Emotion regulation ( ).
VLPFC | 44, 45, 47 | Left VLPFC supports mnemonic control (i.e., task switching, WM and semantic retrieval), and supports access to stored conceptual representations ( ). | Emotion regulation ( ). |
| | Left VLPFC is involved in elaborative (semantic/phonological) encoding of information into episodic memory, the specification of retrieval cues and the maintenance of LTM retrieval ( ; ). Right VLPFC supports memory encoding and retrieval of visuospatial stimuli, action imitation and motor inhibition ( ). | Inhibition of distracting emotions (right VLPFC for inhibition of negative emotions) ( ). |
mPFC | 25, 32 | Learning, memory, and decision-making ( ; ). | Dorsal-caudal mPFC involved in appraisal-expression of negative emotion; ventral-rostral PFC generates emotional regulation-responses ( ). |
OFC | 11, 12, 14 | Decision making ( ). | Emotional processing and responses ( ), social and emotional judgment ( ), facilitation of regret ( ). |
| | | Reward processing and reinforcement learning ( ). |
Effects Deriving From Different Modalities of Emotional Stimuli on Learning and Memory
As discussed above, evidence indicates the neural mechanisms underlying the emotional processing of valence and arousal involve the amygdala and PFC, where the amygdala responds to emotionally arousing stimuli and the PFC responds to the emotional valence of non-arousing stimuli. We have thus far primarily discussed studies examining neural mechanisms underlying the processing of emotional images. However, recent neuroimaging studies have investigated a wider range of visual emotional stimuli. These include words ( Sharot et al., 2004 ), pictures ( Dolcos et al., 2005 ; Weymar et al., 2011 ), film clips ( Cahill et al., 1996 ), and faces ( González-Roldan et al., 2011 ), to investigate neural correlates of emotional processing and the impact of emotion on subsequent memory. These studies provided useful supplemental information for future research on emotional effects of educational multimedia content (combination of words and pictures), an increasingly widespread channel for teaching and learning.
An event-related fMRI study examined the neural correlates of responses to emotional pictures and words in which both were manipulated in terms of positive and negative valence, and where neutral emotional content served as a baseline (“conditioned stimuli”/no activating emotion with valence rating of 5 that spans between 1/negative valence-9/positive valence), even though all stimuli were consistent in terms of arousal levels ( Kensinger and Schacter, 2006 ). Subjects were instructed to rate each stimulus as animate or inanimate and common or uncommon . The results revealed the activation of the amygdala in response to positive and negative valence (valence-independent) for pictures and words. A lateralization effect was observed in the amygdala when processing different emotional stimuli types. The left amygdala responded to words while either the right and/or bilateral amygdala activation regions responded to pictures. In addition, participants were more sensitive to emotional pictures than to emotional words. The mPFC responded more rigorously during the processing of positive than to that of negative stimuli, while the VLPFC responded more to negative stimuli. The researchers concluded that arousal-related responses occur in the amygdala, dmPFC, vmPFC, anterior temporal lobe and temporo-occipital junction, whereas valence-dependent responses were associated with the lateral PFC for negative stimuli and the mPFC for positive stimuli. The lateralization of the amygdala’s activation was consistent with that in other studies that also showed left-lateralized amygdala responses for words ( Hamann and Mao, 2002 ) vs. right-lateralized amygdala responses for images ( Pegna et al., 2005 ). However, a wide range of studies suggest that lateralization likely differs with sex ( Hamann, 2005 ), individual personality ( Hamann and Canli, 2004 ), mood ( Rusting, 1998 ), age ( Allard and Kensinger, 2014 ), sleep ( Walker, 2009 ), subject’s awareness of stimuli ( Morris et al., 1998 ), stress ( Payne et al., 2007 ) and other variables. Hence, these factors should be considered in future studies.
Event-related potentials (ERPs) were used to investigate the modality effects deriving from emotional words and facial expressions as stimuli in healthy, native German speakers ( Schacht and Sommer, 2009a ). German verbs or pseudo-words associated with positive, negative or neutral emotions were used, in addition to happy vs. angry faces, as well as neutral and slightly distorted faces. The results revealed that negative posterior ERPs were evoked in the temporo-parieto-occipital regions, while enhanced positive ERPs were evoked in the fronto-central regions (positive verbs and happy faces) when compared with neutral and negative stimuli. These findings were in agreement with the previous findings ( Schupp et al., 2003 ; Schacht and Sommer, 2009b ). While the same neuronal mechanisms appear to be involved in response to both emotional stimuli types, latency differences were also reported with faster responses to facial stimuli than to words, likely owing to more direct access to neural circuits-approximately 130 ms for happy faces compared to 380 ms for positive verbs ( Schacht and Sommer, 2009a ). Moreover, augmented responses observed in the later positive complex (LPP), i.e., larger late positive waves in response to emotional verbs (both positive and negative) and angry faces, all associated with the increased motivational significance of emotional stimuli ( Schupp et al., 2000 ) and increased selective attention to pictures ( Kok, 2000 ).
Khairudin et al. (2011) investigated effects of emotional content on explicit memory with two standardized stimuli: emotional words from the Affective Norms for English Words (ANEW) and emotional pictures from the IAPS. All stimuli were categorized as positive, negative or neutral, and displayed in two different trials. Results revealed that better memory for emotional images than for emotional words. Moreover, a recognition test demonstrated that positive emotional content was remembered better than negative emotional content. Researchers concluded that emotional valence significantly impacts memory and that negative valence suppressed the explicit memory. Another study by Khairudin et al. (2012) investigated the effects of emotional content on explicit verbal memory by assessing recall and recognition for emotionally positive, negative and neutral words. The results revealed that emotion substantially influences memory performance and that both positive and negative words were remembered more effectively than neutral words. Moreover, emotional words were remembered better in recognition vs. recall test.
Another group studied the impacts of emotion on memory using emotional film clips that varied in emotion with neutral, positive, negative and arousing contents ( Anderson and Shimamura, 2005 ). A subjective experiment for word recall and context recognition revealed that memory, for words associated with emotionally negative film clips, was lower than emotionally neutral, positive and arousing films. Moreover, emotionally arousing film clips were associated with enhanced context recognition memory but not during a free word recall test. Therefore, clarifying whether emotional stimuli enhance recognition memory or recall memory requires further investigation, as it appears that emotional information was better remembered for recognition compared to recall. In brief, greater attentional resource toward emotional pictures with large late positive waves of LPP in the posterior region, the amygdala responds to emotional stimuli (both words and pictures) independent on its valence, leading to enhanced memory. Table Table2 2 summarizes studies on the brain regions that respond to standardized stimuli as cited above, and also for pictures of emotional facial expression or Pictures of Facial Affect (POFA), Affective Norms for English Words (ANEW) for emotional words, as well as for the International Affective Digitized Sound System (IDAS) for emotional sounds.
Comparison of different emotional stimulus categories.
Study | Stimulus types | Emotion categories | Investigation | Brain imaging modality | Brain regions of interest | Findings | Subjects | Status | Age |
---|
| Pictures (IAPS) and words (ANEW) | Positive, negative, and neutral | Brain responses to emotionally positive, negative, and arousing words | Event-related fMRI | Amygdala, PFC, anterior temporal lobe, and temporooccipital junction | ∙ Amygdala, dmPFC, and vmPFC responded equally to both pictures and words regardless of valence. ∙ mPFC was more activated for positive content. ∙ VLPFC was more activated for negative content. ∙ Greater sensitivity for emotional pictures than words. | 21 adults (10 Female, 11 Male) | Healthy | 18–35 years |
| Words (ANEW) | High-arousal positive, high-arousal negative, and neutral | Brain responses to positive and negative emotionally arousing words | Event-related fMRI | Amygdala, vmPFC | ∙ Left amygdala activated for both positive and negative words. ∙ No activation observed in the vmPFC in response to positive or negative words. | 14 adults (All) | Healthy | 20–31 years |
| Faces | Positive, negative, and neutral | Responses to emotional face expression without primary visual areas | Event-related fMRI | Amygdala | ∙ Right amygdala activated for all emotional faces (anger, happiness, and fear). | 1 Male | Blind sight patient | 52 years |
| Pictures (IAPS) | Negative and neutral | Amygdala response to emotional experience during study and LTM | Event-related fMRI | Amygdala | ∙ Left amygdala activation during encoding was a predictor of subsequent recognition memory for pictures with high emotional intensity ratings. | 10 Female | Healthy | – |
| Film clips | Aggressive, sad, and neutral | Responses of EEG frequency bands on the emotional film content | EEG | Occipital (Posterior), central and frontal (anterior) | ∙ EEG theta (4–6 Hz) was more synchronized in occipital and frontal regions for the aggressive films compared with neutral films. ∙ EEG theta (4–6 Hz) respond specifically to visual emotional stimulus. ∙ EEG alpha is associated with attention and habituation. | 18 adults (All Female) | Healthy | 20–33 years |
| Pictures (IAPS) | Pleasant, neutral, and unpleasant | Brain responses to emotional pictures | ERP | Midline (Fz, Cz, and Pz) | ∙ More positivity for pleasant and unpleasant pictures than neutral pictures in the posterior regions. ∙ An indication of selective emotional processing (resulted from the motivational relevance of emotional pictures compared to neutral ones). | 14 Female | – | 18–24 years |
| Words (Spanish nouns)
Pictures (IAPS) | Negative, positive, neutral, and relaxing | Processing of emotional information in words and pictures | ERP | Frontal and parieto-occipital
Centro-parietal and frontal regions | ∙ Both emotional words and pictures were associated with an early posterior negativity and LPC. ∙ Emotional pictures elicited greater amplitude of early posterior negativity after stimulus presentation at the frontal and parieto-occipital regions. ∙ Positive pictures were associated with enhanced early posterior negativity amplitude in the right parieto-occipital regions. ∙ An arousal-dependent effect was observed in the left parieto-occipital regions for both positive and negative stimuli. | 21 volunteers (19 Female, 2 Male) 28 volunteers (21 Female, 7 Male) | Healthy Healthy | 19–27 years 19–29 years |
| Facial expression (POFA) | Fearful vs. neutral | Spatial attention effects on emotional face processing. | ERP | Frontal, central and posterior regions | Faces enhanced N170 amplitude reflecting that spatial attention modulates face encoding at lateral posterior electrodes. However, N170 was insensitive to emotional expression. | 20 subjects (11 Female, 7 Male, 2 excluded due to excess artifacts) | Healthy | 18–32 years |
| Sentence | Negative/high arousal and Neutral/ low arousal | Impact of emotional verb processing in short sentences (Reading) | ERP | Centro-parietal regions | Effect on LPC of negative and high-arousal words, while LPC was not affected by arousal-related words alone. Reported the importance of valence and arousal in emotion-related ERP effects. | 21 participants (11 Female, 10 Male) | Healthy | – |
| Sound (IADS) | Pleasant, unpleasant, and neutral | Auditory cortex response to emotional stimuli | fNIRS | Auditory cortex | Both pleasant and unpleasant sounds led to greater activation in the left and right auditory cortex compared with neutral sound. | 17 participants (10 Female, 7 Male) | Healthy | – |
Neuroimaging Techniques for the Investigation of Emotional-Cognitive Interactions
The brain regions associated with cognitive-emotional interactions can be studied with different functional neuroimaging techniques (fMRI, PET, and fNIRS) to examine hemodynamic responses (indirect measurement). EEG is used to measure brain electrical dynamics (direct measurement) associated with responses to cognitive and emotional tasks. Each technique has particular strengths and weaknesses, as described below.
Functional Magnetic Resonance Imaging (fMRI)
Functional magnetic resonance imaging is a widely used functional neuroimaging tool for mapping of brain activation as it provides a high spatial resolution (a few millimeters). fMRI is an indirect measure of hemodynamic response by measuring changes in local ratios of oxy-hemoglobin vs. deoxy-hemoglobin, typically known as a blood oxygenation level dependent (BOLD) signal ( Cabeza and Nyberg, 2000 ). Dolcos et al. (2005) examined the effects of emotional content on memory enhancement during retrieval process using event-related fMRI to measure retrieval-related activity after a retention interval of 1 year. The researchers concluded that successful retrieval of emotional pictures involved greater activation of the amygdala as well as the entorhinal cortex and hippocampus than that of neutral pictures. Both the amygdala and hippocampus were rigorously activated during recollection compared to familiarity recognition, whereas no differences were found in the entorhinal cortex for either recollection or familiarity recognition. Moreover, a study investigates motivation effect (low vs. high monetary reward) on episodic retrieval by manipulating task difficulty, fMRI data reports that increased activation in the substantia nigra/VTA, MTL, dmPFC, and DLPFC when successful memory retrieval with high difficulty than with low difficulty. Moreover, reward-related of functional connectivities between the (i) SN/VTA–MTL and (ii) SN/VTA–dmPFC appear to increases significantly with increases retrieval accuracy and subjective motivation. Thus, Shigemune et al. (2017) suggest that reward/motivation-related memory enhancement modulated by networking between the SN/VTA (reward-related), dmPFC (motivation-related) and MTL (memory-related) network as well as DLPFC (cognitive controls) with high task difficulty.
Taken together, these findings indicate that the amygdala and MTL have important roles in the recollection of emotional and motivational memory. Another fMRI study reported that greater success for emotional retrieval (emotional hits > misses ) was associated with neural activation of the bilateral amygdala, hippocampus, and parahippocampus, whereas a higher success rate for neutral retrieval is associated with a greater activity in right posterior parahippocampus regions ( Shafer and Dolcos, 2014 ). Hence, fMRI has clearly revealed interactions between cognitive and emotional neural networks during information processing, particularly in response to emotion-related content. Such interactions appear to modulate memory consolidation while also mediating encoding and retrieval processes that underlie successful LTM formation and memory recall. More specifically, it appears that amygdala activation modulates both the hippocampus and visual cortex during visual perception and enhances the selection and organization of salient information via the “bottom-up” approach to higher cognitive functions directed at awareness. Although fMRI is widely used, it poses several limitations such as poor temporal resolution, expensive setup costs, plus the difficulty of having a subject hold still during the procedure in an electromagnetically shielded room (immobility). Furthermore, fMRI is slightly more metabolically sluggish, as BOLD signal exhibits an initial dip, where the increase of subsequent signal is delayed by 2–3 s and it takes approximately 6–12 s to reach to a peak value that reflects the neural responses elicited by a stimulus ( Logothetis et al., 2001 ). This means that fMRI having a coarse temporal resolution (several seconds) when compared with electrophysiological techniques (a few milliseconds) and also not a great technique for visualizing subcortical regions (mesencephalon and brainstem) due to metabolically sluggish compared to PET.
Positron Emission Tomography (PET)
Positron emission tomography is another functional neuroimaging tool that maps CNS physiology and neural activation by measuring glucose metabolism or regional cerebral blood flow (rCBF). PET uses positron-emitting radionuclides such as 18 F-fluorodeoxyglucose (FDG) and positron-emitting-oxygen isotope tagged with water ([ 15 O] H 2 O), etc. This technique identifies different neural networks involving pleasant, unpleasant and neutral emotions ( Lane et al., 1997 ). It thus far appears that increased rCBF in the mPFC, thalamus, hypothalamus, and midbrain associated with pleasant and unpleasant emotional processing, while unpleasant emotions are more specifically associated with the bilateral OTC, cerebellum, left parahippocampal gyrus, hippocampus, and amygdala; moreover, the caudate nucleus is associated with pleasant emotions.
Using PET scanning demonstrated that emotional information enhances visual memory recognition via interactions between perception and memory systems, specifically with greater activation of the lingual gyrus for visual stimuli ( Taylor et al., 1998 ). The results also showed that strong negative emotional valence appeared to enhance the processing of early sensory input. Moreover, differences in neural activation appeared in the left amygdaloid complex (AC) during encoding, while the right PFC and mPFC responded during recognition memory. Similarly, Tataranni et al. (1999) identified CNS regions associated with appetitive states (hunger and satiation) ( Tataranni et al., 1999 ). Hunger stimulated increased rCBF uptake in multiple regions including the hypothalamus, insular cortex, limbic and paralimbic regions (anterior cingulate cortex, parahippocampal and hippocampal formation, the anterior temporal and posterior orbitofrontal cortex), as well as the thalamus, caudate, precuneus, putamen, and cerebellum. Satiation was associated with increased rCBF uptake in the bilateral vmPFC, the DLPFC, and the inferior parietal lobule. These results imply that (i) subcortical regions associated with emotion/motivation involved in hunger that signals distressing feeling (discomfort, pain and anxiety) for the regulation of food intake; and (ii) the PFC associated with inhibition of inappropriate behavioral response involved in satiation that signals excessive food consumption for a termination of meal.
In a study of emotional self-generation using PET noted that the insular cortex, secondary somatosensory cortex, and hypothalamus, as well as the cingulate cortex and nuclei in the brainstem’s tegmentum, including PAG, parabrachial nucleus, and substantia nigra maintained current homeostasis by generating regulatory signals ( Damasio et al., 2000 ). PET scanning has also been used for neuroanatomical mapping of emotions ( Davidson and Irwin, 1999 ), emotional processing ( Choudhary et al., 2015 ), and cognitive functions ( Cabeza and Nyberg, 2000 ). Although PET scanning has a relatively good spatial resolution for both the brain and bodily functions, it is costly and yields lower temporal resolution than does EEG and is invasive as opposed to fMRI. Moreover, PET tends to show better activation of more ancient brain regions in the mesencephalon and brainstem when compared to fMRI. Hence, it is generally reserved for the clinical diagnoses of cancers, neurological diseases processes (e.g., epilepsy and Alzheimer’s disease), and heart diseases.
Electroencephalography (EEG)
Electroencephalography obtains high temporal resolution in milliseconds, portable, less expensive, and non-invasive techniques by attaching scalp electrodes to record brain electrical activity. Moreover, numerous studies reported that EEG is useful in mapping CNS cognitive and emotional processing. The technique offers a comprehensive range of feature extraction and analysis methods, including power spectral analysis, EEG coherence, phase delay, and cross-power analysis. One study examined changes in EEG oscillations in the amygdala during the consolidation of emotionally aroused memory processing that exhibited theta (4–8 Hz) activity ( Paré et al., 2002 ), indicating the facilitation of memory consolidation, improved retention of emotional content, and enhanced memory recall. This finding was later supported by the revelation of increased theta activity in the right frontal ( Friese et al., 2013 ) and right temporal cortices ( Sederberg et al., 2003 ) and consequently associated with the successful encoding of new information. Another study ( Buzsáki, 2002 ) revealed that theta oscillations were positively related to the activation of the hippocampus represent the active brain state during sensory, motor and memory-related processing. The theta waves are generated through an interaction between the entorhinal cortex, the Schaffer collateral (CA3 region) and the pyramidal cell dendrites (both CA3 and CA1 regions) that result in a synaptic modification underlie learning and memory. Thus, theta oscillation is thought to be associated with the encoding of new memories.

Increased gamma oscillation in the neocortex and right amygdala have been reported in response to emotionally arousing pictures during learning and memory tasks undertaken by 148 right-handed female participants ( Headley and Paré, 2013 ). A more detailed study by Müller et al. (1999) reported increased gamma potentials in the left frontal and temporal regions in response to images having a negative valence, whereas increased gamma-bands in the right frontal regions were observed in responses to images with positive valence for 11 right-handed male participants. During an emotionally positive experience, another study reported significantly increased EEG theta-alpha coherence between prefrontal and posterior parietal regions ( Aftanas and Golocheikine, 2001 ). They concluded the change was associated with heightened attention in association with improved performance in memory and emotional processing. Thus, we have a number of EEG investigations of left and right hemispheric activity while processing positive (pleasant) and negative (unpleasant) stimuli that revealed differences in regional electrophysiological activation. Nonetheless, EEG exhibits a relatively poor spatial resolution approximately 5 to 9 cm compared with fMRI and PET ( Babiloni et al., 2001 ). Thus, scalp EEG unable to measure activation much below cortex owing to the distortion of scalp potentials where different volume conduction effects of the cortex, dura mater, skull, and scalp resulting in imprecise localization of the electromagnetic field patterns associated with neural current flow. Subsequent studies have demonstrated that the EEG spatial resolution can be improved using high-resolution EEG (high-density electrode arrays to increase spatial sampling) with surface Laplacian estimation and cortical imaging (details discussion of this area is beyond the scope of this review, see ( Nunez et al., 1994 ) for theoretical and experimental study) or integrating multiple imaging modalities that provide complement information, for instance EEG-fMRI and EEG-fNIRS ( Dale and Halgren, 2001 ).
Functional Near-Infrared Spectroscopy (fNIRS)
Functional near-infrared spectroscopy is an emerging and relatively low-cost imaging technique that is also portable and non-invasive. It can be used to map the hemodynamic responses associated with brain activation. This technology measures cerebral changes in the concentration of oxygenated hemoglobin (oxy-Hb) vs. deoxygenated hemoglobin (deoxy-Hb) using optodes (light emitters and detectors) placed on the scalp ( Villringer et al., 1993 ). It is limited to visualizations of cortical activity compared to the subcortical regions, and findings only imply increased brain activity associated with increased glucose and oxygen consumption. Elevations in cerebral blood flow and oxygen delivery exceed quo oxygen consumption, thereby enabling changes in local cerebral blood oxygenation to be measured by optic penetration.
The number of studies that have implemented this investigative technique are associated with task performance ( Villringer et al., 1993 ), including exercise ( Perrey, 2008 ), cognitive workload ( Durantin et al., 2014 ), psychiatric disorders ( Ehlis et al., 2014 ), emotional processing ( Bendall et al., 2016 ), and aging ( Hock et al., 1995 ). One study used fNIRS to examine the relationship between subjective happiness and emotional changes ( Oonishi et al., 2014 ). The results revealed that the level of subjective happiness influenced the pattern of left-right PFC activation during the emotion-related task, showing increased oxy-Hb in the left PFC when viewing pleasant pictures, and increased oxy-Hb in the right PFC when viewing unpleasant pictures. Viewing unpleasant emotional stimuli accompanied increased in oxy-Hb levels in the bilateral VLPFC while also activating several regions in both the right VLPFC (BA45/47) and left VLPFC (BA10/45/46/47). However, another fNIRS study reported that viewing pleasant emotional stimuli was associated with decreased oxy-Hb in the left DLPFC (BA46/10) when affective images were presented for 6 s ( Hoshi et al., 2011 ). Thus, this study found an opposite pattern indicating left hemisphere involvement in positive/approach processing and right hemisphere involvement in negative/withdrawal processing ( Davidson, 1992 ; Davidson and Irwin, 1999 ). This inconsistent finding of frontal hemispheric asymmetric might result from the comparison of state-related changes rather than baseline levels of asymmetric. Thus, several issues should take into consideration: (i) methodological issues to assess hemispheric asymmetry, including requires repeat measures of anterior asymmetry for at least two sessions, stimulus content should comprise both positive valence and negative valence while maintaining at a similar level of arousal and with a baseline resting condition, appropriate selection of reference electrode and individual differences, etc; and (ii) conceptual issues is related to the fact that prefrontal cortex is an anatomically and functionally heterogeneous and complex region interacts with other cortical and subcortical structures during emotional processing ( Davidson, 2004 ). Another fNIRS study examined the relationship between PFC function and cognitive control of emotion ( Ozawa et al., 2014 ). This was done by presenting emotional IAPS pictures for 5.2 s, followed by the n -back task. The results revealed a significantly greater increase in oxy-HB in the mPFC and left superior frontal gyrus in response to negative pictures compared with neutral pictures. Meanwhile, no significant hemodynamic changes were observed during image presentation and the n -back task, indicating the need for further investigation.
Factors Affecting the Effect of Emotion on Learning and Memory
The preceding section described neuroimaging techniques used to examine brain responses to emotional stimuli during WM processing leading to LTM. This section presents six key factors that are recommended for consideration in the experimental design and appropriate protocol.
Individual Differences
A number of studies have reported numerous influences in addition to a range of individual differences in emotional processing. These include personality traits ( Montag and Panksepp, 2017 ), intellectual ability ( Brackett et al., 2004 ), and sex ( Cahill, 2003 ). Moreover, sex hormones and personality traits (e.g., extraversion and neuroticism) appear to influence individual responses to emotional stimuli as well as modulate emotional processing. Appropriate screening with psychological testing as well as balancing experimental cohorts in terms of sex can help reduce spurious results owing to individual differences.
Age-Related Differences
Studies have also shown that older adults are associated with the greater familiarity with psychological stress and emotional experiences, thus causing positivity biases in emotional processing and better emotional control than in younger adults ( Urry and Gross, 2010 ; Allard and Kensinger, 2014 ). Consequently, the age of participants in a sample population should be considered for both cognitive and emotional studies.
Emotional Stimulus Selection
The selection of emotional stimuli for experimental studies is generally divided into two streams: (1) discrete emotional, and (2) dimensional emotions of valence, arousal, dominance and familiarity ( Russell, 1980 ; Barrett, 1998 ). The latter include pictures from the IAPS database and words from the ANEW database, which are both available for non-commercial research. Appropriate selection of emotional stimuli is another important consideration that ensures experimental tasks are suitable for the investigation of emotional processing in learning and memory. Furthermore, the type of stimulus determines stimulus presentation duration, especially for experimental tasks involving the induction of emotions.
Self-assessment Techniques
There are numerous self-assessment techniques used to measure individual emotional states ( Bradley and Lang, 1994 ). The most widely used techniques are the Self-Assessment Manikin (SAM), the Semantic Differential (SD) scale, and the Likert scale. The SAM is a non-verbal pictorial assessment technique directly measures emotional responses to emotional stimuli for valence, arousal, and dominance. The SD scale consists of a set of bipolar adjective pairs for the subjective rating of image stimuli. The Likert’s “ x -point” scale allows participants to rate their own emotional responses. If a study does not seek to assess distinct emotional states but rather involves the assessment of two primary dimensions of emotion (positive and negative valence), then the Positive and Negative Affect Schedule (PANAS) is a recommended method ( Watson et al., 1988 ). Thus, selection of the most appropriate self-assessment technique is an important part of the experimental design but can also become an overwhelming task.
Selection of Brain Imaging Techniques
As mentioned above, the two major types of brain imaging techniques EEG (direct) and fMRI/PET/fNIRS (indirect) have respective advantages and disadvantages. To overcome these limitations, simultaneous or combined dual-modality imaging (EEG-fMRI or EEG-fNIRS) can now be implemented for complementary data collection. Although functional neuroimaging works to identify the neural correlates of emotional states, technologies such as deep brain stimulation (DBS) and connectivity maps might provide new opportunities to seek understanding of emotions and its corresponding psychological responses.
Neurocognitive Research Design
The neuroscience of cognition and emotion requires appropriate task designs to accomplish specific study objectives ( Amin and Malik, 2013 ). Environmental factors, ethical issues, memory paradigms, cognitive task difficulty, and emotional induction task intensity must be considered for this.
Numerous neuroimaging studies cited thus far have indicated that emotions influence memory processes, to include memory encoding, memory consolidation, and memory retrieval. Emotional attentional and motivational components might explain why emotional content exhibits privileged information processing. Emotion has a “pop-out” effect that increases attention and promotes bottom-up instinctual impact that enhances awareness. Significant emotional modulation affects memory consolidation in the amygdala, and emotional content also appears to mediate memory encoding and retrieval in the PFC, leading to slow rates of memory lapse accompanied by the accurate recall. Moreover, cognitive and emotional interactions also appear to modulate additional memory-related CNS regions, such as the frontal, posterior parietal and visual cortices. The latter are involved in attentional control, association information, and the processing of visual information, respectively. Therefore, higher-level cognitive functions such as learning and memory, appear to be generally guided by emotion, as outlined in the Panksepp’s framework of brain processing ( Panksepp, 1998 ).
Neuroimaging findings also indicate the involvement of the PFC in emotional processing by indirectly influencing WM and semantic memory ( Kensinger and Corkin, 2003 ). This is reflected by the involvement of the DLPFC in WM and the role played by VLPFC in semantic processing, both of which have been found to enhance or impair semantic encoding task performance when emotion is involved. Various parts of the lateral PFC (ventrolateral, dorsolateral and medial prefrontal cortical regions) are suspected of having key roles that support memory retrieval ( Simons and Spiers, 2003 ). All of these findings suggest that PFC-MTL interactions underlie effective semantic memory encoding and thus strategically mediate information processing with increased transfer to the hippocampus, consequently enhancing memory retrieval. Accordingly, learning strategies that emphasize emotional factors are more likely to result in long-term knowledge retention. This consideration is potentially useful in the design of educational materials for academic settings and informed intelligent tutoring systems.
Based on numerous previous findings, future research might take emotional factors more seriously and more explicitly in terms of their potential impact on learning. By monitoring the emotional state of students, the utilization of scientifically derived knowledge of stimulus selection can be particularly useful in the identification of emotional states that advance learning performance and outcomes in educational settings. Moreover, functional neuroimaging investigations now include single and/or combined modalities that obtain complementary datasets that inform a more comprehensive overview of neuronal activity in its entirety. For example, curiosity and motivation promote learning, as it appears cognitive network become energized by the mesolimbic-mesocortical dopamine system (generalized motivational arousal/SEEKING system). In addition, the identification of emotional impact on learning and memory potentially has direct implications for healthy individuals as well as patients with psychiatric disorders such as depression, anxiety, schizophrenia, autism, mania, obsessive-compulsive disorder and post-traumatic stress disorder (PTSD) ( Panksepp, 2011a ). To emphasize, depression and anxiety are the two most commonly diagnosed psychiatric disorders associated with learning/memory impairment and pose negative consequences that (i) limit the total amount of information that can otherwise be learned, and (ii) inhibit immediate recall as well as memory retention and retrieval of newly learned information. Depression and anxiety are also associated with negative emotions such as hopelessness, anxiety, apathy, attention deficit, lack of motivation, and motor and mental insufficiencies. Likewise, neuroscience studies report that decreased activation of the dorsal limbic (the anterior and posterior cingulate) as well as in the prefrontal, premotor and parietal cortices causes attentional disturbance, while increased neural activation in the ventral paralimbic region (the subgenual cingulate, anterior insula, hypothalamus and caudate) is associated with emotional and motivational disorders ( Mayberg, 1997 ).
Concluding Remarks, Open Questions, and Future Directions
Substantial evidence has established that emotional events are remembered more clearly, accurately and for longer periods of time than are neutral events. Emotional memory enhancement appears to involve the integration of cognitive and emotional neural networks, in which activation of the amygdala enhances the processing of emotionally arousing stimuli while also modulating enhanced memory consolidation along with other memory-related brain regions, particularly the amygdala, hippocampus, MTL, as well as the visual, frontal and parietal cortices. Similarly, activation of the PFC enhances cognitive functions, such as strategic and semantic processing that affect WM and also promote the establishment of LTM. Previous studies have primarily used standardized emotional visual, or auditory stimuli such as pictures, words, facial expression, and film clips, often based on the IAPS, ANEW, and POFA databases for emotional pictures, words and facial expressions, respectively. Further studies have typically focused on the way individuals memorize (intentional or incidental episodic memory paradigm) emotional stimuli in controlled laboratory settings. To our knowledge, there are few objective studies that employed brain-mapping techniques to examine semantic memory of learning materials (using subject matter) in the education context. Furthermore, influences derived from emotional factors in human learning and memory remains unclear as to whether positive emotions facilitate learning or negative emotions impair learning and vice versa. Thus, several remaining questions should be addressed in future studies, including (i) the impact of emotion on semantic knowledge encoding and retrieval, (ii) psychological and physiological changes associated with semantic learning and memory, and (iii) the development of methods that incorporate emotional and motivational aspects that improve educational praxes, outcomes, and instruments. The results of studies on emotion using educational learning materials can indeed provide beneficial information for informed designs of new educational courses that obtain more effective teaching and help establish better informed learning environments. Hence, to understand how emotion influence learning and memory requires understanding of an evolutionary consideration of the nested hierarchies of CNS emotional-affective processes as well as a large-scale network, including the midbrain’s PAG and VTA, basal ganglia (amygdala and NAc), and insula, as well as diencephalon (the cingulate and medial frontal cortices through the lateral and medial hypothalamus and medial thalamus) together with the MTL, including the hippocampus as well as the entorhinal cortex, perirhinal cortex, and parahippocampal cortices that responsible for declarative memories. Moreover, the SEEKING system generates positive subjective emotional states-positive expectancy, enthusiastic exploration, and hopefulness, apparently, initiates learning and memory in the brain. All cognitive activity is motivated from ‘underneath’ by basic emotional and homeostatic needs (motivational drives) that explore environmental events for survival while facilitating secondary processes of learning and memory.
Author Contributions
CMT drafted this manuscript. CMT, HUA, MNMS, and ASM revised this draft. All authors reviewed and approved this manuscript.
Conflict of Interest Statement
The authors declare that the research was conducted in the absence of any commercial or financial relationships that could be construed as a potential conflict of interest.
Acknowledgments
We would like to thank Ministry of Education (MOE), Malaysia for the financial support. We gratefully thank Frontiers in Psychology, Specialty Section Emotion Sciences reviewers and the journal Associate Editor, for their helpful input and feedback on the content of this manuscript.
Funding. This research work was supported by the HiCoE grant for CISIR (Ref No. 0153CA-002), Ministry of Education (MOE), Malaysia.
- Aftanas L., Golocheikine S. (2001). Human anterior and frontal midline theta and lower alpha reflect emotionally positive state and internalized attention: high-resolution EEG investigation of meditation. Neurosci. Lett. 310 57–60. 10.1016/S0304-3940(01)02094-8 [ PubMed ] [ CrossRef ] [ Google Scholar ]
- Allard E. S., Kensinger E. A. (2014). Age-related differences in neural recruitment during the use of cognitive reappraisal and selective attention as emotion regulation strategies. Front. Psychol. 5 : 296 10.3389/fpsyg.2014.00296 [ PMC free article ] [ PubMed ] [ CrossRef ] [ Google Scholar ]
- Amaral D. G., Behniea H., Kelly J. (2003). Topographic organization of projections from the amygdala to the visual cortex in the macaque monkey. Neuroscience 118 1099–1120. 10.1016/S0306-4522(02)01001-1 [ PubMed ] [ CrossRef ] [ Google Scholar ]
- Amin H., Malik A. S. (2013). Human memory retention and recall processes. Neurosciences 18 330–344. [ PubMed ] [ Google Scholar ]
- Anderson A. K., Phelps E. A. (2001). Lesions of the human amygdala impair enhanced perception of emotionally salient events. Nature 411 305–309. 10.1038/35077083 [ PubMed ] [ CrossRef ] [ Google Scholar ]
- Anderson L., Shimamura A. P. (2005). Influences of emotion on context memory while viewing film clips. Am. J. Psychol. 118 323–337. [ PubMed ] [ Google Scholar ]
- Ashby F. G., Isen A. M. (1999). A neuropsychological theory of positive affect and its influence on cognition. Psychol. Rev. 106 529–550. 10.1037/0033-295X.106.3.529 [ PubMed ] [ CrossRef ] [ Google Scholar ]
- Babiloni F., Cincotti F., Carducci F., Rossini P. M., Babiloni C. (2001). Spatial enhancement of EEG data by surface Laplacian estimation: the use of magnetic resonance imaging-based head models. Clin. Neurophysiol. 112 724–727 10.1016/S1388-2457(01)00494-1 [ PubMed ] [ CrossRef ] [ Google Scholar ]
- Badre D., Wagner A. D. (2007). Left ventrolateral prefrontal cortex and the cognitive control of memory. Neuropsychologia 45 2883–2901. 10.1016/j.neuropsychologia.2007.06.015 [ PubMed ] [ CrossRef ] [ Google Scholar ]
- Barbey A. K., Koenigs M., Grafman J. (2013). Dorsolateral prefrontal contributions to human working memory. Cortex 49 1195–1205. 10.1016/j.cortex.2012.05.022 [ PMC free article ] [ PubMed ] [ CrossRef ] [ Google Scholar ]
- Barbey A. K., Krueger F., Grafman J. (2009). Structured event complexes in the medial prefrontal cortex support counterfactual representations for future planning. Philos. Trans. R. Soc. B Biol. Sci. 364 1291–1300. 10.1098/rstb.2008.0315 [ PMC free article ] [ PubMed ] [ CrossRef ] [ Google Scholar ]
- Barrett L. F. (1998). Discrete emotions or dimensions? The role of valence focus and arousal focus. Cogn. Emot. 12 579–599. 10.1080/026999398379574 [ CrossRef ] [ Google Scholar ]
- Bartolic E., Basso M., Schefft B., Glauser T., Titanic-Schefft M. (1999). Effects of experimentally-induced emotional states on frontal lobe cognitive task performance. Neuropsychologia 37 677–683. 10.1016/S0028-3932(98)00123-7 [ PubMed ] [ CrossRef ] [ Google Scholar ]
- Battaglia F. P., Benchenane K., Sirota A., Pennartz C. M., Wiener S. I. (2011). The hippocampus: hub of brain network communication for memory. Trends Cogn. Sci. 15 310–318. 10.1016/j.tics.2011.05.008 [ PubMed ] [ CrossRef ] [ Google Scholar ]
- Bayer M., Sommer W., Schacht A. (2010). Reading emotional words within sentences: the impact of arousal and valence on event-related potentials. Int. J. Psychophysiol. 78 299–307. 10.1016/j.ijpsycho.2010.09.004 [ PubMed ] [ CrossRef ] [ Google Scholar ]
- Bechara A., Damasio H., Damasio A. R. (2000). Emotion, decision making and the orbitofrontal cortex. Cereb. Cortex 10 295–307. 10.1093/cercor/10.3.295 [ PubMed ] [ CrossRef ] [ Google Scholar ]
- Bendall R. C., Eachus P., Thompson C. (2016). A brief review of research using near-infrared spectroscopy to measure activation of the prefrontal cortex during emotional processing: the importance of experimental design. Front. Hum. Neurosci. 10 : 529 10.3389/fnhum.2016.00529 [ PMC free article ] [ PubMed ] [ CrossRef ] [ Google Scholar ]
- Blumenfeld R. S., Ranganath C. (2006). Dorsolateral prefrontal cortex promotes long-term memory formation through its role in working memory organization. J. Neurosci. 26 916–925. 10.1523/JNEUROSCI.2353-05.2006 [ PMC free article ] [ PubMed ] [ CrossRef ] [ Google Scholar ]
- Blumenfeld R. S., Ranganath C. (2007). Prefrontal cortex and long-term memory encoding: an integrative review of findings from neuropsychology and neuroimaging. Neuroscientist 13 280–291. 10.1177/1073858407299290 [ PubMed ] [ CrossRef ] [ Google Scholar ]
- Brackett M. A., Mayer J. D., Warner R. M. (2004). Emotional intelligence and its relation to everyday behaviour. Pers. Individ. Dif. 36 1387–1402. 10.1016/S0191-8869(03)00236-8 [ CrossRef ] [ Google Scholar ]
- Bradley M. M., Lang P. J. (1994). Measuring emotion: the self-assessment manikin and the semantic differential. J. Behav. Ther. Exp. Psychiatry 25 49–59. 10.1016/0005-7916(94)90063-9 [ PubMed ] [ CrossRef ] [ Google Scholar ]
- Brod G., Werkle-Bergner M., Shing Y. L. (2013). The influence of prior knowledge on memory: a developmental cognitive neuroscience perspective. Front. Behav. Neurosci. 7 : 139 10.3389/fnbeh.2013.00139 [ PMC free article ] [ PubMed ] [ CrossRef ] [ Google Scholar ]
- Buzsáki G. (2002). Theta oscillations in the hippocampus. Neuron 33 325–340. 10.1016/S0896-6273(02)00586-X [ PubMed ] [ CrossRef ] [ Google Scholar ]
- Cabeza R., Nyberg L. (2000). Imaging cognition II: an empirical review of 275 PET and fMRI studies. J. Cogn. Neurosci. 12 1–47. 10.1162/08989290051137585 [ PubMed ] [ CrossRef ] [ Google Scholar ]
- Cahill L. (2003). Sex-and hemisphere-related influences on the neurobiology of emotionally influenced memory. Prog. Neuro Psychopharmacol. Biol. Psychiatry 27 1235–1241. 10.1016/j.pnpbp.2003.09.019 [ PubMed ] [ CrossRef ] [ Google Scholar ]
- Cahill L., Haier R. J., Fallon J., Alkire M. T., Tang C., Keator D., et al. (1996). Amygdala activity at encoding correlated with long-term, free recall of emotional information. Proc. Natl. Acad. Sci. U.S.A. 93 8016–8021. 10.1073/pnas.93.15.8016 [ PMC free article ] [ PubMed ] [ CrossRef ] [ Google Scholar ]
- Cahill L., McGaugh J. L. (1998). Mechanisms of emotional arousal and lasting declarative memory. Trends Neurosci. 21 294–299. 10.1016/S0166-2236(97)01214-9 [ PubMed ] [ CrossRef ] [ Google Scholar ]
- Camille N., Coricelli G., Sallet J., Pradat-Diehl P., Duhamel J. -R., Sirigu A. (2004). The involvement of the orbitofrontal cortex in the experience of regret. Science 304 1167–1170. 10.1126/science.1094550 [ PubMed ] [ CrossRef ] [ Google Scholar ]
- Canli T., Zhao Z., Brewer J., Gabrieli J. D., Cahill L. (2000). Event-related activation in the human amygdala associates with later memory for individual emotional experience. J. Neurosci. 20 : RC99 . [ PMC free article ] [ PubMed ] [ Google Scholar ]
- Carew T. J., Magsamen S. H. (2010). Neuroscience and education: an ideal partnership for producing evidence-based solutions to guide 21 st century learning. Neuron 67 685–688. 10.1016/j.neuron.2010.08.028 [ PubMed ] [ CrossRef ] [ Google Scholar ]
- Choudhary M., Kumar A., Tripathi M., Bhatia T., Shivakumar V., Beniwal R. P., et al. (2015). F-18 fluorodeoxyglucose positron emission tomography study of impaired emotion processing in first episode schizophrenia. Schizophr. Res. 162 103–107. 10.1016/j.schres.2015.01.028 [ PMC free article ] [ PubMed ] [ CrossRef ] [ Google Scholar ]
- Comte M., Schön D., Coull J. T., Reynaud E., Khalfa S., Belzeaux R., et al. (2014). Dissociating bottom-up and top-down mechanisms in the cortico-limbic system during emotion processing. Cereb. Cortex 26 144–155. 10.1093/cercor/bhu185 [ PubMed ] [ CrossRef ] [ Google Scholar ]
- Craig A. D., Craig A. (2009). How do you feel–now? The anterior insula and human awareness. Nat. Rev. Neurosci. 10 59–70. 10.1038/nrn2555 [ PubMed ] [ CrossRef ] [ Google Scholar ]
- Curtis C. E., D’Esposito M. (2003). Persistent activity in the prefrontal cortex during working memory. Trends Cogn. Sci. 7 415–423. 10.1016/S1364-6613(03)00197-9 [ PubMed ] [ CrossRef ] [ Google Scholar ]
- Cuthbert B. N., Schupp H. T., Bradley M. M., Birbaumer N., Lang P. J. (2000). Brain potentials in affective picture processing: covariation with autonomic arousal and affective report. Biol. Psychol. 52 95–111. 10.1016/S0301-0511(99)00044-7 [ PubMed ] [ CrossRef ] [ Google Scholar ]
- D’Mello S., Lehman B., Pekrun R., Graesser A. (2014). Confusion can be beneficial for learning. Learn. Instr. 29 153–170. 10.1016/j.learninstruc.2012.05.003 [ CrossRef ] [ Google Scholar ]
- Dael N., Mortillaro M., Scherer K. R. (2012). Emotion expression in body action and posture. Emotion 12 1085–1101. 10.1037/a0025737 [ PubMed ] [ CrossRef ] [ Google Scholar ]
- Dale A. M., Halgren E. (2001). Spatiotemporal mapping of brain activity by integration of multiple imaging modalities. Curr. Opin. Neurobiol. 11 202–208. 10.1016/S0959-4388(00)00197-5 [ PubMed ] [ CrossRef ] [ Google Scholar ]
- Damasio A., Carvalho G. B. (2013). The nature of feelings: evolutionary and neurobiological origins. Nat. Rev. Neurosci. 14 143–152. 10.1038/nrn3403 [ PubMed ] [ CrossRef ] [ Google Scholar ]
- Damasio A. R., Grabowski T. J., Bechara A., Damasio H., Ponto L. L., Parvizi J., et al. (2000). Subcortical and cortical brain activity during the feeling of self-generated emotions. Nat. Neurosci. 3 1049–1056. 10.1038/79871 [ PubMed ] [ CrossRef ] [ Google Scholar ]
- Davidson R. J. (1988). EEG measures of cerebral asymmetry: conceptual and methodological issues. Int. J. Neurosci. 39 71–89. 10.3109/00207458808985694 [ PubMed ] [ CrossRef ] [ Google Scholar ]
- Davidson R. J. (1992). Emotion and affective style: hemispheric substrates. Psychol. Sci. 3 39–43. 10.1111/j.1467-9280.1992.tb00254.x [ CrossRef ] [ Google Scholar ]
- Davidson R. J. (2004). What does the prefrontal cortex “do” in affect: perspectives on frontal EEG asymmetry research. Biol. Psychol. 67 219–234. 10.1016/j.biopsycho.2004.03.008 [ PubMed ] [ CrossRef ] [ Google Scholar ]
- Davidson R. J., Irwin W. (1999). The functional neuroanatomy of emotion and affective style. Trends Cogn. Sci. 3 11–21. 10.1016/S1364-6613(98)01265-0 [ PubMed ] [ CrossRef ] [ Google Scholar ]
- Dobbins I. G., Foley H., Schacter D. L., Wagner A. D. (2002). Executive control during episodic retrieval: multiple prefrontal processes subserve source memory. Neuron 35 989–996. 10.1016/S0896-6273(02)00858-9 [ PubMed ] [ CrossRef ] [ Google Scholar ]
- Dolcos F., Iordan A. D., Dolcos S. (2011). Neural correlates of emotion–cognition interactions: a review of evidence from brain imaging investigations. J. Cogn. Psychol. 23 669–694. 10.1080/20445911.2011.594433 [ PMC free article ] [ PubMed ] [ CrossRef ] [ Google Scholar ]
- Dolcos F., LaBar K. S., Cabeza R. (2004). Dissociable effects of arousal and valence on prefrontal activity indexing emotional evaluation and subsequent memory: an event-related fMRI study. Neuroimage 23 64–74. 10.1016/j.neuroimage.2004.05.015 [ PubMed ] [ CrossRef ] [ Google Scholar ]
- Dolcos F., LaBar K. S., Cabeza R. (2005). Remembering one year later: role of the amygdala and the medial temporal lobe memory system in retrieving emotional memories. Proc. Natl. Acad. Sci. U.S.A. 102 2626–2631. 10.1073/pnas.0409848102 [ PMC free article ] [ PubMed ] [ CrossRef ] [ Google Scholar ]
- Dolcos F., McCarthy G. (2006). Brain systems mediating cognitive interference by emotional distraction. J. Neurosci. 26 2072–2079. 10.1523/JNEUROSCI.5042-05.2006 [ PMC free article ] [ PubMed ] [ CrossRef ] [ Google Scholar ]
- Durantin G., Gagnon J.-F., Tremblay S., Dehais F. (2014). Using near infrared spectroscopy and heart rate variability to detect mental overload. Behav. Brain Res. 259 16–23. 10.1016/j.bbr.2013.10.042 [ PubMed ] [ CrossRef ] [ Google Scholar ]
- Ehlis A.-C., Schneider S., Dresler T., Fallgatter A. J. (2014). Application of functional near-infrared spectroscopy in psychiatry. Neuroimage 85 478–488. 10.1016/j.neuroimage.2013.03.067 [ PubMed ] [ CrossRef ] [ Google Scholar ]
- Erk S., Kiefer M., Grothe J., Wunderlich A. P., Spitzer M., Walter H. (2003). Emotional context modulates subsequent memory effect. Neuroimage 18 439–447. 10.1016/S1053-8119(02)00015-0 [ PubMed ] [ CrossRef ] [ Google Scholar ]
- Etkin A., Egner T., Kalisch R. (2011). Emotional processing in anterior cingulate and medial prefrontal cortex. Trends Cogn. Sci. 15 85–93. 10.1016/j.tics.2010.11.004 [ PMC free article ] [ PubMed ] [ CrossRef ] [ Google Scholar ]
- Euston D. R., Gruber A. J., McNaughton B. L. (2012). The role of medial prefrontal cortex in memory and decision making. Neuron 76 1057–1070. 10.1016/j.neuron.2012.12.002 [ PMC free article ] [ PubMed ] [ CrossRef ] [ Google Scholar ]
- Friese U., Köster M., Hassler U., Martens U., Trujillo-Barreto N., Gruber T. (2013). Successful memory encoding is associated with increased cross-frequency coupling between frontal theta and posterior gamma oscillations in human scalp-recorded EEG. Neuroimage 66 642–647. 10.1016/j.neuroimage.2012.11.002 [ PubMed ] [ CrossRef ] [ Google Scholar ]
- González-Roldan A. M., Martínez-Jauand M., Muñoz-García M. A., Sitges C., Cifre I., Montoya P. (2011). Temporal dissociation in the brain processing of pain and anger faces with different intensities of emotional expression. Pain 152 853–859. 10.1016/j.pain.2010.12.037 [ PubMed ] [ CrossRef ] [ Google Scholar ]
- Grimshaw G. M., Carmel D. (2014). An asymmetric inhibition model of hemispheric differences in emotional processing. Front. Psychol. 5 : 489 10.3389/fpsyg.2014.00489 [ PMC free article ] [ PubMed ] [ CrossRef ] [ Google Scholar ]
- Haber S. N., Knutson B. (2010). The reward circuit: linking primate anatomy and human imaging. Neuropsychopharmacology 35 4–26. 10.1038/npp.2009.129 [ PMC free article ] [ PubMed ] [ CrossRef ] [ Google Scholar ]
- Hamann S. (2005). Sex differences in the responses of the human amygdala. Neuroscientist 11 288–293. 10.1177/1073858404271981 [ PubMed ] [ CrossRef ] [ Google Scholar ]
- Hamann S., Canli T. (2004). Individual differences in emotion processing. Curr. Opin. Neurobiol. 14 233–238. 10.1016/j.conb.2004.03.010 [ PubMed ] [ CrossRef ] [ Google Scholar ]
- Hamann S., Mao H. (2002). Positive and negative emotional verbal stimuli elicit activity in the left amygdala. Neuroreport 13 15–19. 10.1097/00001756-200201210-00008 [ PubMed ] [ CrossRef ] [ Google Scholar ]
- Harmon-Jones E., Gable P. A., Peterson C. K. (2010). The role of asymmetric frontal cortical activity in emotion-related phenomena: a review and update. Biol. Psychol. 84 451–462. 10.1016/j.biopsycho.2009.08.010 [ PubMed ] [ CrossRef ] [ Google Scholar ]
- Harmon-Jones E., Gable P. A. (2017). On the role of asymmetric frontal cortical activity in approach and withdrawal motivation: an updated review of the evidence. Psychophysiology 10.1111/psyp.12879 [Epub ahead of print]. [ PubMed ] [ CrossRef ] [ Google Scholar ]
- Headley D. B., Paré D. (2013). In sync: gamma oscillations and emotional memory. Front. Behav. Neurosci. 7 : 170 10.3389/fnbeh.2013.00170 [ PMC free article ] [ PubMed ] [ CrossRef ] [ Google Scholar ]
- Heinzel A., Bermpohl F., Niese R., Pfennig A., Pascual-Leone A., Schlaug G., et al. (2005). How do we modulate our emotions? Parametric fMRI reveals cortical midline structures as regions specifically involved in the processing of emotional valences. Cogn. Brain Res. 25 348–358. 10.1016/j.cogbrainres.2005.06.009 [ PubMed ] [ CrossRef ] [ Google Scholar ]
- Hinojosa J. A., Carretié L., Valcárcel M. A., Méndez-Bértolo C., Pozo M. A. (2009). Electrophysiological differences in the processing of affective information in words and pictures. Cogn. Affect. Behav. Neurosci. 9 173–189. 10.3758/CABN.9.2.173 [ PubMed ] [ CrossRef ] [ Google Scholar ]
- Hock C., Mueller-Spahn F., Schuh-Hofer S., Hofmann M., Dirnagl U., Villringer A. (1995). Age dependency of changes in cerebral hemoglobin oxygenation during brain activation: a near-infrared spectroscopy study. J. Cereb. Blood Flow Metab. 15 1103–1108. 10.1038/jcbfm.1995.137 [ PubMed ] [ CrossRef ] [ Google Scholar ]
- Holmes A., Vuilleumier P., Eimer M. (2003). The processing of emotional facial expression is gated by spatial attention: evidence from event-related brain potentials. Cogn. Brain Res. 16 174–184. 10.1016/S0926-6410(02)00268-9 [ PubMed ] [ CrossRef ] [ Google Scholar ]
- Hoshi Y., Huang J., Kohri S., Iguchi Y., Naya M., Okamoto T., et al. (2011). Recognition of human emotions from cerebral blood flow changes in the frontal region: a study with event-related near-infrared spectroscopy. J. Neuroimaging 21 e94–e101. 10.1111/j.1552-6569.2009.00454.x [ PubMed ] [ CrossRef ] [ Google Scholar ]
- Isen A. M., Daubman K. A., Nowicki G. P. (1987). Positive affect facilitates creative problem solving. J. Pers. Soc. Psychol. 52 1122–1131. 10.1037/0022-3514.52.6.1122 [ PubMed ] [ CrossRef ] [ Google Scholar ]
- Jack R. E., Schyns P. G. (2015). The human face as a dynamic tool for social communication. Curr. Biol. 25 R621–R634. 10.1016/j.cub.2015.05.052 [ PubMed ] [ CrossRef ] [ Google Scholar ]
- Joëls M., Karst H., Alfarez D., Heine V. M., Qin Y., Riel E. V., et al. (2004). Effects of chronic stress on structure and cell function in rat hippocampus and hypothalamus. Stress 7 221–231. 10.1080/10253890500070005 [ PubMed ] [ CrossRef ] [ Google Scholar ]
- Jung N., Wranke C., Hamburger K., Knauff M. (2014). How emotions affect logical reasoning: evidence from experiments with mood-manipulated participants, spider phobics, and people with exam anxiety. Front. Psychol. 5 : 570 10.3389/fpsyg.2014.00570 [ PMC free article ] [ PubMed ] [ CrossRef ] [ Google Scholar ]
- Kensinger E. A., Corkin S. (2003). Effect of negative emotional content on working memory and long-term memory. Emotion 3 378–393. 10.1037/1528-3542.3.4.378 [ PubMed ] [ CrossRef ] [ Google Scholar ]
- Kensinger E. A., Corkin S. (2004). Two routes to emotional memory: distinct neural processes for valence and arousal. Proc. Natl. Acad. Sci. U.S.A. 101 3310–3315. 10.1073/pnas.0306408101 [ PMC free article ] [ PubMed ] [ CrossRef ] [ Google Scholar ]
- Kensinger E. A., Schacter D. L. (2006). Processing emotional pictures and words: effects of valence and arousal. Cogn. Affect. Behav. Neurosci. 6 110–126. 10.3758/CABN.6.2.110 [ PubMed ] [ CrossRef ] [ Google Scholar ]
- Khairudin R., Givi M. V., Shahrazad W. W., Nasir R., Halim F. (2011). Effects of emotional contents on explicit memory process. Pertanika J. Soc. Sci. Humanit. 19 17–26. [ Google Scholar ]
- Khairudin R., Nasir R., Halim F., Zainah A., WS W. S., Ismail K., et al. (2012). Emotion and explicit verbal memory: evidence using Malay Lexicon. Asian Soc. Sci. 8 38 . [ Google Scholar ]
- Kleinginna P. R., Jr., Kleinginna A. M. (1981). A categorized list of emotion definitions, with suggestions for a consensual definition. Motiv. Emot. 5 345–379. 10.1007/BF00992553 [ CrossRef ] [ Google Scholar ]
- Koechlin E., Basso G., Pietrini P., Panzer S., Grafman J. (1999). The role of the anterior prefrontal cortex in human cognition. Nature 399 148–151. 10.1038/20178 [ PubMed ] [ CrossRef ] [ Google Scholar ]
- Kok A. (2000). Age-related changes in involuntary and voluntary attention as reflected in components of the event-related potential (ERP). Biol. Psychol. 54 107–143. 10.1016/S0301-0511(00)00054-5 [ PubMed ] [ CrossRef ] [ Google Scholar ]
- Krause C. M., Viemerö V., Rosenqvist A., Sillanmäki L., Åström T. (2000). Relative electroencephalographic desynchronization and synchronization in humans to emotional film content: an analysis of the 4–6, 6–8, 8–10 and 10–12 Hz frequency bands. Neurosci. Lett. 286 9–12. 10.1016/S0304-3940(00)01092-2 [ PubMed ] [ CrossRef ] [ Google Scholar ]
- Lane R. D., Reiman E. M., Bradley M. M., Lang P. J., Ahern G. L., Davidson R. J., et al. (1997). Neuroanatomical correlates of pleasant and unpleasant emotion. Neuropsychologia 35 1437–1444. 10.1016/S0028-3932(97)00070-5 [ PubMed ] [ CrossRef ] [ Google Scholar ]
- Levy B. J., Wagner A. D. (2011). Cognitive control and right ventrolateral prefrontal cortex: reflexive reorienting, motor inhibition, and action updating. Ann. N. Y. Acad. Sci. 1224 40–62. 10.1111/j.1749-6632.2011.05958.x [ PMC free article ] [ PubMed ] [ CrossRef ] [ Google Scholar ]
- Li L., Chen J.-H. (2006). Emotion Recognition Using Physiological Signals Advances in Artificial Reality and Tele-Existence. Berlin: Springer; 437–446. 10.1007/11941354_44 [ CrossRef ] [ Google Scholar ]
- Logothetis N. K., Pauls J., Augath M., Trinath T., Oeltermann A. (2001). Neurophysiological investigation of the basis of the fMRI signal. Nature 412 150–157. 10.1038/35084005 [ PubMed ] [ CrossRef ] [ Google Scholar ]
- Mayberg H. (1997). Limbic-Cortical Dysregulation. The Neuropsychiatry of Limbic and Subcortical Disorders. Washington, DC: American Psychiatric Press; 167–178. [ Google Scholar ]
- McGaugh J. L. (2000). Memory–a century of consolidation. Science 287 248–251. 10.1126/science.287.5451.248 [ PubMed ] [ CrossRef ] [ Google Scholar ]
- McGaugh J. L. (2004). The amygdala modulates the consolidation of memories of emotionally arousing experiences. Annu. Rev. Neurosci. 27 1–28. 10.1146/annurev.neuro.27.070203.144157 [ PubMed ] [ CrossRef ] [ Google Scholar ]
- McGaugh J. L. (2006). Make mild moments memorable: add a little arousal. Trends Cogn. Sci. 10 345–347. 10.1016/j.tics.2006.06.001 [ PubMed ] [ CrossRef ] [ Google Scholar ]
- McGaugh J. L., Cahill L., Roozendaal B. (1996). Involvement of the amygdala in memory storage: interaction with other brain systems. Proc. Natl. Acad. Sci. U.S.A. 93 13508–13514. 10.1073/pnas.93.24.13508 [ PMC free article ] [ PubMed ] [ CrossRef ] [ Google Scholar ]
- McGaugh J. L., Roozendaal B. (2002). Role of adrenal stress hormones in forming lasting memories in the brain. Curr. Opin. Neurobiol. 12 205–210. 10.1016/S0959-4388(02)00306-9 [ PubMed ] [ CrossRef ] [ Google Scholar ]
- Mega M. S., Cummings J. L., Salloway S., Malloy P. (1996). The limbic system: an anatomic, phylogenetic, and clinical perspective. J. Neuropsychiatry Clin. Neurosci. 9 315–330. [ PubMed ] [ Google Scholar ]
- Metcalfe J., Mischel W. (1999). A hot/cool-system analysis of delay of gratification: dynamics of willpower. Psychol. Rev. 106 3–19. 10.1037/0033-295X.106.1.3 [ PubMed ] [ CrossRef ] [ Google Scholar ]
- Miller E. K., Cohen J. D. (2001). An integrative theory of prefrontal cortex function. Annu. Rev. Neurosci. 24 167–202. 10.1146/annurev.neuro.24.1.167 [ PubMed ] [ CrossRef ] [ Google Scholar ]
- Moll J., de Oliveira-Souza R., Bramati I. E., Grafman J. (2002). Functional networks in emotional moral and nonmoral social judgments. Neuroimage 16 696–703. 10.1006/nimg.2002.1118 [ PubMed ] [ CrossRef ] [ Google Scholar ]
- Montag C., Panksepp J. (2017). Primary emotional systems and personality: an evolutionary perspective. Front. Psychol. 8 : 464 10.3389/fpsyg.2017.00464 [ PMC free article ] [ PubMed ] [ CrossRef ] [ Google Scholar ]
- Morris J. S., Öhman A., Dolan R. J. (1998). Conscious and unconscious emotional learning in the human amygdala. Nature 393 467–470. 10.1038/30976 [ PubMed ] [ CrossRef ] [ Google Scholar ]
- Müller M. M., Keil A., Gruber T., Elbert T. (1999). Processing of affective pictures modulates right-hemispheric gamma band EEG activity. Clin. Neurophysiol. 110 1913–1920. 10.1016/S1388-2457(99)00151-0 [ PubMed ] [ CrossRef ] [ Google Scholar ]
- Neisser U. (1963). The imitation of man by machine. Science 139 193–197. 10.1126/science.139.3551.193 [ PubMed ] [ CrossRef ] [ Google Scholar ]
- Northoff G., Heinzel A., Bermpohl F., Niese R., Pfennig A., Pascual-Leone A., et al. (2004). Reciprocal modulation and attenuation in the prefrontal cortex: an fMRI study on emotional–cognitive interaction. Hum. Brain Mapp. 21 202–212. 10.1002/hbm.20002 [ PMC free article ] [ PubMed ] [ CrossRef ] [ Google Scholar ]
- Northoff G., Heinzel A., De Greck M., Bermpohl F., Dobrowolny H., Panksepp J. (2006). Self-referential processing in our brain—a meta-analysis of imaging studies on the self. Neuroimage 31 440–457. 10.1016/j.neuroimage.2005.12.002 [ PubMed ] [ CrossRef ] [ Google Scholar ]
- Northoff G., Richter A., Gessner M., Schlagenhauf F., Fell J., Baumgart F., et al. (2000). Functional dissociation between medial and lateral prefrontal cortical spatiotemporal activation in negative and positive emotions: a combined fMRI/MEG study. Cereb. Cortex 10 93–107. 10.1093/cercor/10.1.93 [ PubMed ] [ CrossRef ] [ Google Scholar ]
- Nunez P., Silberstein R., Cadusch P., Wijesinghe R., Westdorp A., Srinivasan R. (1994). A theoretical and experimental study of high resolution EEG based on surface Laplacians and cortical imaging. Electroencephalogr. Clin. Neurophysiol. 90 40–57. 10.1016/0013-4694(94)90112-0 [ PubMed ] [ CrossRef ] [ Google Scholar ]
- Ochsner K. N., Bunge S. A., Gross J. J., Gabrieli J. D. (2002). Rethinking feelings: an fMRI study of the cognitive regulation of emotion. J. Cogn. Neurosci. 14 1215–1229. 10.1162/089892902760807212 [ PubMed ] [ CrossRef ] [ Google Scholar ]
- Ochsner K. N., Gross J. J. (2005). The cognitive control of emotion. Trends Cogn. Sci. 9 242–249. 10.1016/j.tics.2005.03.010 [ PubMed ] [ CrossRef ] [ Google Scholar ]
- Öhman A., Flykt A., Esteves F. (2001). Emotion drives attention: detecting the snake in the grass. J. Exp. Psychol. 130 466–478. 10.1037/0096-3445.130.3.466 [ PubMed ] [ CrossRef ] [ Google Scholar ]
- Okon-Singer H., Hendler T., Pessoa L., Shackman A. J. (2015). The neurobiology of emotion–cognition interactions: fundamental questions and strategies for future research. Front. Hum. Neurosci. 9 : 58 10.3389/fnhum.2015.00058 [ PMC free article ] [ PubMed ] [ CrossRef ] [ Google Scholar ]
- Oonishi S., Hori S., Hoshi Y., Seiyama A. (2014). Influence of Subjective Happiness on the Prefrontal Brain Activity: An fNIRS Study Oxygen Transport to Tissue XXXVI. Berlin: Springer; 287–293. [ PubMed ] [ Google Scholar ]
- Opialla S., Lutz J., Scherpiet S., Hittmeyer A., Jäncke L., Rufer M., et al. (2015). Neural circuits of emotion regulation: a comparison of mindfulness-based and cognitive reappraisal strategies. Eur. Arch. Psychiatry Clin. Neurosci. 265 45–55. 10.1007/s00406-014-0510-z [ PubMed ] [ CrossRef ] [ Google Scholar ]
- Oudeyer P.-Y., Gottlieb J., Lopes M. (2016). Intrinsic motivation, curiosity, and learning: theory and applications in educational technologies. Prog. Brain Res. 229 257–284. 10.1016/bs.pbr.2016.05.005 [ PubMed ] [ CrossRef ] [ Google Scholar ]
- Ozawa S., Matsuda G., Hiraki K. (2014). Negative emotion modulates prefrontal cortex activity during a working memory task: a NIRS study. Front. Hum. Neurosci. 8 : 46 10.3389/fnhum.2014.00046 [ PMC free article ] [ PubMed ] [ CrossRef ] [ Google Scholar ]
- Panksepp J. (1998). Affective Neuroscience: The Foundations of Human and Animal Emotions. Oxford: Oxford university press. [ Google Scholar ]
- Panksepp J. (2005). Affective consciousness: core emotional feelings in animals and humans. Conscious. Cogn. 14 30–80. 10.1016/j.concog.2004.10.004 [ PubMed ] [ CrossRef ] [ Google Scholar ]
- Panksepp J. (2007). Criteria for basic emotions: is DISGUST a primary “emotion”? Cogn. Emot . 21 1819–1828. 10.1080/02699930701334302 [ CrossRef ] [ Google Scholar ]
- Panksepp J. (2011a). The basic emotional circuits of mammalian brains: do animals have affective lives? Neurosci. Biobehav. Rev . 35 1791–1804. 10.1016/j.neubiorev.2011.08.003 [ PubMed ] [ CrossRef ] [ Google Scholar ]
- Panksepp J. (2011b). Cross-species affective neuroscience decoding of the primal affective experiences of humans and related animals. PLoS ONE 6 : e21236 10.1371/journal.pone.0021236 [ PMC free article ] [ PubMed ] [ CrossRef ] [ Google Scholar ]
- Panksepp J., Normansell L., Cox J. F., Siviy S. M. (1994). Effects of neonatal decortication on the social play of juvenile rats. Physiol. Behav. 56 429–443. 10.1016/0031-9384(94)90285-2 [ PubMed ] [ CrossRef ] [ Google Scholar ]
- Panksepp J., Solms M. (2012). What is neuropsychoanalysis? Clinically relevant studies of the minded brain. Trends Cogn. Sci. 16 6–8. 10.1016/j.tics.2011.11.005 [ PubMed ] [ CrossRef ] [ Google Scholar ]
- Papousek I., Weiss E. M., Schulter G., Fink A., Reiser E. M., Lackner H. K. (2014). Prefrontal EEG alpha asymmetry changes while observing disaster happening to other people: cardiac correlates and prediction of emotional impact. Biol. Psychol. 103 184–194. 10.1016/j.biopsycho.2014.09.001 [ PubMed ] [ CrossRef ] [ Google Scholar ]
- Paré D., Collins D. R., Pelletier J. G. (2002). Amygdala oscillations and the consolidation of emotional memories. Trends Cogn. Sci. 6 306–314. 10.1016/S1364-6613(02)01924-1 [ PubMed ] [ CrossRef ] [ Google Scholar ]
- Payne J. D., Jackson E. D., Hoscheidt S., Ryan L., Jacobs W. J., Nadel L. (2007). Stress administered prior to encoding impairs neutral but enhances emotional long-term episodic memories. Learn. Mem. 14 861–868. 10.1101/lm.743507 [ PMC free article ] [ PubMed ] [ CrossRef ] [ Google Scholar ]
- Pegna A. J., Khateb A., Lazeyras F., Seghier M. L. (2005). Discriminating emotional faces without primary visual cortices involves the right amygdala. Nat. Neurosci. 8 24–25. 10.1038/nn1364 [ PubMed ] [ CrossRef ] [ Google Scholar ]
- Pekrun R. (1992). The impact of emotions on learning and achievement: towards a theory of cognitive/motivational mediators. Appl. Psychol. 41 359–376. 10.1111/j.1464-0597.1992.tb00712.x [ CrossRef ] [ Google Scholar ]
- Perlstein W. M., Elbert T., Stenger V. A. (2002). Dissociation in human prefrontal cortex of affective influences on working memory-related activity. Proc. Natl. Acad. Sci. U.S.A. 99 1736–1741. 10.1073/pnas.241650598 [ PMC free article ] [ PubMed ] [ CrossRef ] [ Google Scholar ]
- Perrey S. (2008). Non-invasive NIR spectroscopy of human brain function during exercise. Methods 45 289–299. 10.1016/j.ymeth.2008.04.005 [ PubMed ] [ CrossRef ] [ Google Scholar ]
- Pessoa L. (2008). On the relationship between emotion and cognition. Nat. Rev. Neurosci. 9 148–158. 10.1038/nrn2317 [ PubMed ] [ CrossRef ] [ Google Scholar ]
- Phelps E. A. (2004). Human emotion and memory: interactions of the amygdala and hippocampal complex. Curr. Opin. Neurobiol. 14 198–202. 10.1016/j.conb.2004.03.015 [ PubMed ] [ CrossRef ] [ Google Scholar ]
- Plichta M. M., Gerdes A. B., Alpers G., Harnisch W., Brill S., Wieser M., et al. (2011). Auditory cortex activation is modulated by emotion: a functional near-infrared spectroscopy (fNIRS) study. Neuroimage 55 1200–1207. 10.1016/j.neuroimage.2011.01.011 [ PubMed ] [ CrossRef ] [ Google Scholar ]
- Poldrack R. A., Wagner A. D., Prull M. W., Desmond J. E., Glover G. H., Gabrieli J. D. (1999). Functional specialization for semantic and phonological processing in the left inferior prefrontal cortex. Neuroimage 10 15–35. 10.1006/nimg.1999.0441 [ PubMed ] [ CrossRef ] [ Google Scholar ]
- Ramnani N., Owen A. M. (2004). Anterior prefrontal cortex: insights into function from anatomy and neuroimaging. Nat. Rev. Neurosci. 5 184–194. 10.1038/nrn1343 [ PubMed ] [ CrossRef ] [ Google Scholar ]
- Richardson M. P., Strange B. A., Dolan R. J. (2004). Encoding of emotional memories depends on amygdala and hippocampus and their interactions. Nat. Neurosci. 7 278–285. 10.1038/nn1190 [ PubMed ] [ CrossRef ] [ Google Scholar ]
- Richter-Levin G., Akirav I. (2000). Amygdala-hippocampus dynamic interaction in relation to memory. Mol. Neurobiol. 22 11–20. 10.1385/MN:22:1-3:011 [ PubMed ] [ CrossRef ] [ Google Scholar ]
- Rolls E. T. (2000). The orbitofrontal cortex and reward. Cereb. Cortex 10 284–294. 10.1093/cercor/10.3.284 [ PubMed ] [ CrossRef ] [ Google Scholar ]
- Russell J. A. (1980). A circumplex model of affect. J. Pers. Soc. Psychol. 39 1161–1178. 10.1037/h0077714 [ CrossRef ] [ Google Scholar ]
- Russell J. A., Bachorowski J.-A., Fernández-Dols J.-M. (2003). Facial and vocal expressions of emotion. Annu. Rev. Psychol. 54 329–349. 10.1146/annurev.psych.54.101601.145102 [ PubMed ] [ CrossRef ] [ Google Scholar ]
- Russell J. A., Barrett L. F. (1999). Core affect, prototypical emotional episodes, and other things called emotion: dissecting the elephant. J. Pers. Soc. Psychol. 76 805–819. 10.1037/0022-3514.76.5.805 [ PubMed ] [ CrossRef ] [ Google Scholar ]
- Rusting C. L. (1998). Personality, mood, and cognitive processing of emotional information: three conceptual frameworks. Psychol. Bull. 124 165–196. 10.1037/0033-2909.124.2.165 [ PubMed ] [ CrossRef ] [ Google Scholar ]
- Rutishauser U., Ross I. B., Mamelak A. N., Schuman E. M. (2010). Human memory strength is predicted by theta-frequency phase-locking of single neurons. Nature 464 903–907. 10.1038/nature08860 [ PubMed ] [ CrossRef ] [ Google Scholar ]
- Schacht A., Sommer W. (2009a). Emotions in word and face processing: early and late cortical responses. Brain Cogn. 69 538–550. 10.1016/j.bandc.2008.11.005 [ PubMed ] [ CrossRef ] [ Google Scholar ]
- Schacht A., Sommer W. (2009b). Time course and task dependence of emotion effects in word processing. Cogn. Affect. Behav. Neurosci. 9 28–43. 10.3758/CABN.9.1.28 [ PubMed ] [ CrossRef ] [ Google Scholar ]
- Schiff N. D., Plum F. (2000). The role of arousal and “gating” systems in the neurology of impaired consciousness. J. Clin. Neurophysiol. 17 438–452. 10.1097/00004691-200009000-00002 [ PubMed ] [ CrossRef ] [ Google Scholar ]
- Schupp H. T., Cuthbert B. N., Bradley M. M., Cacioppo J. T., Ito T., Lang P. J. (2000). Affective picture processing: the late positive potential is modulated by motivational relevance. Psychophysiology 37 257–261. 10.1111/1469-8986.3720257 [ PubMed ] [ CrossRef ] [ Google Scholar ]
- Schupp H. T., Markus J., Weike A. I., Hamm A. O. (2003). Emotional facilitation of sensory processing in the visual cortex. Psychol. Sci. 14 7–13. 10.1111/1467-9280.01411 [ PubMed ] [ CrossRef ] [ Google Scholar ]
- Schupp H. T., Stockburger J., Codispoti M., Junghöfer M., Weike A. I., Hamm A. O. (2007). Selective visual attention to emotion. J. Neurosci. 27 1082–1089. 10.1523/JNEUROSCI.3223-06.2007 [ PMC free article ] [ PubMed ] [ CrossRef ] [ Google Scholar ]
- Schwabe L., Wolf O. T. (2010). Learning under stress impairs memory formation. Neurobiol. Learn. Mem. 93 183–188. 10.1016/j.nlm.2009.09.009 [ PubMed ] [ CrossRef ] [ Google Scholar ]
- Sederberg P. B., Kahana M. J., Howard M. W., Donner E. J., Madsen J. R. (2003). Theta and gamma oscillations during encoding predict subsequent recall. J. Neurosci. 23 10809–10814. [ PMC free article ] [ PubMed ] [ Google Scholar ]
- Seli P., Wammes J. D., Risko E. F., Smilek D. (2016). On the relation between motivation and retention in educational contexts: the role of intentional and unintentional mind wandering. Psychon. Bull. Rev. 23 1280–1287. 10.3758/s13423-015-0979-0 [ PubMed ] [ CrossRef ] [ Google Scholar ]
- Shafer A. T., Dolcos F. (2014). Dissociating retrieval success from incidental encoding activity during emotional memory retrieval, in the medial temporal lobe. Front. Behav. Neurosci. 8 : 177 10.3389/fnbeh.2014.00177 [ PMC free article ] [ PubMed ] [ CrossRef ] [ Google Scholar ]
- Sharot T., Delgado M. R., Phelps E. A. (2004). How emotion enhances the feeling of remembering. Nat. Neurosci. 7 1376–1380. 10.1038/nn1353 [ PubMed ] [ CrossRef ] [ Google Scholar ]
- Sharot T., Phelps E. A. (2004). How arousal modulates memory: disentangling the effects of attention and retention. Cogn. Affect. Behav. Neurosci. 4 294–306. 10.3758/CABN.4.3.294 [ PubMed ] [ CrossRef ] [ Google Scholar ]
- Shen L., Wang M., Shen R. (2009). Affective e-learning: using” Emotional” data to improve learning in pervasive learning environment. Educ. Technol. Soc. 12 176–189. [ Google Scholar ]
- Shigemune Y., Tsukiura T., Nouchi R., Kambara T., Kawashima R. (2017). Neural mechanisms underlying the reward-related enhancement of motivation when remembering episodic memories with high difficulty. Hum. Brain Mapp. 10.1002/hbm.23599 [Epub ahead of print]. [ PMC free article ] [ PubMed ] [ CrossRef ] [ Google Scholar ]
- Simons J. S., Spiers H. J. (2003). Prefrontal and medial temporal lobe interactions in long-term memory. Nat. Rev. Neurosci. 4 637–648. 10.1038/nrn1178 [ PubMed ] [ CrossRef ] [ Google Scholar ]
- Squire L. R. (1992). Memory and the hippocampus: a synthesis from findings with rats, monkeys, and humans. Psychol. Rev. 99 195–231. 10.1037/0033-295X.99.2.195 [ PubMed ] [ CrossRef ] [ Google Scholar ]
- Squire R. F., Noudoost B., Schafer R. J., Moore T. (2013). Prefrontal contributions to visual selective attention. Annu. Rev. Neurosci. 36 451–466. 10.1146/annurev-neuro-062111-150439 [ PubMed ] [ CrossRef ] [ Google Scholar ]
- Talmi D. (2013). Enhanced emotional memory cognitive and neural mechanisms. Curr. Dir. Psychol. Sci. 22 430–436. 10.1177/0963721413498893 [ CrossRef ] [ Google Scholar ]
- Talmi D., Schimmack U., Paterson T., Moscovitch M. (2007). The role of attention and relatedness in emotionally enhanced memory. Emotion 7 89–102. 10.1037/1528-3542.7.1.89 [ PubMed ] [ CrossRef ] [ Google Scholar ]
- Tataranni P. A., Gautier J.-F., Chen K., Uecker A., Bandy D., Salbe A. D., et al. (1999). Neuroanatomical correlates of hunger and satiation in humans using positron emission tomography. Proc. Natl. Acad. Sci. U.S.A. 96 4569–4574. 10.1073/pnas.96.8.4569 [ PMC free article ] [ PubMed ] [ CrossRef ] [ Google Scholar ]
- Taylor S. F., Liberzon I., Fig L. M., Decker L. R., Minoshima S., Koeppe R. A. (1998). The effect of emotional content on visual recognition memory: a PET activation study. Neuroimage 8 188–197. 10.1006/nimg.1998.0356 [ PubMed ] [ CrossRef ] [ Google Scholar ]
- Um E., Plass J. L., Hayward E. O., Homer B. D. (2012). Emotional design in multimedia learning. J. Educ. Psychol. 104 485–498. 10.1037/a0026609 [ CrossRef ] [ Google Scholar ]
- Urry H. L., Gross J. J. (2010). Emotion regulation in older age. Curr. Dir. Psychol. Sci. 19 352–357. 10.1177/0963721410388395 [ CrossRef ] [ Google Scholar ]
- Villringer A., Planck J., Hock C., Schleinkofer L., Dirnagl U. (1993). Near infrared spectroscopy (NIRS): a new tool to study hemodynamic changes during activation of brain function in human adults. Neurosci. Lett. 154 101–104. 10.1016/0304-3940(93)90181-J [ PubMed ] [ CrossRef ] [ Google Scholar ]
- Vogel S., Schwabe L. (2016). Learning and memory under stress: implications for the classroom. Sci. Learn. 1 1–10. 10.1038/npjscilearn.2016.11 [ PMC free article ] [ PubMed ] [ CrossRef ] [ Google Scholar ]
- Volman I., Roelofs K., Koch S., Verhagen L., Toni I. (2011). Anterior prefrontal cortex inhibition impairs control over social emotional actions. Curr. Biol. 21 1766–1770. 10.1016/j.cub.2011.08.050 [ PubMed ] [ CrossRef ] [ Google Scholar ]
- Vuilleumier P. (2005). How brains beware: neural mechanisms of emotional attention. Trends Cogn. Sci. 9 585–594. 10.1016/j.tics.2005.10.011 [ PubMed ] [ CrossRef ] [ Google Scholar ]
- Vytal K., Hamann S. (2010). Neuroimaging support for discrete neural correlates of basic emotions: a voxel-based meta-analysis. J. Cogn. Neurosci. 22 2864–2885. 10.1162/jocn.2009.21366 [ PubMed ] [ CrossRef ] [ Google Scholar ]
- Wager T. D., Davidson M. L., Hughes B. L., Lindquist M. A., Ochsner K. N. (2008). Prefrontal-subcortical pathways mediating successful emotion regulation. Neuron 59 1037–1050. 10.1016/j.neuron.2008.09.006 [ PMC free article ] [ PubMed ] [ CrossRef ] [ Google Scholar ]
- Wagner A. D., Maril A., Bjork R. A., Schacter D. L. (2001). Prefrontal contributions to executive control: fMRI evidence for functional distinctions within lateral prefrontal cortex. Neuroimage 14 1337–1347. 10.1006/nimg.2001.0936 [ PubMed ] [ CrossRef ] [ Google Scholar ]
- Walker M. P. (2009). The role of sleep in cognition and emotion. Ann. N. Y. Acad. Sci. 1156 168–197. 10.1111/j.1749-6632.2009.04416.x [ PubMed ] [ CrossRef ] [ Google Scholar ]
- Watson D., Clark L. A., Tellegen A. (1988). Development and validation of brief measures of positive and negative affect: the PANAS scales. J. Pers. Soc. Psychol. 54 1063–1070. 10.1037/0022-3514.54.6.1063 [ PubMed ] [ CrossRef ] [ Google Scholar ]
- Watt D. F. (2012). “Theoretical challenges in the conceptualization of motivation in neuroscience: Implications for the bridging of neuroscience and psychoanalysis,” in From the Couch to the Lab: Trends in Psychodynamic Neuroscience eds Fotopoulou A., Pfaff D., Conway M. A. (Oxford: Oxford University Press; ). [ Google Scholar ]
- Watt D. F., Pincus D. I. (2004). “Neural substrates of consciousness: implications for clinical psychiatry,”in Textbook of Biological Psychiatry ed. Panksepp J. (Hoboken, NJ: Wiley; ) 75–110. [ Google Scholar ]
- Weymar M., Löw A., Hamm A. O. (2011). Emotional memories are resilient to time: evidence from the parietal ERP old/new effect. Hum. Brain Mapp. 32 632–640. 10.1002/hbm.21051 [ PMC free article ] [ PubMed ] [ CrossRef ] [ Google Scholar ]
- Yamasaki H., LaBar K. S., McCarthy G. (2002). Dissociable prefrontal brain systems for attention and emotion. Proc. Natl. Acad. Sci. U.S.A. 99 11447–11451. 10.1073/pnas.182176499 [ PMC free article ] [ PubMed ] [ CrossRef ] [ Google Scholar ]
- Yiend J. (2010). The effects of emotion on attention: a review of attentional processing of emotional information. Cogn. Emot. 24 3–47. 10.1080/02699930903205698 [ CrossRef ] [ Google Scholar ]
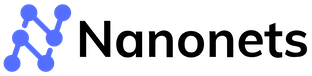
What is Retrieval Augmented Generation (RAG)?
In the AI space, where technological development is happening at a rapid pace, Retrieval Augmented Generation, or RAG, is a game-changer. But what is RAG, and why does it hold such importance in the present AI and natural language processing (NLP) world?
Before answering that question, let's briefly talk about Large Language Models (LLMs) . LLMs, like GPT-3, are AI bots that can generate coherent and relevant text. They learn from the massive amount of text data they read. We all know the ultimate chatbot, ChatGPT, which we have all used to send a mail or two. RAG enhances LLMs by making them more accurate and relevant. RAG steps up the game for LLMs by adding a retrieval step. The easiest way to think of it is like having both a very large library and a very skillful writer in your hands. You interact with RAG by asking it a question; it then utilizes its access to a rich database to mine relevant information and pieces together a coherent and detailed answer with this information. Overall, you get a two-in-one response because it contains both correct data and is full of details. What makes RAG unique? By combining retrieval and generation, RAG models significantly improve the quality of answers AI can provide in many disciplines. Here are some examples:
- Customer Support : Ever been frustrated with a chatbot that gives vague answers? RAG can provide precise and context-aware responses, making customer interactions smoother and more satisfying.
- Healthcare : Think of a doctor accessing up-to-date medical literature in seconds. RAG can quickly retrieve and summarize relevant research, aiding in better medical decisions.
- Insurance : Processing claims can be complex and time-consuming. RAG can swiftly gather and analyze necessary documents and information, streamlining claims processing and improving accuracy
These examples highlight how RAG is transforming industries by enhancing the accuracy and relevance of AI-generated content.
In this blog, we'll dive deeper into the workings of RAG, explore its benefits, and look at real-world applications. We’ll also discuss the challenges it faces and potential areas for future development. By the end, you'll have a solid understanding of Retrieval-Augmented Generation and its transformative potential in the world of AI and NLP. Let's get started!
Looking to build a RAG app tailored to your needs? We've implemented solutions for our customers and can do the same for you. Book a call with us today!
Understanding Retrieval-Augmented Generation
Retrieval-Augmented Generation (RAG) is a smart approach in AI to improve the accuracy and credibility of Generative AI and LLM models by bringing together two key techniques: retrieving information and generating text. Let’s break down how this works and why it’s so valuable.
What is RAG and How Does It Work?
Think of RAG as your personal research assistant. Imagine you’re writing an essay and need to include accurate, up-to-date information. Instead of relying on your memory alone, you use a tool that first looks up the latest facts from a huge library of sources and then writes a detailed answer based on that information. This is what RAG does—it finds the most relevant information and uses it to create well-informed responses.
How Retrieval and Generation Work Together
- Retrieval : First, RAG searches through a vast amount of data to find pieces of information that are most relevant to the question or topic. For example, if you ask about the latest smartphone features, RAG will pull in the most recent articles and reviews about smartphones. This retrieval process often utilizes embeddings and vector databases. Embeddings are numerical representations of data that capture semantic meanings, making it easier to compare and retrieve relevant information from large datasets. Vector databases store these embeddings, allowing the system to efficiently search through vast amounts of information and find the most relevant pieces based on similarity.
- Generation : After retrieving this information, RAG uses a text generation model that relies on deep learning techniques to create a response. The generative model takes the retrieved data and crafts a response that is easy to understand and relevant. So, if you’re looking for information on new phone features, RAG will not only pull the latest data but also explain it in a clear and concise manner.
You might have some questions about how the retrieval step operates and its implications for the overall system. Let’s address a few common doubts:
- Is the Data Static or Dynamic? The data that RAG retrieves can be either static or dynamic. Static data sources remain unchanged over time, while dynamic sources are frequently updated. Understanding the nature of your data sources helps in configuring the retrieval system to ensure it provides the most relevant information. For dynamic data, embeddings and vector databases are regularly updated to reflect new information and trends.
- Who Decides What Data to Retrieve? The retrieval process is configured by developers and data scientists. They select the data sources and define the retrieval mechanisms based on the needs of the application. This configuration determines how the system searches and ranks the information. Developers may also use open-source tools and frameworks to enhance retrieval capabilities, leveraging community-driven improvements and innovations.
- How Is Static Data Kept Up-to-Date? Although static data doesn’t change frequently, it still requires periodic updates. This can be done through re-indexing the data or manual updates to ensure that the retrieved information remains relevant and accurate. Regular re-indexing can involve updating embeddings in the vector database to reflect any changes or additions to the static dataset.
- How Does Static Data Differ from Training Data? Static data used in retrieval is separate from the training data. While training data helps the model learn and generate responses, static data enhances these responses with up-to-date information during the retrieval phase. Training data helps the model learn how to generate clear and relevant responses, while static data keeps the information up-to-date and accurate.
It’s like having a knowledgeable friend who’s always up-to-date and knows how to explain things in a way that makes sense.
What problems does RAG solve
RAG represents a significant leap forward in AI for several reasons. Before RAG, Generative AI models generated responses based on the data they had seen during their training phase. It was like having a friend who was really good at trivia but only knew facts from a few years ago. If you asked them about the latest trends or recent news, they might give you outdated or incomplete information. For example, if you needed information about the latest smartphone release, they could only tell you about phones from previous years, missing out on the newest features and specs.
RAG changes the game by combining the best of both worlds—retrieving up-to-date information and generating responses based on that information. This way, you get answers that are not only accurate but also current and relevant. Let’s talk about why RAG is a big deal in the AI world:
- Enhanced Accuracy : RAG improves the accuracy of AI-generated responses by pulling in specific, up-to-date information before generating text. This reduces errors and ensures that the information provided is precise and reliable.
- Increased Relevance : By using the latest information from its retrieval component, RAG ensures that the responses are relevant and timely. This is particularly important in fast-moving fields like technology and finance, where staying current is crucial.
- Better Context Understanding : RAG can generate responses that make sense in the given context by utilizing relevant data. For example, it can tailor explanations to fit the needs of a student asking about a specific homework problem.
- Reducing AI Hallucinations : AI hallucinations occur when models generate content that sounds plausible but is factually incorrect or nonsensical. Since RAG relies on retrieving factual information from a database, it helps mitigate this problem, leading to more reliable and accurate responses.
Here’s a simple comparison to show how RAG stands out from traditional generative models:
Feature | Traditional Generative Models | Retrieval-Augmented Generation (RAG) |
Information Source | Generates text based on training data alone | Retrieves up-to-date information from a large database |
Accuracy | May produce errors or outdated info | Provides precise and current information |
Relevance | Depends on the model's training | Uses relevant data to ensure answers are timely and useful |
Context Understanding | May lack context-specific details | Uses retrieved data to generate context-aware responses |
Handling AI Hallucinations | Prone to generating incorrect or nonsensical content | Reduces errors by using factual information from retrieval |
In summary, RAG combines retrieval and generation to create AI responses that are accurate, relevant, and contextually appropriate, while also reducing the likelihood of generating incorrect information. Think of it as having a super-smart friend who’s always up-to-date and can explain things clearly. Really convenient, right?
Technical Overview of Retrieval-Augmented Generation (RAG)
In this section, we’ll be diving into the technical aspects of RAG, focusing on its core components, architecture, and implementation.
Key Components of RAG
- BM25 : This model improves the effectiveness of search by ranking documents based on term frequency and document length, making it a powerful tool for retrieving relevant information from large datasets.
- Dense Retrieval : Uses advanced neural network and deep learning techniques to understand and retrieve information based on semantic meaning rather than just keywords. This approach, powered by models like BERT, enhances the relevance of the retrieved content.
- GPT-3 : Known for its ability to produce highly coherent and contextually appropriate text. It generates responses based on the input it receives, leveraging its extensive training data.
- T5 : Converts various NLP tasks into a text-to-text format, which allows it to handle a broad range of text generation tasks effectively.
There are other such models that are available which offer unique strengths and are also widely used in various applications.
How RAG Works: Step-by-Step Flow
- User Input : The process begins when a user submits a query or request.
- Search : The retrieval model (e.g., BM25 or Dense Retrieval) searches through a large dataset to find documents relevant to the query.
- Selection : The most pertinent documents are selected from the search results.
- Input Processing : The selected documents are passed to the generative model (e.g., GPT-3 or T5).
- Response Generation : The generative model creates a coherent response based on the retrieved information and the user’s query.
- Output : The final response is delivered to the user, combining the retrieved data with the generative model’s capabilities.
RAG Architecture
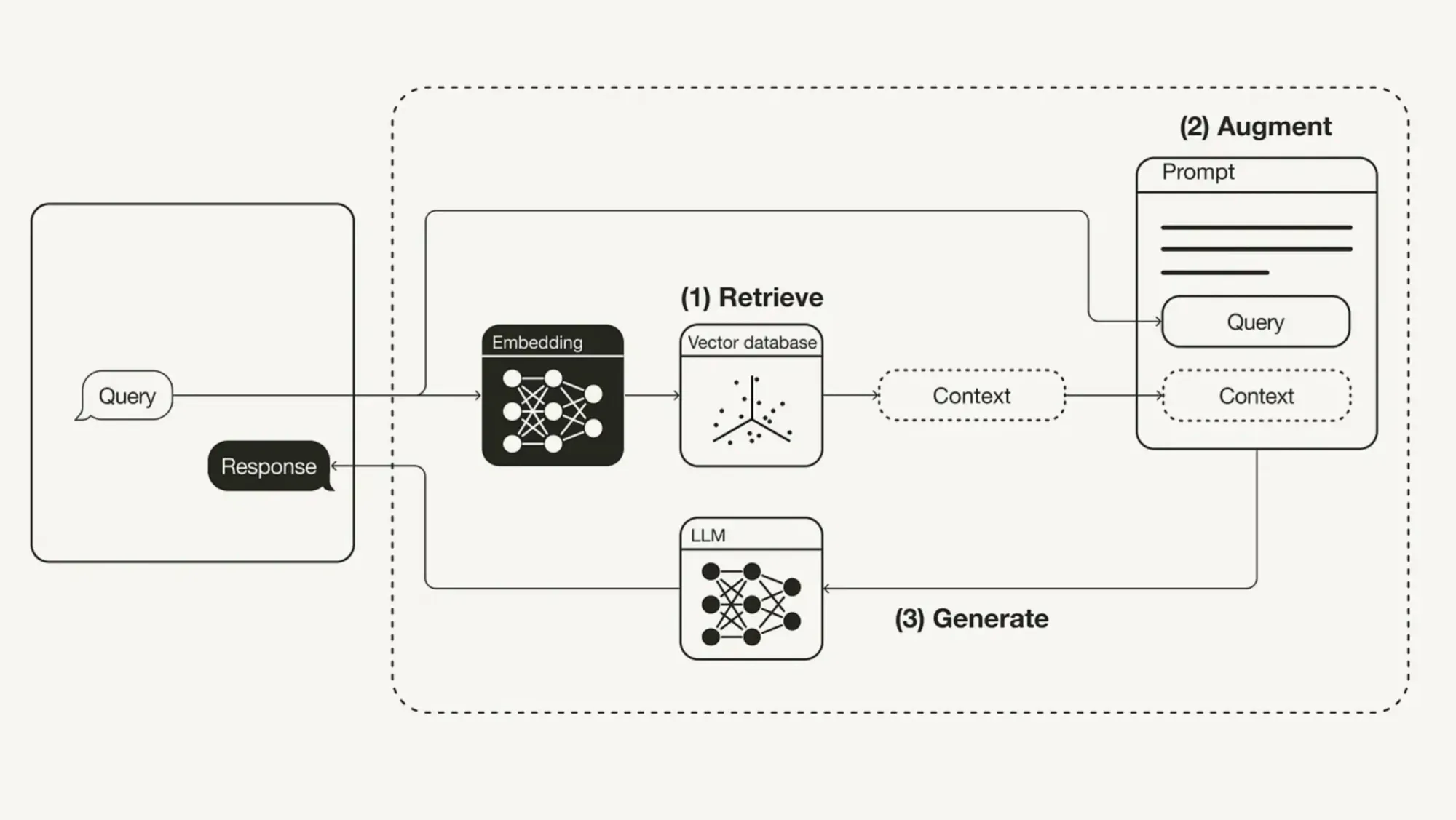
Data flows from the input query to the retrieval component, which extracts relevant information. This data is then passed to the generation component, which creates the final output, ensuring that the response is both accurate and contextually relevant.
Implementing RAG
For practical implementation:
- Hugging Face Transformers : A robust library that simplifies the use of pre-trained models for both retrieval and generation tasks. It provides user-friendly tools and APIs to build and integrate RAG systems efficiently. Additionally, you can find various repositories and resources related to RAG on platforms like GitHub for further customization and implementation guidance.
- LangChain : Another valuable tool for implementing RAG systems. LangChain provides an easy way to manage the interactions between retrieval and generation components, enabling more seamless integration and enhanced functionality for applications utilizing RAG. For more information on LangChain and how it can support your RAG projects, check out our detailed blog post here .
For a comprehensive guide on setting up your own RAG system, check out our blog, "Building a Retrieval-Augmented Generation (RAG) App: A Step-by-Step Tutorial", which offers detailed instructions and example code.
Applications of Retrieval-Augmented Generation (RAG)
Retrieval-Augmented Generation (RAG) isn’t just a fancy term—it’s a transformative technology with practical applications across various fields. Let’s dive into how RAG is making a difference in different industries and some real-world examples that showcase its potential and AI applications.
Industry-Specific Applications
Customer Support Imagine chatting with a support bot that actually understands your problem and gives you spot-on answers. RAG enhances customer support by pulling in precise information from vast databases, allowing chatbots to provide more accurate and contextually relevant responses. No more vague answers or repeated searches; just quick, helpful solutions.
Content Creation Content creators know the struggle of finding just the right information quickly. RAG helps by generating content that is not only contextually accurate but also relevant to current trends. Whether it’s drafting blog posts, creating marketing copy, or writing reports, RAG assists in producing high-quality, targeted content efficiently.
Healthcare In healthcare, timely and accurate information can be a game-changer. RAG can assist doctors and medical professionals by retrieving and summarizing the latest research and treatment guidelines. . This makes RAG highly effective in domain-specific fields like medicine, where staying updated with the latest advancements is crucial.
Education Think of RAG as a supercharged tutor. It can tailor educational content to each student’s needs by retrieving relevant information and generating explanations that match their learning style. From personalized tutoring sessions to interactive learning materials, RAG makes education more engaging and effective.
Implementing a RAG App is one option. Another is getting on a call with us so we can help create a tailored solution for your RAG needs. Discover how Nanonets can automate customer support workflows using custom AI and RAG models.
Automate your customer support using Nanonets' RAG models
Automated FAQ Generation Ever visited a website with a comprehensive FAQ section that seemed to answer every possible question? RAG can automate the creation of these FAQs by analyzing a knowledge base and generating accurate responses to common questions. This saves time and ensures that users get consistent, reliable information.
Document Management Managing a vast array of documents within an enterprise can be daunting. RAG systems can automatically categorize, summarize, and tag documents, making it easier for employees to find and utilize the information they need. This enhances productivity and ensures that critical documents are accessible when needed.
Financial Data Analysis In the financial sector, RAG can be used to sift through financial reports, market analyses, and economic data. It can generate summaries and insights that help financial analysts and advisors make informed investment decisions and provide accurate recommendations to clients.
Research Assistance Researchers often spend hours sifting through data to find relevant information. RAG can streamline this process by retrieving and summarizing research papers and articles, helping researchers quickly gather insights and stay focused on their core work.
Best Practices and Challenges in Implementing RAG
In this final section, we’ll look at the best practices for implementing Retrieval-Augmented Generation (RAG) effectively and discuss some of the challenges you might face.
Best Practices
- Data Quality Ensuring high-quality data for retrieval is crucial. Poor-quality data leads to poor-quality responses. Always use clean, well-organized data to feed into your retrieval models. Think of it as cooking—you can’t make a great dish with bad ingredients.
- Model Training Training your retrieval and generative models effectively is key to getting the best results. Use a diverse and extensive dataset to train your models so they can handle a wide range of queries. Regularly update the training data to keep the models current.
- Evaluation and Fine-Tuning Regularly evaluate the performance of your RAG models and fine-tune them as necessary. Use metrics like precision, recall, and F1 score to gauge accuracy and relevance. Fine-tuning helps in ironing out any inconsistencies and improving overall performance.
- Handling Large Datasets Managing and retrieving data from large datasets can be challenging. Efficient indexing and retrieval techniques are essential to ensure quick and accurate responses. An analogy here can be finding a book in a massive library—you need a good catalog system.
- Contextual Relevance Ensuring that the generated responses are contextually relevant and accurate is another challenge. Sometimes, the models might generate responses that are off the mark. Continuous monitoring and tweaking are necessary to maintain relevance.
- Computational Resources RAG models, especially those utilizing deep learning, require significant computational resources, which can be expensive and demanding. Efficient resource management and optimization techniques are essential to keep the system running smoothly without breaking the bank.
Recap of Key Points: We’ve explored the fundamentals of RAG, its technical overview, applications, and best practices and challenges in implementation. RAG’s ability to combine retrieval and generation makes it a powerful tool in enhancing the accuracy and relevance of AI-generated content.
The future of RAG is bright, with ongoing research and development promising even more advanced models and techniques. As RAG continues to evolve, we can expect even more accurate and contextually aware AI systems.
Found the blog informative? Have a specific use case for building a RAG solution? Our experts at Nanonets can help you craft a tailored and efficient solution. Schedule a call with us today to get started!
Related content
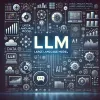
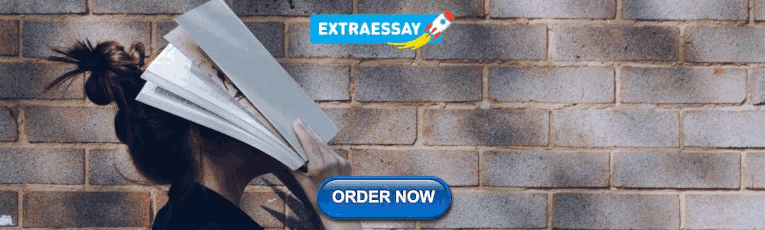
IMAGES
COMMENTS
Abstract. Memory retrieval involves the interaction between external sensory or internally generated cues and stored memory traces (or engrams) in a process termed 'ecphory'. While ecphory has been examined in human cognitive neuroscience research, its neurobiological foundation is less understood. To the extent that ecphory involves ...
Recall: This type of memory retrieval involves being able to access the information without being cued. Answering a question on a fill-in-the-blank test is a good example of recall. Recollection: This type of memory retrieval involves reconstructing memory, often utilizing logical structures, partial memories, narratives, or clues. For example, writing an answer on an essay exam often involves ...
Nature Reviews Psychology (2023) Accurately retrieving information from memory boosts later retrieval. However, retrieving memories can also open a window to errors when erroneous information is ...
Memory is the term given to the structures and processes involved in the storage and subsequent retrieval of information. Memory is essential to all our lives. Without a memory of the past, we cannot operate in the present or think about the future. We would not be able to remember what we did yesterday, what we have done today, or what we plan ...
This paper explores memory from a cognitive neuroscience perspective and examines associated neural mechanisms. It examines the different types of memory: working, declarative, and non-declarative, and the brain regions involved in each type. The paper highlights the role of different brain regions, such as the prefrontal cortex in working ...
Memories are stored in long-term memory and retrieved through activation by retrieval cues. Retrieval fails for several reasons, like context mismatch, overgrown pathways, and interference. We can ...
There are three main processes that characterize how memory works. These processes are encoding, storage, and retrieval (or recall). Encoding . Encoding refers to the process through which information is learned. That is, how information is taken in, understood, and altered to better support storage (which you will look at in Section 3.1.2).
Retrieving a memory seems to involve a set of distinct processes, with different neural substrates, that are coordinated to orchestrate the act of remembering. Recent advances in cognitive science ...
Abstract. Memory retrieval involves the interaction between external sensory or internally generated cues and stored memory traces (or engrams) in a process termed 'ecphory'. While ecphory has ...
Memory is the process of retaining of knowledge over a period for the function of affecting future actions. It can be divided into declarative and procedural types. The process of memory consolidation is done in the hippocampus. The long-term memories are spread among various areas of the cerebrum depending on the different perceptual ...
Spontaneous Retrieval in Prospective Memory. In J. S. Nairne (Ed.), The foundations of remembering: Essays in honor of Henry L. Roediger, III (pp. 225-240). Psychology Press. Abstract. This chapter focuses on episodic memory. The authors take a broad view that episodic memory also allows people to mentally place themselves forward in time.
There are three ways you can retrieve information out of your long-term memory storage system: recall, recognition, and relearning. Recall is what we most often think about when we talk about memory retrieval: it means you can access information without cues. For example, you would use recall for an essay test.
After the encoding process information is stored so that it can be retrieved when needed (Brem, Ran & Pascual-leone, 2013). This essay will explore the three different types of memory mentioned earlier and how the encoding, storage and retrieval model explains the process of forming, maintaining and recalling memories.
Retrieval cues can be external, such as an image, text, a scent, or some other stimulus that relates to the memory. They can also be internal, such as a thought or sensation that is relevant to ...
We outline a theoretical framework encompassing the relationship between encoding and retrieval processes in episodic memory. There are no cortical regions or networks that are specialized for encoding; rather, successful encoding depends on the same regions that are engaged during on-line processing.
Memory is the encoding, storage, and retrieval in the human mind of past experiences. The basic pattern of remembering involves attention to an event followed by representation of that event in the brain. Repeated attention, or practice, enables activities such as playing a musical instrument or recitation of a poem.
Abstract. One of the key goals of memory research is to develop a basic understanding of the nature and characteristics of memory processes and systems. Another important goal is to develop useful applications of basic research to everyday life. This editorial considers two lines of work that illustrate some of the prospects for applying memory ...
Retrieval practice is a learning technique that repeatedly has been shown to enhance long-term retention when compared to other methods of learning, such as re-reading (Roediger & Karpicke, 2006a; Wiklund-Hörnqvist et al., 2014), group discussions (Stenlund et al., 2017), and concept mapping (Karpicke & Blunt, 2011).This retrieval-based benefit on long-term learning is commonly denoted as the ...
Decay Theory of Forgetting. According to the trace theory of memory, physical and chemical changes in the brain results in a memory "trace." Information in short-term memory lasts several seconds and if it is not rehearsed, the neurochemical memory trace quickly fades. According to the trace decay theory of forgetting, the events that happen ...
Memory of episodes (events) is at the core of psychological research on memory. This type of memory encompasses events that an individual has experienced and thus is a collection of experiences that occurred at a specific time and place (Tulving, Citation 1972, Citation 1983, Citation 2002).The retrieval of information is typically different for verbally encoded events versus those encoded in ...
Decent Essays. 686 Words. 3 Pages. Open Document. Memory retrieval is likely to be good after repeated testing of that material. In fact, practising retrieval has a larger effect on memory than revising the information (Hockley, 2009). Another factor that influences the quality of retrieved information is the way one studied the material.
c. Psychologists describe memory as. Multiple choice question. the process of focusing on some stimuli while ignoring others. the retention of information or experience over time. the process by which information comes out of storage. the process by which information gets into storage. b.
Right VLPFC supports memory encoding and retrieval of visuospatial stimuli, action imitation and motor inhibition (Levy and Wagner, 2011). Inhibition of distracting emotions (right VLPFC for inhibition of negative emotions) (Dolcos and McCarthy, 2006). mPFC: 25, 32: Learning, memory, and decision-making (Euston et al., 2012; Brod et al., 2013).
Retrieval-Augmented Generation (RAG) is a smart approach in AI to improve the accuracy and credibility of Generative AI and LLM models. ... Imagine you're writing an essay and need to include accurate, up-to-date information. Instead of relying on your memory alone, you use a tool that first looks up the latest facts from a huge library of ...