to the response to number 3 above.
5. We note that Figure 1 in your submission contain [map/satellite] images which may be
copyrighted. All PLOS content is published under the Creative Commons Attribution License
(CC BY 4.0), which means that the manuscript, images, and Supporting Information files will
be freely available online, and any third party is permitted to access, download, copy,
distribute, and use these materials in any way, even commercially, with proper attribution. For
these reasons, we cannot publish previously copyrighted maps or satellite images created
using proprietary data, such as Google software (Google Maps, Street View, and Earth). For
more information, see our copyright guidelines: http://journals.plos.org/plosone/s/licensesand-copyright .
The data used for land cover in Figure 1 is not copyrighted. We initially cited the dataset in our figure legend, but to avoid confusion have removed that line and now only include it in the supplemental table listing data sources as with all of the other environmental and anthropogenic variables used.
6. Please review your reference list to ensure that it is complete and correct. If you have cited
papers that have been retracted, please include the rationale for doing so in the manuscript
text, or remove these references and replace them with relevant current references. Any
changes to the reference list should be mentioned in the rebuttal letter that accompanies your
revised manuscript. If you need to cite a retracted article, indicate the article’s retracted status
in the References list and also include a citation and full reference for the retraction notice.
We have reviewed our reference list, fixed a couple of formatting issues, and found no retracted articles.
Reviewer #1:
The authors analyze camera detection rates of black bears in and around a conflict hotspot. Urban-wildlife interfaces are expanding rapidly and understanding habitat use of key species in human environments is critical for their management. The manuscript is well-written, the objectives are clearly defined and addressed, and the findings are discussed
within a relevant context. I have few comments:
The authors acknowledge complexities with individual bear identification and discuss future
directions of research that include density estimates and individual movements. Is there
however any indication that multiple and enough individuals have been captured during your
study to be representative for the broader population. In particular because generalist species
typically display a great amount of behavioral plasticity, and the extent of the study area is
rather small (~80km2).
We have added the following to the Methods section [Line 178-180]: “Based on estimated female black bear home ranges on Vancouver Island (7.83 km2) [22], we expected the study area to include multiple overlapping home ranges, capturing a representative sample of the local bear population.” Additionally, in the Results we added “While individual bears are not reliably distinguishable from camera trap images, we did photograph multiple bears of different sizes at the same sites, sows with cubs of different sizes taken around the same date, and multiple photos taken close in time at different sites that were unlikely to be the same bear.” to Line 403-406. Therefore, we are confident we captured multiple individuals and that our inferences would extend beyond just a few bears to the broader population.
Related to above comment and a potential avenue for future research, would there be any
(expected) differences in habitat use between adult males, adult females, and females with
cubs? And how would this influence conflict or coexistence?
This is a good point. There is evidence from the literature suggesting that in general male black bears use areas of human development more than females and are thus more likely to be involved in human-carnivore conflict (Beckmann and Berger 2003, Merkle et al. 2013). However, one study found that while females used less developed areas in good natural food years, they selected higher development areas than males in poor natural food years (with and without cubs; Johnson et al. 2015). This could have an exacerbated negative effect on black bear populations if natural food sources are scarce and females are involved in more conflict which may lead to fatal mitigation methods. In fact, a population sink was identified in the Lake Tahoe Basin, Nevada, USA, because female bears in urban areas had higher age-specific mortality rates (Beckmann & Lackey 2008).
We have expanded on our suggestion for future research to look at individual variation within a population (and the implications for managing sex-specific conflicts) [Line 590-594]: “If bears are attracted to urban areas, determining the sexes of those individuals would be important as females of reproductive age have a greater impact on population demography. In general, male black bears have been found to use more developed areas and thus be involved in more conflict [12,16], however in poor natural food years, females may select areas of higher development than males [59].”
How did you deal with the fact that not detecting a bear at a camera trap location may be a
false absence? And consequently the zero’s in the zero inflated model may not all be true
absences, which may induce bias in inferences on habitat use?
We respond to this comment in detail below under Reviewer 2’s suggestion to use occupancy models to account for imperfect detection, please see our response under the section “Methods”, question 3 (page 9 below).
L388-393: It is not clear to me on what base you make these inferences. You report, for
example, a negative association between bear habitat use and human density, but no effects of
trail density are reported. From looking at Fig3, it seems however that both variables have
similar coefficient estimates, standard errors (not overlapping zero) and confidence intervals
(overlapping zero). The same applies for the quadratic term of human density and elevation. I
would therefore think that only ‘Active Days’ and ‘EVI’ are informative predictors of bear
habitat use.
We thank the reviewer for catching this discrepancy. We interpret significant effects as those with a 95% confidence interval that does not overlap zero, so have reworded the Results to clarify that EVI is the only significant predictor variable and moved the model results for human density down with the other predictors [Line 422-424], and removed language stating human density was significant from the Abstract [Line 39-40] and Discussion [Line 500; 507; 517-519].
L414-418: Please be consistent in reporting p-values and could you include these in the
previous results section?
We thank the reviewer for catching this error and have included the p-values for the first set of models in the Results section as well [Line 420-431].
We have edited Line 449 to add “compared to spring”.
L460-463 & L469-471: Is there any previous research in natural systems that shows seasonal
shifts in habitat use as a consequence of hyperphagia?
Yes, previous research has found that bears shift to areas of high natural food abundance before denning. We have added text to highlight that similarity on Line 509-511: “This is similar to observations of seasonal shifts in natural diets before hyperphagia, where bears select swamp habitat with an abundance of berries, grasses, and willows [50].”
Reviewer #2:
In this paper the authors look at spatio-temporal variation in the distribution of black bears in
an area where conflict between black bears and humans is high. Additionally, they also look at
the correlation between black bear detections and the probability of black-bear conflict that
was estimated from previous research. In general, I think this is an interesting and well written
piece of research. I often found myself in agreement with the logical flow of the research, the
modeling choices made, and the interpretation of said models. Great work!
Perhaps my biggest concern is the secondary modeling approach, which treats the estimated
conflict probability as known, when in fact it is something that is estimated with error. In my
review below I have a number of hopefully helpful citations about this specific issue for the
I also suggest the authors look at a recent article of mine (Fidino et al. 2022), which shows
how integrated models could be use to combine human-wildlife conflict data with camera trap
data. I'm not on the hunt for a citation here, but since it is relevant to this piece of research I
am sharing in case you were interested in how to do this in the future.
Fidino, M., Lehrer, E. W., Kay, C. A., Yarmey, N. T., Murray, M. H., Fake, K., ... & Magle, S.
B. (2022). Integrated species distribution models reveal spatiotemporal patterns of human–
wildlife conflict. Ecological Applications, e2647.
Thank you for sharing this new paper! We agree this is a promising approach and we have added a citation to it in our recommendations for future research in Line 563-565: “Future research could further integrate reports of human-black bear conflict with camera trap surveys to more accurately predict and target where and when conflicts may happen, as in Fidino et al. [55].”
Line 46: The 'such as' breaks up this sentence in a weird way. I think you can remove it and
the examples become a little more clear.
We have edited Line 46 to removed “such as”.
1. Well written introduction, I only have some minor comments on some of the wording /
sentence structure.
Line 64: Is sustainable the correct word here? I think it could be, but also it tends to have a bit
of a positive connotation to it, so it reads a little off to say sustainable risks of death. Maybe
'acceptable' could be used instead? Just a minor point, feel free to ignore if you disagree.
We can see how with a positive connotation, “sustainable” may be a jarring word to use in this context. However, the definition of coexistence we are referencing in this sentence and using in our paper was defined in the citation Carter & Linnell, 2016 [8], and we believe reflects the understanding that some conflict is unavoidable but should be mitigated to levels that sustain wildlife populations, human health, and livelihoods.
Line 69: This sentence is a little circular. Changes to avoid direct conflict can increase direct
conflict when risk of direct conflict is lower. I think it's the second use of direct conflict,
which makes it feel a little redundant. Could that bit right after the first (e.g.,) just get
removed? I think the point still comes across.
We have changed the sentence to remove the second instance of “direct conflict” and combined the examples in parentheses: “Behavioural changes to avoid direct conflict may actually increase indirect conflicts due to attempts by animals to access calorie-rich human food attractants (e.g., accessing garbage, fruit, or crops at night when interaction with people is less likely).” [Line 68-70]
Line 76-77: Is 'year round' needed when you already state they are available consistently?
"...as they are predictable, consistent, and spatially aggregated sources of..."
We have edited the sentence to remove “…available consistently (e.g. weekly), year-round, and are…” and replaced with: “…predictable, consistent, and…” [Line 76-77].
Line 82-84: Does it help here to remind the reader that the urban-wildlife interface is
increases, and therefore increased risk of ecological traps for such species?
We have edited the sentence from “These ecological traps function as population sinks which have negative impacts on long-term population persistence [14].” to “These ecological traps function as population sinks which have negative impacts on long-term population persistence [14], and may become more common as the urban-wildland interface increases.” [Line 84-85]
Line 88: ...to signal wildlife mortality risks…
The current line “Strategies include protecting anthropogenic food attractants or creating a hostile environment to signal the mortality risks for wildlife in human-dominated areas.” is meant to generalize human-carnivore conflict mitigation strategies that block or modify carnivore behaviour. In the latter case, techniques such as an electric fence (used as an example in the following sentence) provide an unpleasant or painful sensation to signal danger – this signal is directed at the wildlife in question and thus the phrasing “…to signal wildlife mortality risks…” would be unclear. To clarify our language, we have edited the word “for” to “to”, making the sentence read: “… to signal the mortality risks to wildlife…” [Line 89].
Line 98: ...and can alter their foraging patterns to…
We have edited “…the ability to quickly…” to the recommended “…can alter their…” [Line 99].
Line 103: ..., and can result in seasonal…
We have edited “but” to “and” as recommended [Line 104].
Line 103-105: Sentence starting with "If such attractants" reads a little weird. Maybe it's the
'attractants can attract animals' part or that the '...and food-conditioning' bit at the end feels like
a different thought tacked on.
We have changed the sentence to highlight the connection between attractant availability, reliance of bears on that food source, and behaviour change as a lead in to the next example: “If such attractants remain constant and available, they can draw animals from surrounding wild areas and create a reliance on anthropogenic food, which may change bear behaviour in the urban-wildland interface. In Nevada…” [Line 104-107].
Line 105 - 108: This sentence could be made more active (and therefore a little more
succinct). "In Nevada, urban black bears are less active each day (as they satisfied their caloric
needs faster), more nocturnal, and hibernate less."
We have replaced the sentence with the recommended text [Line 107-108].
Line 116-120: Conflict probability of what? Do you mean human-wildlife conflict probability
in general? Human-bear conflict in particular?
We have edited to clarify it is “human-black bear” conflict probability [Line 120].
Line 123-125: Great point, and the introduction sets a really strong foundation for the need to
study this.
1. I would imagine that the distance between camera trapping locations would be species
specific to maintain spatial independence. For black bear, multiple camera traps can be
contained within the home range of a single bear, and therefore I would assume that spatial
independence is not met. This citation is also a pre-print, though it's been around for 12 years.
Maybe just soften the language here (e.g,. say to increase spatial independence rather than
maintain spatial independence).
We changed “maintain” to “increase” as suggested [Line 191], and we corrected the error in our citation (it is published in a journal, not a pre-print).
Kays, R., Tilak, S., Kranstauber, B., Jansen, P., Carbone, C., Rowcliffe, M., Fountain, T., Eggert, J., He, Z., 2011. Camera traps as sensor networks for monitoring animal communities. International Journal of Research and Reviews in Wireless Sensor Networks 1, 19-29.
While we agree that individual bears could be detected at multiple camera traps, we are suggesting that detections are statistically independent as measures of habitat use (as evidenced by the lack of spatial autocorrelation in detections). If individual identities were known, a random effect could be used to account for between-individual variation, but that is not possible with our data.
2. Was the sampling effort term included as an offset to the model or did you just include it as
a covariate? If the latter was done, I would encourage adding a log offset term instead, as that
is a more standard approach when there is variation in sampling effort. If I had to guess, you
used a log-offset, so just be specific about that here in the methods.
We have updated the models to include Active Days as an offset instead of as a covariate and subsequently removed Active Days from Figure 3. The model’s results remain the same, with the conflict model still the top model and parameters estimates nearly identical (i.e. only changing by tenths or hundredths of a decimal place). The Methods and Results have been edited to reflect these changes, with text to clarify our modelling approach [Line 240-242]: “All models also included the number of active camera days as an offset to account for variable sampling effort (i.e. not all cameras were active for all study days).” and updated estimates in the Results [Line 416-431], Table 1, and Table S3.
3. I could see some readers get their hackles up about not using an occupancy model here. I
leave it to the authors, but it may help to just get ahead of it here (by using a zero-inflated
model you may be estimating habitat use conditional on presence anyways, depending on the
class of zero-inflated model you fit). Likewise, occupancy models are literally just zeroinflated
logistic regression, so your approach has substantial overlap. For example, the old
MacKenzie et al. occupancy modeling book talks about these similarities on about page 135
(i.e., the use of the zero-inflated binomial to model occupancy).
MacKenzie, D. I., Nichols, J. D., Royle, J. A., Pollock, K. H., Bailey, L. L., & Hines, J. E.
(2017). Occupancy estimation and modeling: inferring patterns and dynamics of species
occurrence. Elsevier.
We appreciate that the use of occupancy models is very popular in the camera trap literature, however we feel that they are often applied uncritically and their reliability is an area of active research. We chose to directly model detection rates as measure of site habitat use, since occupancy models have an unsupported assumption of site closure and treat heterogeneity in detections within (arbitrarily defined) sampling periods as observation error, whereas we think it more reflects the signal of interest. Furthermore, some studies have suggested that estimates from occupancy models may not be reliable, particularly for species with relatively large home ranges relative to the spacing of sampling points (e.g., Neilson et al. 2018). We have added some text to clarify our thinking in manuscript on Line 233-235: “We chose to directly model detection rates instead of commonly proposed occupancy models as estimates from the latter may not be reliable for species with relatively large home ranges compared to the spacing of sampling points [33].”
4. There are a few different kinds of zero-inflated glmms, can you be a little more specific?
For example, what is the error distribution that was used (negative binomial, Poisson, etc.). Is
this a two part model and therefore the conditional model cannot include zeroes or is it a
mixture model and therefore the conditional models can include zero? A little more
explanation here would be helpful. note: I see now that the distributional information is shared
much lower, around line 297. I'd move that little bit of info upwards so it's not separated from
when you introduce the modeling framework.
We moved up the text explaining the negative binomial distribution earlier, as suggested, from Line 294-298 to Line 242-245: “Models were run with the ‘glmmTMB’ package [35] in Program R [36]. We used the negative binomial distribution “Nbinom2” which treats the variance quadratically because all candidate models had a lower AICc compared to the “Nbinom1” distribution (where variance was treated linearly) [35].”
Further, the package glmmTMB runs the zero-inflated model as a mixture model, so the conditional models can include zero. For more information see:
Brooks, M. E., Kristensen, K., van Benthem, K. J., Magnusson, A., Berg, C. W., Nielsen, A., Skaug, H. J., Machler, M., & Bolker, B. M. (2017). glmmTMB balances speed and flexibility among packages for Zero-inflated Generalized Linear Mixed Modeling. The R Journal, 9(2), 378-400. https://doi.org/10.32614/RJ-2017-066
5. Thank you for using a set of candidate models to assess your different hypotheses. Solid
approach. Given the two set hypotheses brought up in the introduction, in may help to add a
little more connection between those hypotheses and the set of candidate models given that
there are two hypotheses but four candidate models. You should also fit a fifth null model as
well (just the active days term plus the site random effect). Given the results I suspect the null
will provide the worst fit (highest delta AIC), but it's nice to demonstrate this.
We have added some clarifying language to the Methods section outlining our habitat use models to connect the four candidate models with the overarching two hypotheses to Line 284-289: “We expected if our overall “conflict hypothesis” was supported, the best fit model would show selection for human-dominated areas with high values for variables associated with high conflict probability; selection for wild areas with high values for low conflict probability variables, would support our “coexistence hypothesis”. We tested four sets of candidate models to see which types of predictor variables best explained bear habitat use.”
We also edited the table caption for Table 1 from “Model sets are grouped by hypothesis…” to “Models are grouped by candidate set…” [Line 306-307].
We did run a null model and have now included mention of that in our Methods [Line 299] as well as Table 1. The reviewer is correct in that the null did have the highest delta AIC (24.1 which is more than 10 dAIC higher than the next model, see Table 1).
6. Based on the introduction (lines 133-134) I thought that modeled conflict probabilities
would be incorporated into your glmms, but instead it looks like counts of conflict are
included instead. I suspect other readers will also be confused about this. What makes this
more confusing, is that there is a second batch of models done that uses the estimated conflict probabilities.
We used counts of conflict reported within the year of camera trapping in the first set of models to incorporate local observations from the same temporal scale as the habitat use dependent variable. For the second set of models, we wanted to test how well the predictions from a regional model built using seven years of previous conflict reports matched the local habitat use results from the first set of models. To clarify, we replaced the word “also” with “subsequently” in Line 137: “To assess the relationship between habitat use and conflict, we subsequently modeled detections using previously estimated seasonal conflict probabilities from the same region [18].”
We have also added some text in the Methods section for the second set of models to state our intention to test the regional conflict probabilities against local habitat use [Line 326-327]: “…, and thus test predictions from a regional model of conflict at a local scale…”.
We used the number of reported conflicts within a 500 m buffer of a camera site within the study year as one of our additional local-scale test variables. We decided to use conflicts per year instead of per month because there were not enough reports per month at most of our sites to include.
8. Using the output from one model as a predictor in another is okay, but the uncertainty of
those estimates should also be propagated into the secondary model. From my reading of this
secondary model set, I'm guessing that these spatially explicit probabilities are treated as
known (i.e., measured without error). If this is the case, using such predictions in secondary
analysis leads to anticonservative tests because this error is excluded from further tests (i.e.,
estimates are too precise). Some papers about this topic that the authors may find useful
The Houslay & Wilson paper is open access, so that is where I'd start. I've personally found
this easiest to account for in a Bayesian framework (e.g., if you have the mean and SE of each
prediction you can set a prior for each data point to propagate that uncertainty), but there are
likely ways to deal with this in a frequentist framework as well (e.g., bootstrapping, but
resampling the predicted covariate instead of the response variable).
Thank you for this reflection and the useful references. We acknowledge that we did not propagate the uncertainty from the previously published conflict models into this secondary modelling in this study, and that this is an important consideration. However, this would require an overhaul of our modelling approach, and the addition of further details of the previous models, that is beyond the scope of our intentions for this manuscript. We anticipate that local conflict managers are more likely to use maps of the mean estimates of predicted conflict probabilities in land use planning, i.e. to delineate relatively higher vs lower predicted probabilities. Our intention was to see if these mean predictions from the previously published regional models corresponded to our estimates of local black bear habitat use, in the context of comparing competing model-based hypotheses, rather than to estimate precise coefficients from this second stage of modelling. Thus, we felt it was reasonable to use the mean predictions as “certain” in the way managers might for planning purposes. However, we have added text to clarify that we did not propagate uncertainty and suggested that future models could better integrate conflict and habitat use (Line 561-565): “We do note that our second set of models comparing habitat use to seasonal conflict probability did not propagate the uncertainty from the models used to estimate the conflict probabilities. Future research could further integrate reports of human-black bear conflict with camera trap surveys to more accurately predict and target where and when conflicts may happen, as in Fidino et al. [55].”
We defined conflicts as any interaction that has a negative impact on either black bears or humans. Common conflicts with bears included accessing garbage and other anthropogenic food sources, property damage, and livestock predation. These conflicts are further explained in Klees van Bommel et al. 2020, but we noted “garbage attractants to include fruit, compost, and other rural attractants” along with the citation on Line 123-124. Livestock predation may be considered greater severity, but we did not attempt to rank conflicts.
1. Failing to detect an effect does not mean that there was no effect (e.g., line 391 - 392). I'd
just reword to "We failed to detect an effect of road density" so that you avoid confirming the
null (which these tests do not do). Other than that, great breakdown of the results.
We edited as recommended [Line 422].
1. Given the conflicting hypotheses, do the authors feel that one hypothesis was supported
more than the other?
We believe that further research is required to strengthen support for one hypothesis over the other. We recommended extending the length of camera trap sampling and the addition of finer scale data on anthropogenic and natural bear foods – particularly salmon and berries – to determine the role that natural food availability plays in bear conflict behaviours [Lines 594-598]. However, given the conflicting support among hypotheses in our study, it is likely, that new hypotheses that explicit address behavioural differences across seasons, sex, and ages should also be tested. We provide such recommendations for future research, as well as the recommendation to test if urban areas are sinks affecting the broader black bear community and if conflicts result from widespread behaviours or are limited to certain “conflict bears”.
2. Any caveats worth bringing up here? For example, there was the assumption that EVI
indicates forage availability. Is it possible for there to be human-bear conflicts that go
unreported and so the conflicts / year metric used may have some error?
Yes, to clarify our assumption the EVI indicated forage availability and crop ripeness, we edited Line 550-553 to read: “If our assumption that higher EVI indicates forage availability and crop ripeness is correct, as has been shown in other studies [12,38,39], Sooke bears may be selecting for…”
We also agree it is important to emphasize that conflict is reported with uncertainty and have included text in Line 565-570: “Conflict reports themselves are a sample of all the conflict that occurs, and thus contain error as some conflict goes unreported. However, community demographics have been found to have limited influence on the chance of reporting conflicts, and conflicts relating to safety or property damage (which encompass many human-black bear conflicts) are more likely to be reported [18]. We therefore assumed that sampling of conflicts across Sooke was not systematically biased.”
We edited “sink” to “trap” [Line 587].
1. The axis text on many of the figures is a very light gray, I'd suggest replacing with black to
make it easier to read.
The axis text is in black, but in some cases the axis label is in a larger font and bolded, so the axis text may appear lighter in comparison. We have increased the font size for the axis text on figures 3-5 to make them clearer.
2. You could increase the line width for the 95% CI's on figure 3&4, plus the mean estimate
on figure 5.
We have doubled the width of the 95% confidence interval lines for Figures 3 and 4, as well as for the mean estimates in Figure 5.
Additional Edits
We have updated the author affiliation for co-author Melissa Todd on Line 15.
Line 132 citation changed from “[British Columbia Conservation Officer Service, unpublished data]” to “[18]” as the results were previously published in Klees van Bommel et al. 2020.
We edited Line 158-164 to clarify the findings of black bear hunting data, from “Vancouver Island, British Columbia, Canada, is home to black bears living at high densities near urban areas. While recent bear population estimates for Vancouver Island are not available, high bear abundance is indicated by some of the highest hunter harvesting rates in the province, increasing from 300 to 700 bears per year since the 1980s with no change in hunter success [23]. The municipality of Sooke, on the southern tip of Vancouver Island, British Columbia, Canada,...” to “Vancouver Island, BC, Canada, is home to black bears living at high densities near urban areas. Recent bear population estimates for Vancouver Island are not available, however high bear abundance is indicated by some of the highest average annual harvest densities across BC during the past ten years (up to 25 bears/100 km2) [23]. The municipality of Sooke, on the southern tip of Vancouver Island,…”
We have further edited our explanations of EVI in a couple locations for improved clarity. Firstly, in Line 262-263 we specified that EVI is a proxy for fruit abundance as greenness peaks at the same time: “EVI has been used as a proxy for fruit abundance (grapes, Vitis spp.) in rural areas as ripeness peaks at the same time as greenness [39].” Secondly, we added “...as a proxy for food and cover.” to Line 293.
We also clarified that we didn’t include counts of conflict in our “conflict” habitat use model like we did in our “anthropogenic” model because we wanted to test if the same predictors from Klees van Bommel et al. 2020 were also the best fit for these data in Line 290-292: “...using the same predictors as Klees van Bommel et al. [18] used to model regional-scale on human-black bear conflict in the same study area, but applied to the local camera scale…”
To clarify why spring is not shown in Fig 4, we added text to the Methods in Line 337-338: “Seasons were modelled as a factor, with spring used as the intercept.”
We added a note that the scale at which we extracted variables may have contributed to many variables having an insignificant impact on habitat use [Line 524-525]: “The lack of a similar effect in our study may have resulted from the 150 m buffer size we extracted our variables at, or…”
Finally, some of these edits were suggested by Garth Mowat who provided comments through our co-author Melissa Todd. We have thus included
Response to Map Copyright Question
1. Please note that PLOS ONE is unable to publish previously copyrighted maps or satellite images, or images created using proprietary data. For these reasons, we cannot publish images generated by software which copyrights their output (such as Google Maps, Street View, and Earth). In order to use these images in your submission, we require explicit permission from the copyright owner to publish the figures under the CC BY 4.0 license.
At this time, please kindly clarify the following regarding Figure 1:
a) Where did the authors obtain the maps, satellite images, basemaps, shapefiles, map data, etc. in Figure 1?
The map was created by author Joanna Klees van Bommel using ArcGIS Pro. The camera locations are data collected by author Joanna Klees van Bommel while setting the camera traps for the duration of the project. The land cover dataset which serves as a basemap and the Parks shapefile were obtained from CRD Regional Parks. The T’Sou-ke Nation Land shapefile was adapted from the “Aboriginal Lands of Canada Legislative Boundaries” dataset by the Government of Canada.
b) Please state whether the map/satellite images have been previously copyrighted to your knowledge.
The map was created by author Joanna Klees van Bommel using ArcGIS Pro. According to their website: “You do not need to obtain permission from Esri to include static maps, whether screen capture or printed, in academic publications, for personal use, or in most use cases that do not involve direct resale or commercial monetization of the map.” https://resources.esri.ca/education-and-research/how-to-cite-arcgis-maps-and-data
The camera locations are data collected by author Joanna Klees van Bommel while setting the camera traps for the duration of the project and are not copyrighted.
The land cover dataset and Parks shapefile were obtained from CRD Regional Parks and are not copyrighted.
The T’Sou-ke Nation Land shapefile was adapted from the “Aboriginal Lands of Canada Legislative Boundaries” dataset licenced under an Open Government Licence – Canada which allows for uses including to “Copy, modify, publish, translate, adapt, distribute or otherwise use the Information in any medium, mode or format for any lawful purpose.” https://open.canada.ca/en/open-government-licence-canada
c) If any of the map/satellite images have been previously copyrighted, we require specific consent from the copyright holder to publish these images in PLOS ONE, under the CC BY 4.0 license. To seek permission from the copyright owner to publish your map figures under the specific Creative Commons Attribution License (CCAL), CC BY 4.0, please contact them with the following text and PLOS ONE Request for Permission form ( http://journals.plos.org/plosone/s/file?id=7c09/content-permission-form.pdf ):
“I request permission for the open-access journal PLOS ONE to publish XXX under the Creative Commons Attribution License (CCAL) CC BY 4.0 ( http://creativecommons.org/licenses/by/4.0/ ). Please be aware that this license allows unrestricted use and distribution, even commercially, by third parties. Please reply and provide explicit written permission to publish XXX under a CC BY license.”
Please upload the granted permission to the manuscript as an other file. In the figure caption of the copyrighted figure, please include the following text: “Republished from [ref] under a CC BY license, with permission from [name of publisher], original copyright [original copyright year].”
Not applicable.
Submitted filename: Response to Reviewers.docx
Decision Letter 1
Coexistence or conflict: black bear habitat use along an urban-wildland gradient
PONE-D-22-12898R1
We’re pleased to inform you that your manuscript has been judged scientifically suitable for publication and will be formally accepted for publication once it meets all outstanding technical requirements.
Within one week, you’ll receive an e-mail detailing the required amendments. When these have been addressed, you’ll receive a formal acceptance letter and your manuscript will be scheduled for publication.
An invoice for payment will follow shortly after the formal acceptance. To ensure an efficient process, please log into Editorial Manager at http://www.editorialmanager.com/pone/ , click the 'Update My Information' link at the top of the page, and double check that your user information is up-to-date. If you have any billing related questions, please contact our Author Billing department directly at gro.solp@gnillibrohtua .
If your institution or institutions have a press office, please notify them about your upcoming paper to help maximize its impact. If they’ll be preparing press materials, please inform our press team as soon as possible -- no later than 48 hours after receiving the formal acceptance. Your manuscript will remain under strict press embargo until 2 pm Eastern Time on the date of publication. For more information, please contact gro.solp@sserpeno .
Additional Editor Comments:
The revisions helped improve clarity and strengthened the manuscript. Congratulations on your paper.
Acceptance letter
18 Nov 2022
Dear Dr. Klees van Bommel:
I'm pleased to inform you that your manuscript has been deemed suitable for publication in PLOS ONE. Congratulations! Your manuscript is now with our production department.
If your institution or institutions have a press office, please let them know about your upcoming paper now to help maximize its impact. If they'll be preparing press materials, please inform our press team within the next 48 hours. Your manuscript will remain under strict press embargo until 2 pm Eastern Time on the date of publication. For more information please contact gro.solp@sserpeno .
If we can help with anything else, please email us at gro.solp@enosolp .
Thank you for submitting your work to PLOS ONE and supporting open access.
PLOS ONE Editorial Office Staff
on behalf of
Dr. Bogdan Cristescu
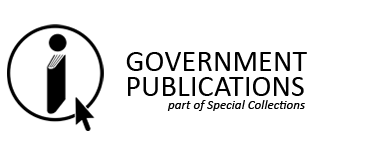
- Special Collections Home
- Databases A-Z
- Digital Collections
- Institutional Repositories
- Find items on the shelf
- Theses & Dissertations
- Subject Guides
- Government by Subject – International and Foreign Countries
- SA Government by Subject
- Useful Guides
- South African Government Links
- Searching the Catalogue
- Referencing Help
- UCT Libraries
- Bolus Herbarium Library
- Brand van Zyl Law Library
- Built Environment Library
- Government Publications
- Bongani Mayosi Health Sciences Library
- Hiddingh Hall Library
- Institute of Child Health Library
- WH Bell Music Library
- Special Collections
- Publication Archive
White Paper on Citizenship, Immigration and Refugee Protection: Towards a complete overhaul of the migration system in South Africa
The Department of Home Affais has published the final White Paper on Citizenship, Immigration and Refugee Protection: Towards a complete overhaul of the migration system in South Africa. "The outcome of the engagements and public comments is that the policy positions adopted in the White Paper enjoy wide support. Only a handful public interest groups are opposed to selected policy positions such as, withdrawal from the 1951 Refugee Convention, the 1967 Protocol and re-acceding with reservations, proposed repeal of section 4 (3) of the South African Citizenship Act and the first safe country principle."
Numbers, Facts and Trends Shaping Your World
Read our research on:
Full Topic List
Regions & Countries
- Publications
- Our Methods
- Short Reads
- Tools & Resources
Read Our Research On:
Gender pay gap in U.S. hasn’t changed much in two decades
The gender gap in pay has remained relatively stable in the United States over the past 20 years or so. In 2022, women earned an average of 82% of what men earned, according to a new Pew Research Center analysis of median hourly earnings of both full- and part-time workers. These results are similar to where the pay gap stood in 2002, when women earned 80% as much as men.
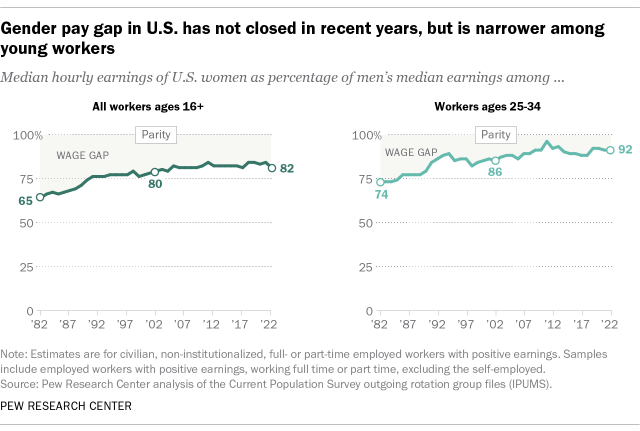
As has long been the case, the wage gap is smaller for workers ages 25 to 34 than for all workers 16 and older. In 2022, women ages 25 to 34 earned an average of 92 cents for every dollar earned by a man in the same age group – an 8-cent gap. By comparison, the gender pay gap among workers of all ages that year was 18 cents.
While the gender pay gap has not changed much in the last two decades, it has narrowed considerably when looking at the longer term, both among all workers ages 16 and older and among those ages 25 to 34. The estimated 18-cent gender pay gap among all workers in 2022 was down from 35 cents in 1982. And the 8-cent gap among workers ages 25 to 34 in 2022 was down from a 26-cent gap four decades earlier.
The gender pay gap measures the difference in median hourly earnings between men and women who work full or part time in the United States. Pew Research Center’s estimate of the pay gap is based on an analysis of Current Population Survey (CPS) monthly outgoing rotation group files ( IPUMS ) from January 1982 to December 2022, combined to create annual files. To understand how we calculate the gender pay gap, read our 2013 post, “How Pew Research Center measured the gender pay gap.”
The COVID-19 outbreak affected data collection efforts by the U.S. government in its surveys, especially in 2020 and 2021, limiting in-person data collection and affecting response rates. It is possible that some measures of economic outcomes and how they vary across demographic groups are affected by these changes in data collection.
In addition to findings about the gender wage gap, this analysis includes information from a Pew Research Center survey about the perceived reasons for the pay gap, as well as the pressures and career goals of U.S. men and women. The survey was conducted among 5,098 adults and includes a subset of questions asked only for 2,048 adults who are employed part time or full time, from Oct. 10-16, 2022. Everyone who took part is a member of the Center’s American Trends Panel (ATP), an online survey panel that is recruited through national, random sampling of residential addresses. This way nearly all U.S. adults have a chance of selection. The survey is weighted to be representative of the U.S. adult population by gender, race, ethnicity, partisan affiliation, education and other categories. Read more about the ATP’s methodology .
Here are the questions used in this analysis, along with responses, and its methodology .
The U.S. Census Bureau has also analyzed the gender pay gap, though its analysis looks only at full-time workers (as opposed to full- and part-time workers). In 2021, full-time, year-round working women earned 84% of what their male counterparts earned, on average, according to the Census Bureau’s most recent analysis.
Much of the gender pay gap has been explained by measurable factors such as educational attainment, occupational segregation and work experience. The narrowing of the gap over the long term is attributable in large part to gains women have made in each of these dimensions.
Related: The Enduring Grip of the Gender Pay Gap
Even though women have increased their presence in higher-paying jobs traditionally dominated by men, such as professional and managerial positions, women as a whole continue to be overrepresented in lower-paying occupations relative to their share of the workforce. This may contribute to gender differences in pay.
Other factors that are difficult to measure, including gender discrimination, may also contribute to the ongoing wage discrepancy.
Perceived reasons for the gender wage gap
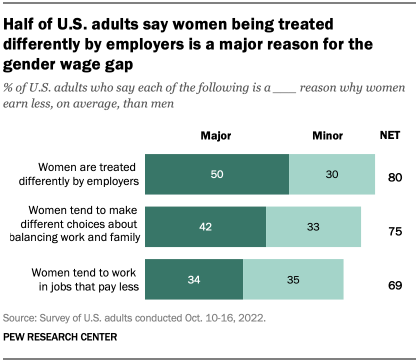
When asked about the factors that may play a role in the gender wage gap, half of U.S. adults point to women being treated differently by employers as a major reason, according to a Pew Research Center survey conducted in October 2022. Smaller shares point to women making different choices about how to balance work and family (42%) and working in jobs that pay less (34%).
There are some notable differences between men and women in views of what’s behind the gender wage gap. Women are much more likely than men (61% vs. 37%) to say a major reason for the gap is that employers treat women differently. And while 45% of women say a major factor is that women make different choices about how to balance work and family, men are slightly less likely to hold that view (40% say this).
Parents with children younger than 18 in the household are more likely than those who don’t have young kids at home (48% vs. 40%) to say a major reason for the pay gap is the choices that women make about how to balance family and work. On this question, differences by parental status are evident among both men and women.
Views about reasons for the gender wage gap also differ by party. About two-thirds of Democrats and Democratic-leaning independents (68%) say a major factor behind wage differences is that employers treat women differently, but far fewer Republicans and Republican leaners (30%) say the same. Conversely, Republicans are more likely than Democrats to say women’s choices about how to balance family and work (50% vs. 36%) and their tendency to work in jobs that pay less (39% vs. 30%) are major reasons why women earn less than men.
Democratic and Republican women are more likely than their male counterparts in the same party to say a major reason for the gender wage gap is that employers treat women differently. About three-quarters of Democratic women (76%) say this, compared with 59% of Democratic men. And while 43% of Republican women say unequal treatment by employers is a major reason for the gender wage gap, just 18% of GOP men share that view.
Pressures facing working women and men
Family caregiving responsibilities bring different pressures for working women and men, and research has shown that being a mother can reduce women’s earnings , while fatherhood can increase men’s earnings .
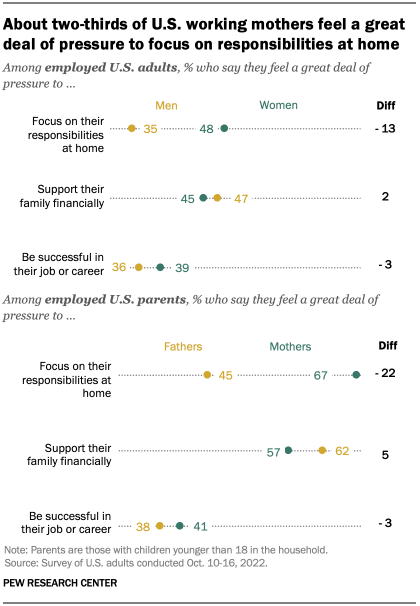
Employed women and men are about equally likely to say they feel a great deal of pressure to support their family financially and to be successful in their jobs and careers, according to the Center’s October survey. But women, and particularly working mothers, are more likely than men to say they feel a great deal of pressure to focus on responsibilities at home.
About half of employed women (48%) report feeling a great deal of pressure to focus on their responsibilities at home, compared with 35% of employed men. Among working mothers with children younger than 18 in the household, two-thirds (67%) say the same, compared with 45% of working dads.
When it comes to supporting their family financially, similar shares of working moms and dads (57% vs. 62%) report they feel a great deal of pressure, but this is driven mainly by the large share of unmarried working mothers who say they feel a great deal of pressure in this regard (77%). Among those who are married, working dads are far more likely than working moms (60% vs. 43%) to say they feel a great deal of pressure to support their family financially. (There were not enough unmarried working fathers in the sample to analyze separately.)
About four-in-ten working parents say they feel a great deal of pressure to be successful at their job or career. These findings don’t differ by gender.
Gender differences in job roles, aspirations
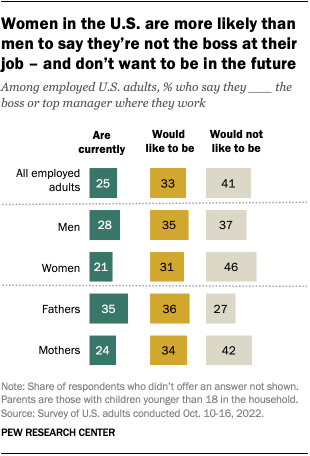
Overall, a quarter of employed U.S. adults say they are currently the boss or one of the top managers where they work, according to the Center’s survey. Another 33% say they are not currently the boss but would like to be in the future, while 41% are not and do not aspire to be the boss or one of the top managers.
Men are more likely than women to be a boss or a top manager where they work (28% vs. 21%). This is especially the case among employed fathers, 35% of whom say they are the boss or one of the top managers where they work. (The varying attitudes between fathers and men without children at least partly reflect differences in marital status and educational attainment between the two groups.)
In addition to being less likely than men to say they are currently the boss or a top manager at work, women are also more likely to say they wouldn’t want to be in this type of position in the future. More than four-in-ten employed women (46%) say this, compared with 37% of men. Similar shares of men (35%) and women (31%) say they are not currently the boss but would like to be one day. These patterns are similar among parents.
Note: This is an update of a post originally published on March 22, 2019. Anna Brown and former Pew Research Center writer/editor Amanda Barroso contributed to an earlier version of this analysis. Here are the questions used in this analysis, along with responses, and its methodology .
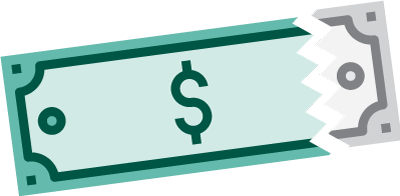
What is the gender wage gap in your metropolitan area? Find out with our pay gap calculator
- Gender & Work
- Gender Equality & Discrimination
- Gender Pay Gap
- Gender Roles
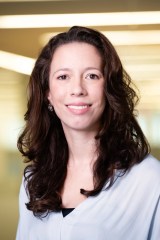
Women have gained ground in the nation’s highest-paying occupations, but still lag behind men
Diversity, equity and inclusion in the workplace, the enduring grip of the gender pay gap, more than twice as many americans support than oppose the #metoo movement, women now outnumber men in the u.s. college-educated labor force, most popular.
1615 L St. NW, Suite 800 Washington, DC 20036 USA (+1) 202-419-4300 | Main (+1) 202-857-8562 | Fax (+1) 202-419-4372 | Media Inquiries
Research Topics
- Age & Generations
- Coronavirus (COVID-19)
- Economy & Work
- Family & Relationships
- Gender & LGBTQ
- Immigration & Migration
- International Affairs
- Internet & Technology
- Methodological Research
- News Habits & Media
- Non-U.S. Governments
- Other Topics
- Politics & Policy
- Race & Ethnicity
- Email Newsletters
ABOUT PEW RESEARCH CENTER Pew Research Center is a nonpartisan fact tank that informs the public about the issues, attitudes and trends shaping the world. It conducts public opinion polling, demographic research, media content analysis and other empirical social science research. Pew Research Center does not take policy positions. It is a subsidiary of The Pew Charitable Trusts .
Copyright 2024 Pew Research Center
Terms & Conditions
Privacy Policy
Cookie Settings
Reprints, Permissions & Use Policy
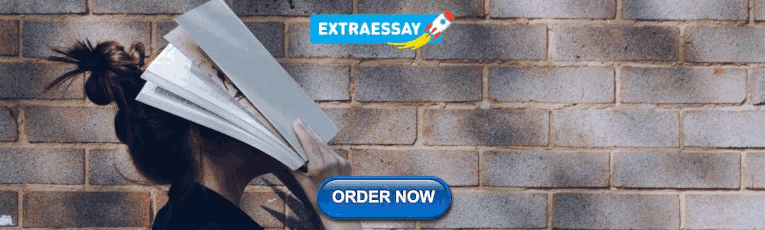
IMAGES
VIDEO
COMMENTS
The research was approved by the Swarthmore College Institutional Review Board, and written consent was obtained from participants prior to study participation. ... Each participant received a sheet of paper with the heading "My Thoughts" and a column on the right side with a sample check mark at the top. Participants were told that they ...
The ongoing and predicted decrease in Arctic sea ice raises concerns for ice-dependent species such as polar bears (), whose survival will depend on establishing populations in fragmented habitats and maintaining genetic connectivity among them ().Recent forecasts of reduced polar bear distribution and abundance are based on broad-scale climate projections and the estimated number of days that ...
Given the circumpolar distribution of polar bears, each population is experiencing a different level of climate change related effects, stressing the need for population-specific research. The focal population of this paper—the Western Hudson Bay—is near the southern limits of the species' range and is among the most affected by climate ...
Declining Arctic sea ice is increasing polar bear land use. Polar bears on land are thought to minimize activity to conserve energy. Here, we measure the daily energy expenditure (DEE), diet ...
Compassionate Care ALS. P.O. Box 1052. West Falmouth, Mass. 02574. Winchester Unitarian Society. 478 Main Street. Winchester, Mass. 01890. Daniel M. Wegner, a pioneering social psychologist who helped to reveal the mysteries of human experience through his work on thought suppression, conscious will, and mind perception, died July 5 at age 65.
This meta-analysis tests Wegner's original proposition that detection of immediate enhancement effects depends on the cognitive load experienced by individuals when enacting thought suppression and proposes that the introduction of additional cognitive load would compete for the allocation of existing cognitive resources and impair capacity for thought suppression.
A related phenomenon has been studied in the field of selective attention research. Tsal and Makovski coined the term "Attentional White Bear" (AWB) to describe the paradoxical consequences of an instruction to ignore an object in a visual field.In total contradiction to the instruction, participants actively allocated attention to the locations that contained the distractors they were ...
Tsal Y., Makovski T. (2006). The attentional white bear phenomenon: The mandatory allocation of attention to expected distractor locations. Journal of Experimental Psychology: Human Perception and Performance, 32, 351-363.
A more direct measure of proactive allocation of attention to distractors is provided in the attentional-white-bear (AWB) paradigm (Tsal & Makovski, 2006).In the AWB paradigm, participants engage in a primary flanker task—identifying a central letter flanked by two diagonal distractors (e.g., top left and bottom right) appearing in the same locations throughout a block of trials.
In three spatial cueing experiments, we investigated whether a negative search criterion (i.e., a task-relevant feature that negatively defines the target) can guide visual attention in a top-down ...
If a white bear came to mind, he told them, they should ring a bell. Despite the explicit instructions to avoid it, the participants thought of a white bear more than once per minute, on average. ... The research, published in the Journal of Personality and Social Psychology in 1987 (Vol. 53, No. 1) initiated an entirely new field of study on ...
The White Bear Suppression Inventory (WBSI) focuses on failing suppression attempts. E. Rassin. Psychology. 2003. Experimental studies have produced evidence to suggest that suppressing unwanted thoughts paradoxically results in even more unwanted thoughts. Therefore, suppression is considered to be an…. Expand.
Very quietly we paddle to shore in a raft from the research vessel, which has stopped at the mouth of a small river cascading into the Pacific, one of more than a hundred salmon-bearing rivers in ...
In a second paper, published in the same journal this week, a different group of researchers demonstrated similar capabilities with both wild and captive Alaskan polar bears and Swedish Eurasian ...
The results provide first evidence of top-down attentional suppression dependent on current task goals as a strategy operating in parallel to the goal-directed search for target-defining features. In three spatial cueing experiments, we investigated whether a negative search criterion (i.e., a task-relevant feature that negatively defines the target) can guide visual attention in a top-down ...
With urban areas expanding and climate change shrinking bears' habitats, the animals' interactions with humans will make — or break — efforts to preserve their populations.
Pages 85-89 In: The end of the road reader (Karin Hokkanen and Anne Stewart, eds). Published by Northwoods Writers Guild, PO Box 749, Ely, MN 55731-0749. Printed by Echo Press, Alexandria, MN. 109. Rogers, L. L. 1997. Nectar-feeding by Cape May, Tennessee, and Nashville Warblers in Minnesota.
The giant panda (Ailuropoda melanoleuca) is an iconic mammal, but the function of its black-and-white coloration is mysterious. Using photographs of giant pandas taken in the wild and state-of-the ...
The sea ice in Svalbard, Norway, where Polar Bears International conducts our annual maternal den study, has become less predictable in recent years, impacting research in the area. A changing Arctic and the loss of "bear-able" days. The loss of sea ice habitat from human-caused climate warming is the primary threat to polar bears—and to ...
Spatial patterns and rarity of the white-phased 'Spirit bear' allele reveal gaps in habitat protection Christina N. Service 1 , 2 , 3 Mathieu Bourbonnais 4 Megan S. Adams 1 , 2
This research paper, ... We addressed three conservation objectives for the white‐phased 'Spirit bear' polymorphism, a rare and endemic white‐coated phenotype of black bear (Ursus americanus ) in Kitasoo/Xai'xais and Gitga'at Territories and beyond in coastal British Columbia, Canada.
As the urban-wildland interface expands, coexistence will require both humans and wildlife to adapt to avoid conflict. The current combination of black bear use of low-conflict, forested habitats for most of the year, and their increased nocturnal behaviour in areas of higher human density, represents behavioural plasticity via spatial and ...
"This study examines how white Americans' beliefs about affirmative action intersect with three important workplace factors: supervisory status (supervisors vs. subordinates), employment sector (public vs. private), and workplace racial composition. ... Our analyses reveal declining opposition to affirmative action over time, though a majority of whites, regardless of authority level, still ...
The wider gap has been largely driven by Democrats: Today, 84% of Democrats say abortion should be legal in all or most cases, up from 72% in 2016 and 63% in 2007. Republicans' views have shown far less change over time: Currently, 38% of Republicans say abortion should be legal in all or most cases, nearly identical to the 39% who said this ...
How Pew Research Center will report on generations moving forward. Journalists, researchers and the public often look at society through the lens of generation, using terms like Millennial or Gen Z to describe groups of similarly aged people. This approach can help readers see themselves in the data and assess where we are and where we're ...
Previous research indicates that prior information about a target feature, such as its color, can speed search. ... Search 217,797,097 papers from all fields of science. Search. Sign In Create Free Account. DOI: 10.1177/0956797615626564; Corpus ID: 37893519; Taming the White Bear @article{Cunningham2016TamingTW, title={Taming the White Bear ...
The Department of Home Affais has published the final White Paper on Citizenship, Immigration and Refugee Protection: Towards a complete overhaul of the migration system in South Africa. "The outcome of the engagements and public comments is that the policy positions adopted in the White Paper enjoy wide support.
About eight-in-ten U.S. murders in 2021 - 20,958 out of 26,031, or 81% - involved a firearm. That marked the highest percentage since at least 1968, the earliest year for which the CDC has online records. More than half of all suicides in 2021 - 26,328 out of 48,183, or 55% - also involved a gun, the highest percentage since 2001.
The gender gap in pay has remained relatively stable in the United States over the past 20 years or so. In 2022, women earned an average of 82% of what men earned, according to a new Pew Research Center analysis of median hourly earnings of both full- and part-time workers. These results are similar to where the pay gap stood in 2002, when women earned 80% as much as men.