Mental Representations and Their Analysis: An Epistemological Perspective
- First Online: 31 December 2009
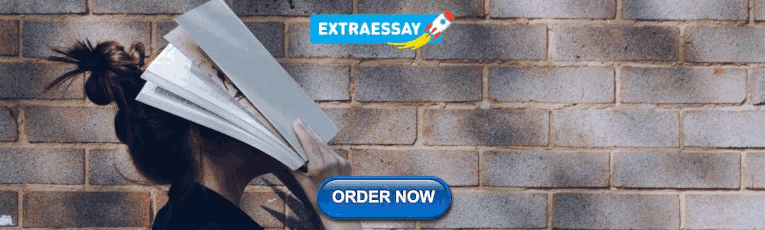
Cite this chapter
- J. Michael Spector 4
862 Accesses
11 Citations
It is widely accepted that mental representations (e.g., mental models and other internal cognitive structures) play a key role in the development of knowledge and expertise. This is especially true in problem-solving domains that involve many interacting components (e.g., complex dynamic systems). However, mental representations are not directly observable. In order to help improve understanding (knowledge and performance) in these domains, it is useful to have a good sense of how an individual is thinking about the problem situation. More specifically, understanding mental model development and formation can improve learning and instruction. Meaningful formative feedback often depends on the ability to identify faulty or incomplete or inappropriate internal representations of one or more aspects of the problem situation. In order to provide such feedback in a timely manner, it is necessary to have methods and tools that reliably reveal a learner’s relevant mental representations. Such methods and tools used to assess mental models are the primary focus in this volume. In this chapter, the focus is on the epistemological status of mental models and their analysis. The specific questions addressed herein are as follows: (a) What can we know about mental representations? (b) Can mental models be reliably assessed? and, (c) How useful are mental model measures in facilitating the development of knowledge and expertise? These are large questions that are not likely to have complete and definitive answers in the near future. The discussion in this chapter should be regarded only as a modest attempt to encourage further dialogue and investigation.
This is a preview of subscription content, log in via an institution to check access.
Access this chapter
- Available as PDF
- Read on any device
- Instant download
- Own it forever
- Available as EPUB and PDF
- Compact, lightweight edition
- Dispatched in 3 to 5 business days
- Free shipping worldwide - see info
- Durable hardcover edition
Tax calculation will be finalised at checkout
Purchases are for personal use only
Institutional subscriptions
Anderson, J. L. (2007). How can the human mind occur in the physical world ? New York: Oxford University Press.
Google Scholar
Bloom, B. S. (Ed.). (1956). Taxonomy of educational objectives, Handbook 1: The cognitive domain . New York: McKay.
Bransford, J. D., Brown, A. L., & Cocking, R. R. (Eds.). (2000). How people learn: Brain, mind, experience, and school . Washington, DC: National Academy Press.
Cronbach, L. J., & Meehl, P. E. (1955). Construct validity in psychological tests. Psychological Bulletin, 52 , 281–302.
Article Google Scholar
Dreyfus, H. L., & Dreyfus, S. E. (1986). Mind over machine: The power of human intuition and expertise in the era of the computer . New York: Free Press.
Ericsson, K. A. (2001). Attaining excellence through deliberate practice: Insights from the study of expert performance. In M. Ferrari (Ed.), The pursuit of excellence in education (pp. 21–55). Mahwah, NJ: Erlbaum.
Ericsson, K. A., & Simon, H. A. (1993). Protocol analysis: Verbal reports as data (Rev. ed.). Cambridge, MA: MIT Press.
Ericsson, K. A., & Smith, J. (1991). Prospects and limits in the empirical study of expertise: An introduction. In K. A. Ericsson & J. Smith (Eds.), Toward a general theory of expertise: Prospects and limits (pp. 1–38). Cambridge: Cambridge University Press.
Gagné, R. M. (1985). The conditions of learning (4th ed.). New York: Holt, Rinehart, and Winston.
Gagné, R. M., & Merrill, M. D. (1990). Integrative goals for instructional design. Educational Technology Research and Development, 38 (1), 23–40.
Ifenthaler, D. (2007, October). Relational, structural, and semantic analysis of graphical representations and concept maps. Introducing the SMD-technology . Paper presented at the Annual Meeting of the Association for Educational Communications and Technology, Anaheim, CA.
Johnson-Laird, P. N. (1983). Mental models . Cambridge, MA: Harvard University Press.
Jonassen, D. H. (2000). Toward a design theory of problem solving. Educational Technology Research & Development, 48 , 63–85.
Klein, G. A., Orsanu, J., Calderwood, R., & Zsambok, C. E. (1993). Decision making in action: Models and methods . Norwood, NJ: Ablex.
Kim, H. (2008). An investigation of the effects of model-centered instruction in individual and collaborative contexts: The case of acquiring instructional design expertise . Unpublished dissertation, Florida State University, Tallahassee, FL.
Lee, J. (2008). Effects of model-centered instruction and levels of expertise on ill-structured problem solving . Unpublished dissertation, Florida State University, Tallahassee, FL.
McKeown, J. (2008). Using annotated concept map assessments as predictors of performance and understanding of complex problems for teacher technology integration . Unpublished dissertation, Florida State University, Tallahassee, FL.
Merrill, M. D. (1993). An integrated model for automating instructional design and delivery. In J. M. Spector, M. C. Polson, & D. J. Muraida (Eds.), Automating instructional design: Concepts and issues (pp. 147–190). Englewood Cliffs, NJ: Educational Technology.
Olson, S., & Loucks-Horsley, S. (2000). Inquiry and the national science education standards: A guide for teaching and learning . Washington, DC: National Academies Press.
Pirnay-Dummer, P. (2007, April). Model inspection trace of concepts and relations. A heuristic approach to language-oriented model assessment . Paper presented at the annual meeting of the American Educational Research Association, Chicago, IL.
Pirnay-Dummer, P., Ifenthaler, D., & Spector, J. M. (2009). Highly integrated model assessment technology and tools. Educational Technology Research and Development , 57 (6).
Rittel, H., & Webber, M. (1973). Dilemmas in a general theory of planning. Policy Sciences, 4 , 155–169.
Seel, N. M. (2001). Epistemology, situated cognition, and mental models: “Like a bridge over troubled water”. Instructional Science , 29 (4–5), 403–427.
Spector, J. M. (2004). Current issues in new learning. In K. Morgan, C. A. Brebbia, J. Sanchez, & A. Voiskounsky (Eds.), Human perspectives in the Internet society: Culture, psychology and gender (pp. 429–440). Southampton, UK: WIT Press.
Spector, J. M. (2006). Introduction to the special issue on models, simulations and learning in complex domains. Technology, Instruction, Cognition and Learning, 3 (3–4), 199–204.
Spector, J. M., & Koszalka, T. A. (2004). The DEEP methodology for assessing learning incomplex domains (Final report to the National Science Foundation Evaluative Research and Evaluation Capacity Building). Syracuse, NY: Syracuse University.
Spector, J. M., & Merrill, M. D. (2008). Editorial: Effective, efficient and engaging (E 3 ) learning in the digital age. Distance Education, 29 (2), 123–126.
Taricani, E. M., & Clariana, R. B. (2006). A technique for automatically scoring open-ended concept maps. Eduational Technology Research and Development, 54 (1), 65–82.
van Merriënboer, J. J. G., & Dijkstra, S. (1997). The four-component instructional-design model for training complex cognitive skills. In R. D. Tennyson, F. Schott, N. Seel, & S. Dijkstra (Eds.), Instructional design: International perspectives (Vol. 1, pp. 427–446). Mahwah, NJ: Lawrence Erlbaum.
van Merriënboer, J. J. G., & Kirschner, P. A. (2007). Ten steps to complex learning . Mahwah, NJ: Lawrence Erlbaum.
Wittgenstein, L. (1922). Tractatus logico-philosophicus (C. K. Ogden Trans.). London: Routledge & Kegan Paul.
Wittgenstein, L. (1953). Philosophical investigations . London: Blackwell.
Download references
Author information
Authors and affiliations.
Learning and Performance Support Laboratory, University of Georgia, Athens, GA, USA
J. Michael Spector
You can also search for this author in PubMed Google Scholar
Corresponding author
Correspondence to J. Michael Spector .
Editor information
Editors and affiliations.
Abt. Lernforschung, Universität Freiburg, Rempartstr. 11, Freiburg, 79085, Germany
Dirk Ifenthaler
Pablo Pirnay-Dummer
Inst. Erziehungswissenschaft, Univ. Freiburg, Freiburg, 79085, Germany
Norbert M. Seel
Rights and permissions
Reprints and permissions
Copyright information
© 2010 Springer Science+Business Media, LLC
About this chapter
Spector, J.M. (2010). Mental Representations and Their Analysis: An Epistemological Perspective. In: Ifenthaler, D., Pirnay-Dummer, P., Seel, N. (eds) Computer-Based Diagnostics and Systematic Analysis of Knowledge. Springer, Boston, MA. https://doi.org/10.1007/978-1-4419-5662-0_3
Download citation
DOI : https://doi.org/10.1007/978-1-4419-5662-0_3
Published : 31 December 2009
Publisher Name : Springer, Boston, MA
Print ISBN : 978-1-4419-5661-3
Online ISBN : 978-1-4419-5662-0
eBook Packages : Humanities, Social Sciences and Law Education (R0)
Share this chapter
Anyone you share the following link with will be able to read this content:
Sorry, a shareable link is not currently available for this article.
Provided by the Springer Nature SharedIt content-sharing initiative
- Publish with us
Policies and ethics
- Find a journal
- Track your research

- Table of Contents
- Random Entry
- Chronological
- Editorial Information
- About the SEP
- Editorial Board
- How to Cite the SEP
- Special Characters
- Advanced Tools
- Support the SEP
- PDFs for SEP Friends
- Make a Donation
- SEPIA for Libraries
- Entry Contents
Bibliography
Academic tools.
- Friends PDF Preview
- Author and Citation Info
- Back to Top
Mental Representation
The notion of a “mental representation” is, arguably, in the first instance a theoretical construct of cognitive science. As such, it is a basic concept of the Computational Theory of Mind, according to which cognitive states and processes are constituted by the occurrence, transformation and storage (in the mind/brain) of information-bearing structures (representations) of one kind or another.
However, on the assumption that a representation is an object with semantic properties (content, reference, truth-conditions, truth-value, etc.), a mental representation may be more broadly construed as a mental object with semantic properties. As such, mental representations (and the states and processes that involve them) need not be understood only in cognitive/computational terms. On this broader construal, mental representation is a philosophical topic with roots in antiquity and a rich history and literature predating the recent “cognitive revolution,” and which continues to be of interest in pure philosophy. Though most contemporary philosophers of mind acknowledge the relevance and importance of cognitive science, they vary in their degree of engagement with its literature, methods and results; and there remain, for many, issues concerning the representational properties of the mind that can be addressed independently of the computational hypothesis.
Though the term ‘Representational Theory of Mind’ is sometimes used almost interchangeably with ‘Computational Theory of Mind’, I will use it here to refer to any theory that postulates the existence of semantically evaluable mental objects, including philosophy’s stock-in-trade mentalia – thoughts, concepts, percepts, ideas, impressions, notions, rules, schemas, images, phantasms, etc. – as well as the various sorts of “subpersonal” representations postulated by cognitive science. Representational theories may thus be contrasted with theories, such as those of Baker (1995), Collins (1987), Dennett (1987), Gibson (1966, 1979), Reid (1764/1997), Stich (1983) and Thau (2002), which deny the existence of such things.
1. The Representational Theory of Mind
2. propositional attitudes.
- 3. Conceptual and Nonconceptual Representation
4. Representationalism and Phenomenalism
6. content determination, 7. internalism and externalism, 8. the computational theory of mind, 9. thought and language, other internet resources, related entries.
The Representational Theory of Mind (RTM) (which goes back at least to Aristotle) takes as its starting point commonsense mental states, such as thoughts, beliefs, desires, perceptions and imagings. Such states are said to have “intentionality” – they are about or refer to things, and may be evaluated with respect to properties like consistency, truth, appropriateness and accuracy. (For example, the thought that cousins are not related is inconsistent, the belief that Elvis is dead is true, the desire to eat the moon is inappropriate, a visual experience of a ripe strawberry as red is accurate, an imaging of George Washington with dreadlocks is inaccurate.)
RTM defines such intentional mental states as relations to mental representations, and explains the intentionality of the former in terms of the semantic properties of the latter. For example, to believe that Elvis is dead is to be appropriately related to a mental representation whose propositional content is that Elvis is dead . (The desire that Elvis be dead, the fear that he is dead, the regret that he is dead, etc., involve different relations to the same mental representation.) To perceive a strawberry is, on the representational view, to have a sensory experience of some kind which is appropriately related to (e.g., caused by) the strawberry.
RTM also understands mental processes such as thinking, reasoning and imagining as sequences of intentional mental states. For example, to imagine the moon rising over a mountain is, inter alia , to entertain a series of mental images of the moon (and a mountain). To infer a proposition q from the propositions p and if p then q is ( inter alia ) to have a sequence of thoughts of the form p , if p then q , q .
Contemporary philosophers of mind have typically supposed (or at least hoped ) that the mind can be naturalized – i.e., that all mental facts have explanations in the terms of natural science. This assumption is shared within cognitive science, which attempts to provide accounts of mental states and processes in terms (ultimately) of features of the brain and central nervous system. In the course of doing so, the various sub-disciplines of cognitive science (including cognitive and computational psychology and cognitive and computational neuroscience) postulate a number of different kinds of structures and processes, many of which are not directly implicated by mental states and processes as commonsensically conceived. There remains, however, a shared commitment to the idea that mental states and processes are to be explained in terms of mental representations.
In philosophy, recent debates about mental representation have centered around the existence of propositional attitudes (beliefs, desires, etc.) and the determination of their contents (how they come to be about what they are about), and the existence of phenomenal properties and their relation to the content of thought and perceptual experience. Within cognitive science itself, the philosophically relevant debates have been focused on the computational architecture of the brain and central nervous system, and the compatibility of scientific and commonsense accounts of mentality.
Intentional Realists such as Dretske (e.g., 1988) and Fodor (e.g., 1987) note that the generalizations we apply in everyday life in predicting and explaining each other’s behavior (often collectively referred to as “folk psychology”) are both remarkably successful and indispensable. What a person believes, doubts, desires, fears, etc. is a highly reliable indicator of what that person will do; and we have no other way of making sense of each other’s behavior than by ascribing such states and applying the relevant generalizations. We are thus committed to the basic truth of commonsense psychology and, hence, to the existence of the states its generalizations refer to. (Some realists, such as Fodor, also hold that commonsense psychology will be vindicated by cognitive science, given that propositional attitudes can be construed as computational relations to mental representations.)
Intentional Eliminativists , such as Churchland, (perhaps) Dennett and (at one time) Stich argue that no such things as propositional attitudes (and their constituent representational states) are implicated by the successful explanation and prediction of our mental lives and behavior. Churchland (1981) denies that the generalizations of commonsense propositional-attitude psychology are true. He argues that folk psychology is a theory of the mind with a long history of failure and decline, and that it resists incorporation into the framework of modern scientific theories (including cognitive psychology). As such, it is comparable to alchemy and phlogiston theory, and ought to suffer a comparable fate. Commonsense psychology is false , and the states (and representations) it postulates simply don’t exist. (It should be noted that Churchland is not an eliminativist about mental representation tout court . See, e.g., Churchland 1989.)
Dennett (1987a) grants that the generalizations of commonsense psychology are true and indispensable, but denies that this is sufficient reason to believe in the entities they appear to refer to. He argues that to give an intentional explanation of a system’s behavior is merely to adopt the “intentional stance” toward it. If the strategy of assigning contentful states to a system and predicting and explaining its behavior (on the assumption that it is rational – i.e., that it behaves as it should, given the propositional attitudes it should have, given its environment) is successful, then the system is intentional, and the propositional-attitude generalizations we apply to it are true. But there is nothing more to having a propositional attitude than this. (See Dennett 1987a: 29.)
Though he has been taken to be thus claiming that intentional explanations should be construed instrumentally, Dennett (1991) insists that he is a “moderate” realist about propositional attitudes, since he believes that the patterns in the behavior and behavioral dispositions of a system on the basis of which we (truly) attribute intentional states to it are objectively real. In the event that there are two or more explanatorily adequate but substantially different systems of intentional ascriptions to an individual, however, Dennett claims there is no fact of the matter about what the individual believes (1987b, 1991). This does suggest an irrealism at least with respect to the sorts of things Fodor and Dretske take beliefs to be; though it is not the view that there is simply nothing in the world that makes intentional explanations true.
(Davidson 1973, 1974 and Lewis 1974 also defend the view that what it is to have a propositional attitude is just to be interpretable in a particular way. It is, however, not entirely clear whether they intend their views to imply irrealism about propositional attitudes.)
Stich (1983) argues that cognitive psychology does not (or, in any case, should not) taxonomize mental states by their semantic properties at all, since attribution of psychological states by content is sensitive to factors that render it problematic in the context of a scientific psychology. Cognitive psychology seeks causal explanations of behavior and cognition, and the causal powers of a mental state are determined by its intrinsic “structural” or “syntactic” properties. The semantic properties of a mental state, however, are determined by its extrinsic properties – e.g., its history, environmental or intramental relations. Hence, such properties cannot figure in causal-scientific explanations of behavior. (Fodor 1994 and Dretske 1988 are realist attempts to come to grips with some of these problems.) Stich proposes a syntactic theory of the mind, on which the semantic properties of mental states play no explanatory role. (Stich has since changed his views on a number of these issues. See Stich 1996.)
3. Conceptual and Non-Conceptual Representation
It is a traditional assumption among realists about mental representations that representational states come in two basic varieties (cf. Boghossian 1995). There are those, such as thoughts, that are composed of concepts and have no phenomenal (“what-it’s-like”) features (“qualia”), and those, such as sensations, which have phenomenal features but no conceptual constituents. (Nonconceptual content is usually defined as a kind of content that states of a creature lacking concepts might nonetheless have. [ 1 ] ) On this taxonomy, mental states can represent either in a way analogous to expressions of natural languages or in a way analogous to drawings, paintings, maps, photographs or movies. Perceptual states such as seeing that something is blue, are sometimes thought of as hybrid states, consisting of, for example, a non-conceptual sensory experience and a belief, or some more integrated compound of conceptual and non-conceptual elements. (There is an extensive literature on the representational content of perceptual experience. See the entry on the contents of perception .)
Disagreement over non-conceptual representation concerns the existence and nature of phenomenal properties, the role they play in determining the contents of sensory representations, and which kinds of properties can be represented by non-conceptual states. Dennett (1988), for example, denies that there are such things as qualia at all (as they are standardly construed); while Brandom (2002), McDowell (1994), Rey (1991) and Sellars (1956) deny that they are needed to explain the content of sensory experience. Among those who accept that experiences have phenomenal content, some (Dretske, Lycan, Tye) argue that it is reducible to a kind of intentional content, while others (Block, Loar, Peacocke) argue that it is irreducible. (See the discussion in the next section.) A further debate concerns the non-conceptual representability of high-level properties such as kind properties and moral properties. (See, e.g., Dretske 1995 and Siegel 2010, and the entry on the contents of perception.)
Some historical discussions of the representational properties of mind (e.g., Aristotle De Anima , Locke 1689/1975, Hume 1739/1978) seem to assume that nonconceptual representations – percepts (“impressions”), images (“ideas”) and the like – are the only (or at least the main) kinds of mental representations, and that the mind represents the world in virtue of being in states that resemble things in it. On such a view, all representational states have their content in virtue of their sensory phenomenal features. Powerful arguments, however, focusing on the lack of generality (Berkeley Principles of Human Knowledge ), ambiguity (Wittgenstein 1953) and non-compositionality (Fodor 1981d) of sensory and imagistic representations, as well as their unsuitability to function as logical (Frege 1918/1997, Geach 1957) or mathematical (Frege 1884/1953) concepts, and the symmetry of resemblance (Goodman 1976), convinced philosophers that no theory of mind can get by with only nonconceptual representations construed in this way. (For more discussion, see the entry on nonconceputal mental content .)
There has also been dissent from the traditional claim that conceptual representations (thoughts, beliefs) lack phenomenology. Chalmers (1996), Flanagan (1992), Goldman (1993), Horgan and Tienson (2002), Jackendoff (1987), Levine (1993, 1995, 2001), McGinn (1991a), Pitt (2004, 2009, 2011, 2013), Searle (1992), Siewert (1998, 2011) and Strawson (1994, 2010), claim that purely conceptual (conscious) representational states themselves have a proprietary kind of phenomenology. This view – bread and butter, it should be said, among historical and contemporary Phenomenologists – has been gaining momentum of late among analytic philosophers of mind. (See, e.g., the essays in Bayne and Montague 2011 and Kriegel 2013, and Chudnoff 2015, Farkas 2008a, Kriegel 2011, Mendelovici 2018, Montague 2016.) If this claim is correct, the question of what role phenomenology plays in the determination of representational content re-arises for conceptual representation; and the eliminativist ambitions of Sellars, Brandom, Rey, et al. would meet a new obstacle. It would also raise prima facie problems for reductive representationalism, as well as for reductive naturalistic theories of intentional content, and externalism in general.
The view that there is a proprietary phenomenology of conscious thought – a cognitive ( conceptual , propositional ) phenomenology – claims that there is something it’s like to occurrently, consciously think a thought (entertain a propositional content), which is as different from other kinds of phenomenology (visual, auditory, etc.) as they are from each other. Opinions diverge, however, with respect to the role such phenomenology plays in determining the contents of conceptual/propositional representations. Some (e.g., Siewert) claim that it plays no such role. Others (e.g., Horgan and Tienson, Strawson) hold that it determines only “narrow” contents, further, “broad” contents being determined by extrinsic relations to represented objects and properties. Still others (e.g., Farkas 2008b, Pitt) argue that it is the only kind of conceptual content, insisting on a sharp distinction between content (sense) and reference. There is also disagreement about whether or not cognitive phenomenology determines but is distinct from conceptual/propositional content (e.g., Pitt 2004) or is identical to it (e.g., Pitt 2009).
Outstanding challenges for this thesis include unconscious thought (which seems to entail the existence of unconscious phenomenology, on this view), indexical concepts (whose content is standardly taken to be referentially individuated; see Pitt 2013 for an attempt to address this challenge), and nominal concepts (concepts expressed by utterances of names, likewise standardly referentially individuated).
See the entries on consciousness and intentionality and phenomenal intentionality for further discussion.
Among realists about non-conceptual representations, the central division is between representationalists (also called “representationists” and “intentionalists”) – e.g., Dretske (1995), Harman (1990), Leeds (1993), Lycan (1987, 1996), Rey (1991), Thau (2002), Tye (1995, 2000, 2009) – and phenomenalists (also called “phenomenists”) – e.g., Block (1996, 2003), Chalmers (1996, 2004), Evans (1982), Loar (2003a, 2003b), Peacocke (1983, 1989, 1992, 2001), Raffman (1995), Shoemaker (1990). Representationalists claim that the phenomenal content of a non-conceptual representation – i.e., its phenomenal character – is reducible to a kind of intentional content, naturalistically construed (à la Dretske). On this view, phenomenal contents are extrinsic properties represented by non-conceptual representations. In contrast, phenomenalists claim that the phenomenal content of a non-conceptual mental representation is identical to its intrinsic phenomenal properties.
The representationalist thesis is often formulated as the claim that phenomenal properties are representational or intentional. However, this formulation is ambiguous between a reductive and a non-reductive claim (though the term ‘representationalism’ is most often used for the reductive claim. See Chalmers 2004a). As a reductive claim, it means that the phenomenal content of an experience, the properties that characterize what it is like to have it (i.e., qualia ), are certain extrinsic properties it represents. For example, the blueness one might mention in describing one’s experience (perceptual representation) of a clear sky at noon is a property of the sky, not of one’s experience of it. Blueness is relevant to the characterization of one’s experience because one’s experience represents it, not because one’s experience instantiates it. An experience of the sky no more instantiates blueness than a thought that snow is cold instantiates coldness. On this view, the phenomenal content of sensory experience is explained as its representation of extrinsic properties. (See Byrne and Tye 2006, Dretske 1995, Harman 1990, Lycan 1987, 1996 and Tye 2014, 2015 for elaboration and defense of this “qualia externalism.” See Thompson 2008 and Pitt 2017 for objections to this account.) (See also the entry on representational theories of consciousness .)
As a non-reductive claim, it means that the phenomenal content of an experience is its intrinsic subjective phenomenal properties, which are themselves representational. One’s experience of the sky represents its color by instantiating phenomenal blueness. Among phenomenalists there is disagreement over whether non-conceptual representation requires complex structuring of phenomenal properties (Block and Peacocke, op. cit., Robinson 1994) or not (Loar 2003b). So-called “Ganzfeld” experiences, in which, for example, the visual field is completely taken up with a uniform experience of a single color, are a standard test case: Do Ganzfeld experiences represent anything? (It may be that doubts about the representationality of such experiences are simply a consequence of the fact that (outside of the laboratory) we never encounter things that would produce them. Supposing we routinely did (and especially if we had names for them), it seems unlikely such skepticism would arise.)
Most (reductive) representationalists are motivated by the conviction that one or another naturalistic explanation of intentionality (see the next section) is, in broad outline, correct, and by the desire to complete the naturalization of the mental by applying such theories to the problem of phenomenality. (Needless to say, many phenomenalists are just as eager to naturalize the phenomenal – though not in the same way.)
The main argument for representationalism appeals to the transparency of experience (cf. Tye 2000: 45–51). The properties that characterize what it’s like to have a sensory experience are presented in experience as properties of objects perceived: in attempting to attend to an experience, one seems to “see through it” to the objects and properties it is experiences of . [ 2 ] They are not presented as properties of the experience itself. If nonetheless they were properties of the experience, perception would be massively deceptive. But perception is not massively deceptive. In veridical perception, these properties are locally instantiated; in illusion and hallucination, they are not. On this view, introspection is indirect perception: one comes to know what phenomenal features one’s experience has by coming to know what objective features it represents. (Cf. also Dretske 1996, 1999.)
In order to account for the intuitive differences between conceptual and sensory representations, representationalists appeal to structural or functional properties. Dretske (1995), for example, distinguishes experiences and thoughts on the basis of the origin and nature of their functions: an experience of a property P is a state of a system whose evolved function is to indicate the presence of P in the environment; a thought representing the property P , on the other hand, is a state of a system whose assigned (learned) function is to calibrate the output of the experiential system. Rey (1991) takes both thoughts and experiences to be relations to sentences in the language of thought, and distinguishes them on the basis of (the functional roles of) such sentences’ constituent predicates. Lycan (1987, 1996) distinguishes them in terms of their functional-computational profiles. Tye (2000) distinguishes them in terms of their functional roles and the intrinsic structure of their vehicles: thoughts are representations in a language-like medium, whereas experiences are image-like representations consisting of “symbol-filled arrays.” (Cf. the account of mental images in Tye 1991.)
Phenomenalists tend to make use of the same sorts of features (function, intrinsic structure) in explaining some of the intuitive differences between thoughts and experiences; but they do not suppose that such features exhaust the differences between phenomenal and non-phenomenal representations. For the phenomenalist, it is the phenomenal properties of experiences – qualia themselves – that constitute the fundamental difference between experience and thought. Peacocke (1992), for example, develops the notion of a perceptual “scenario” (an assignment of phenomenal properties to coordinates of a three-dimensional egocentric space), whose content is “correct” (a semantic property) if in the corresponding “scene” (the portion of the external world represented by the scenario) properties are distributed as their phenomenal analogues are in the scenario.
Another sort of representation appealed to by some phenomenalists (e.g., Chalmers (2003), Block (2003)) is what Chalmers calls a “pure phenomenal concept.” A phenomenal concept in general is a concept whose denotation is a phenomenal property, and it may be discursive (‘the color of ripe bananas’), demonstrative (‘ this color’; Loar 1990/96)), or even more direct. On Chalmers’s view, a pure phenomenal concept is (something like) a conceptual/phenomenal hybrid consisting of a phenomenological “sample” (an image or an occurrent sensation) integrated with (or functioning as) a conceptual component (see also Balog 1999 and Papineau 2002). Phenomenal concepts are postulated to account for the apparent fact (among others) that, as McGinn (1991b) puts it, “you cannot form [introspective] concepts of conscious properties unless you yourself instantiate those properties.” One cannot have a phenomenal concept of a phenomenal property P , and, hence, phenomenal beliefs about P , without having experience of P , because P itself is (in some way) constitutive of the concept of P . (Cf. Jackson 1982, 1986 and Nagel 1974.) (The so-called “ phenomenal concept strategy” puts pure phenomenal concepts to use in defending the Knowledge Argument against physicalism. See Loar 1990/96, Chalmers 2004a. Alter and Walter 2007 is an excellent collection of essays on phenomenal concepts. See Conee 1994 and Pitt 2019 for skeptical responses to this strategy.)
Though imagery has played an important role in the history of philosophy of mind, the important contemporary literature on it is primarily psychological. (Tye 1991 and McGinn 2004 are notable recent exceptions.) In a series of psychological experiments done in the 1970s (summarized in Kosslyn 1980 and Shepard and Cooper 1982), subjects’ response time in tasks involving mental manipulation and examination of presented figures was found to vary in proportion to the spatial properties (size, orientation, etc.) of the figures presented. The question of how these experimental results are to be explained kindled a lively debate on the nature of imagery and imagination.
Kosslyn (1980) claims that the results suggest that the tasks were accomplished via the examination and manipulation of mental representations that themselves have spatial properties – i.e., pictorial representations, or images . Others, principally Pylyshyn (1979, 1981a, 1981b, 2003), argue that the empirical facts can be explained in terms exclusively of discursive , or propositional representations and cognitive processes defined over them. (Pylyshyn takes such representations to be sentences in a language of thought.)
The idea that pictorial representations are literally pictures in the head is not taken seriously by proponents of the pictorial view of imagery (see, e.g., Kosslyn and Pomerantz 1977). The claim is, rather, that mental images represent in a way that is relevantly like the way pictures represent. (Attention has been focused on visual imagery – hence the designation ‘pictorial’; though of course there may be imagery in other modalities – auditory, olfactory, etc. – as well. See O’Callaghan 2007 for discussion of auditory imagery.)
The distinction between pictorial and discursive representation can be characterized in terms of the distinction between analog and digital representation (Goodman 1976). This distinction has itself been variously understood (Fodor & Pylyshyn 1981, Goodman 1976, Haugeland 1981, Lewis 1971, McGinn 1989), though a widely accepted construal is that analog representation is continuous (i.e., in virtue of continuously variable properties of the representation), while digital representation is discrete (i.e., in virtue of properties a representation either has or doesn’t have) (Dretske 1981). (An analog/digital distinction may also be made with respect to cognitive processes . (Block 1983.)) On this understanding of the analog/digital distinction, imagistic representations, which represent in virtue of properties that may vary continuously (such as being more or less bright, loud, vivid, etc.), would be analog, while conceptual representations, whose properties do not vary continuously (a thought cannot be more or less about Elvis: either it is or it is not) would be digital.
It might be supposed that the pictorial/discursive distinction is best made in terms of the phenomenal/non-phenomenal distinction, but it is not obvious that this is the case. For one thing, there may be non-phenomenal properties of representations that vary continuously. Moreover, there are ways of understanding pictorial representation that presuppose neither phenomenality nor analogicity. According to Kosslyn (1980, 1982, 1983), a mental representation is “quasi-pictorial” when every part of the representation corresponds to a part of the object represented, and relative distances between parts of the object represented are preserved among the parts of the representation. But distances between parts of a representation can be defined functionally rather than spatially – for example, in terms of the number of discrete computational steps required to combine stored information about them. (Cf. Rey 1981.)
Tye (1991) proposes a view of images on which they are hybrid representations, consisting both of pictorial and discursive elements. On Tye’s account, images are “(labeled) interpreted symbol-filled arrays.” The symbols represent discursively, while their arrangement in arrays has representational significance (the location of each “cell” in the array represents a specific viewer-centered 2-D location on the surface of the imagined object).
See the entry on mental imagery for further discussion.
The contents of mental representations are typically taken to be abstract objects (properties, relations, propositions, sets, etc.). A pressing question, especially for the naturalist, is how mental representations come to have their contents. Here the issue is not how to naturalize content (abstract objects can’t be naturalized), but, rather, how to specify naturalistic content-determining relations between mental representations and the abstract objects they express. There are two basic types of contemporary naturalistic theories of content-determination and causal-informational and functional . [ 3 ]
Causal-informational theories (Dretske 1981, 1988, 1995) hold that the content of a mental representation is grounded in the information it carries about what does (Devitt 1996) or would (Fodor 1987, 1990a) cause it to occur. [ 4 ] There is, however, widespread agreement that causal-informational relations are not sufficient to determine the content of mental representations. Such relations are common, but representation is not. Tree trunks, smoke, thermostats and ringing telephones carry information about what they are causally related to, but they do not represent (in the relevant sense) what they carry information about. A mental representation can be caused by something it does not represent, and can represent something that has not caused it, whereas nothing can be caused by something that doesn’t cause it.
The main attempts to specify what makes a causal-informational state a mental representation are Asymmetric Dependency Theories (e.g., Fodor 1987, 1990a, 1994) and Teleological Theories (Dretske 1988, 1995, Fodor 1990b, Millikan 1984, Neander 2017, Papineau 1987). The Asymmetric Dependency Theory distinguishes merely informational relations from representational relations on the basis of their higher-order relations to each other: informational relations depend upon representational relations, but not vice versa. For example, if tokens of a mental state type are reliably caused by horses, cows-on-dark-nights, zebras-in-the-mist and Great Danes, then they carry information about horses, etc. If, however, such tokens are caused by cows-on-dark-nights, etc. because they were caused by horses, but not vice versa, then they represent horses (or the property horse ).
According to Teleological Theories, representational relations are those a representation-producing mechanism has the selected (by evolution or learning) function of establishing. For example, zebra-caused horse-representations do not mean zebra , because the mechanism by which such tokens are produced has the selected function of indicating horses, not zebras. The horse-representation-producing mechanism that responds to zebras is malfunctioning .
See the entries on teleological theories of mental content and causal theories of mental content .
Functional theories (Block 1986, Harman 1973), hold that the content of a mental representation is determined, at least in part, by its (causal, computational, inferential) relations to other mental representations. They differ on whether relata should include all other mental representations or only some of them, and on whether to include external states of affairs. The view that the content of a mental representation is determined by its inferential/computational relations with all other representations is holism ; the view it is determined by relations to only some other mental states is localism (or molecularism ). (The non-functional view that the content of a mental state depends on none of its relations to other mental states is atomism .) Functional theories that recognize no content-determining external relata have been called solipsistic (Harman 1987). Some theorists posit distinct roles for internal and external connections, the former determining semantic properties analogous to sense, the latter determining semantic properties analogous to reference (McGinn 1982, Sterelny 1989).
(Reductive) representationalists (Dretske, Lycan, Tye) usually take one or another of these theories to provide an explanation of the (non-conceptual) content of experiential states. They thus tend to be externalists (see the next section) about phenomenological as well as conceptual content. Phenomenalists and non-reductive representationalists (Block, Chalmers, Loar, Peacocke, Siewert), on the other hand, take it that the representational content of such states is (at least in part) determined by their intrinsic phenomenal properties. Further, those who advocate a phenomenally-based approach to conceptual content (Horgan and Tienson, Kriegel, Loar, Pitt, Searle, Siewert) also seem to be committed to internalist individuation of the content (if not the reference) of such states.
Persistent indeterminacy problems with causal-informational-teleological theories of content determination have motivated a growing number of (analytic) philosophers to seek a different approach, grounded not in external relations of representational states but in their intrinsic phenomenal properties. This approach has come to be known as the “Phenomenal Intentionality Research Program” (Kriegel 2013), or, simply “Phenomenal Intentionality.” These philosophers (including Bourget, Kriegel, Loar, Mendelovici, Montague, Pitt, Searle, Smithies (2012, 2013a and b, 2019), Strawson and Siewert), argue that causal-informational-teleological relations cannot yield the fine-grained, determinate content conceptual and perceptual representations possess, and that such content can only be delivered by phenomenal character. The cognitive phenomenology thesis (discussed above) is an important component of this overall approach.
Generally, those who, like informational theorists, think relations to one’s (natural or social) environment are (at least partially) determinative of the content of mental representations are externalists , or anti-individualists (e.g., Burge 1979, 1986b, 2010, McGinn 1977), whereas those who, like some proponents of functional theories, think representational content is determined by an individual’s intrinsic properties alone, are internalists (or individualists ; cf. Putnam 1975, Fodor 1981c). [ 5 ]
This issue is widely taken to be of central importance, since psychological explanation, whether commonsense or scientific, is supposed to be both causal and content-based. (Beliefs and desires cause the behaviors they do because they have the contents they do. For example, the desire that one have a beer and the beliefs that there is beer in the refrigerator and that the refrigerator is in the kitchen may explain one’s getting up and going to the kitchen.) If, however, a mental representation’s having a particular content is due to factors extrinsic to it, it is unclear how its having that content could determine its causal powers, which, arguably, must be intrinsic (see Stich 1983, Fodor 1982, 1987, 1994). Some who accept the standard arguments for externalism have argued that internal factors determine a component of the content of a mental representation. They say that mental representations have both “narrow” content (determined by intrinsic factors) and “wide” or “broad” content (determined by narrow content plus extrinsic factors). (This distinction may be applied to the sub-personal representations of cognitive science as well as to those of commonsense psychology. See von Eckardt 1993: 189.)
Narrow content has been variously construed. Putnam (1975), Fodor (1982: 114; 1994: 39ff), and Block (1986: 627ff), for example, seem to understand it as something like de dicto content (i.e., Fregean sense , or perhaps character , à la Kaplan 1989). On this construal, narrow content is context-independent and directly expressible. Fodor (1987) and Block (1986), however, have also characterized narrow content as radically inexpressible . On this construal, narrow content is a kind of proto-content, or content-determinant, and can be specified only indirectly, via specifications of context/wide-content pairings. On both construals, narrow contents are characterized as functions from context to (wide) content. The narrow content of a representation is determined by properties intrinsic to it or its possessor, such as its syntactic structure or its intramental computational or inferential role.
Burge (1986b) has argued that causation-based worries about externalist individuation of psychological content, and the introduction of the narrow notion, are misguided. Fodor (1994, 1998) has more recently urged that a scientific psychology might not need narrow content in order to supply naturalistic (causal) explanations of human cognition and action, since the sorts of cases they were introduced to handle, viz., Twin-Earth cases and Frege cases, are either nomologically impossible or dismissible as exceptions to non-strict psychological laws.
On the most common versions of externalism, though intentional contents are externally determined, mental representations themselves, and the states they partly constitute, remain “in the head.” More radical versions are possible. One might maintain that since thoughts are individuated by their contents, and some thought contents are partially constituted by objects external to the mind, then some thoughts are partly constituted by objects external to the mind. On such a view, a singular thought – i.e., a thought about a particular object – literally contains the object it is about. It is “object-involving.” Such a thought (and the mind that thinks it) thus extend beyond the boundaries of the skull. (This appears to be the view articulated in McDowell 1986, on which there is “interpenetration” between the mind and the world.)
See the entries on externalism about mental content and narrow mental content .
Clark and Chalmers (1998) and Clark (2001, 2005, 2008) have argued that mental representations may exist entirely “outside the head.” On their view, which they call “active externalism,” cognitive processes (e.g., calculation) may be realized in external media (e.g., a calculator or pen and paper), and the “coupled system” of the individual mind and the external workspace ought to count as a cognitive system – a mind –in its own right. Symbolic representations on external media would thus count as mental representations.
Clark and Chalmers’s paper has inspired a burgeoning literature on extended, embodied and interactive cognition. (Menary 2010 is a recent collection of essays. See also the entry on embodied cognition .)
The leading contemporary version of the Representational Theory of Mind, the Computational Theory of Mind (CTM), claims that the brain is a kind of computer and that mental processes are computations. According to CTM, cognitive states are constituted by computational relations to mental representations of various kinds, and cognitive processes are sequences of such states.
CTM develops RTM by attempting to explain all psychological states and processes in terms of mental representation. In the course of constructing detailed empirical theories of human and other animal cognition, and developing models of cognitive processes implementable in artificial information processing systems, cognitive scientists have proposed a variety of types of mental representations. While some of these may be suited to be mental relata of commonsense psychological states, some – so-called “subpersonal” or “sub-doxastic” representations – are not. Though many philosophers believe that CTM can provide the best scientific explanations of cognition and behavior, there is disagreement over whether such explanations will vindicate the commonsense psychological explanations of prescientific RTM.
According to Stich’s (1983) Syntactic Theory of Mind, for example, computational theories of psychological states should concern themselves only with the formal properties of the objects those states are relations to. Commitment to the explanatory relevance of content , however, is for most cognitive scientists fundamental (Fodor 1981a, Pylyshyn 1984, Von Eckardt 1993). That mental processes are computations, that computations are rule-governed sequences of semantically evaluable objects , and that the rules apply to the symbols in virtue of their content, are central tenets of mainstream cognitive science.
Explanations in cognitive science appeal to a many different kinds of mental representation, including, for example, the “mental models” of Johnson-Laird 1983, the “retinal arrays,” “primal sketches” and “2½-D sketches” of Marr 1982, the “frames” of Minsky 1974, the “sub-symbolic” structures of Smolensky 1989, the “quasi-pictures” of Kosslyn 1980, and the “interpreted symbol-filled arrays” of Tye 1991 – in addition to representations that may be appropriate to the explanation of commonsense psychological states. Computational explanations have been offered of, among other mental phenomena, belief (Fodor 1975, 2008 Field 1978), visual perception (Marr 1982, Osherson, et al. 1990), rationality (Newell and Simon 1972, Fodor 1975, Johnson-Laird and Wason 1977), language learning and use (Chomsky 1965, Pinker 1989), and musical comprehension (Lerdahl and Jackendoff 1983).
A fundamental disagreement among proponents of CTM concerns the realization of personal-level representations (e.g., thoughts) and processes (e.g., inferences) in the brain. The central debate here is between proponents of Classical Architectures and proponents of Connectionist Architectures .
The classicists (e.g., Turing 1950, Fodor 1975, 2000, 2003, 2008, Fodor and Pylyshyn 1988, Marr 1982, Newell and Simon 1976) hold that mental representations are symbolic structures, which typically have semantically evaluable constituents, and that mental processes are rule-governed manipulations of them that are sensitive to their constituent structure. The connectionists (e.g., McCulloch & Pitts 1943, Rumelhart 1989, Rumelhart and McClelland 1986, Smolensky 1988) hold that mental representations are realized by patterns of activation in a network of simple processors (“nodes”) and that mental processes consist of the spreading activation of such patterns. The nodes themselves are, typically, not taken to be semantically evaluable; nor do the patterns have semantically evaluable constituents. (Though there are versions of Connectionism – “localist” versions – on which individual nodes are taken to have semantic properties (e.g., Ballard 1986.) It is arguable, however, that localist theories are neither definitive nor representative of the connectionist program (Smolensky 1988, 1991, Chalmers 1993).)
Classicists are motivated (in part) by properties thought seems to share with language. Fodor’s Language of Thought Hypothesis (LOTH) (Fodor 1975, 1987, 2008), according to which the system of mental symbols constituting the neural basis of thought is structured like a language, provides a well-worked-out version of the classical approach as applied to commonsense psychology. (Cf. also Marr 1982 for an application of classical approach in scientific psychology.) According to the LOTH, the potential infinity of complex representational mental states is generated from a finite stock of primitive representational states, in accordance with recursive formation rules. This combinatorial structure accounts for the properties of productivity and systematicity of the system of mental representations. As in the case of symbolic languages, including natural languages (though Fodor does not suppose either that the LOTH explains only linguistic capacities or that only verbal creatures have this sort of cognitive architecture), these properties of thought are explained by appeal to the content of the representational units and their combinability into contentful complexes. That is, the semantics of both language and thought is compositional : the content of a complex representation is determined by the contents of its constituents and their structural configuration. (See, e.g.,Fodor and Lepore 2002.)
Connectionists are motivated mainly by a consideration of the architecture of the brain, which apparently consists of layered networks of interconnected neurons. They argue that this sort of architecture is unsuited to carrying out classical serial computations. For one thing, processing in the brain is typically massively parallel. In addition, the elements whose manipulation drives computation in connectionist networks (principally, the connections between nodes) are neither semantically compositional nor semantically evaluable, as they are on the classical approach. This contrast with classical computationalism is often characterized by saying that representation is, with respect to computation, distributed as opposed to local : representation is local if it is computationally basic; and distributed if it is not. (Another way of putting this is to say that for classicists mental representations are computationally atomic , whereas for connectionists they are not.)
Moreover, connectionists argue that information processing as it occurs in connectionist networks more closely resembles some features of actual human cognitive functioning. For example, whereas on the classical view learning involves something like hypothesis formation and testing (Fodor 1981c), on the connectionist model it is a matter of evolving distribution of “weights” (strengths) on the connections between nodes, and typically does not involve the formulation of hypotheses regarding the identity conditions for the objects of knowledge. The connectionist network is “trained up” by repeated exposure to the objects it is to learn to distinguish; and, though networks typically require many more exposures to the objects than do humans, this seems to model at least one feature of this type of human learning quite well. (Cf. the sonar example in Churchland 1989.)
Further, degradation in the performance of such networks in response to damage is gradual, not sudden as in the case of a classical information processor, and hence more accurately models the loss of human cognitive function as it typically occurs in response to brain damage. It is also sometimes claimed that connectionist systems show the kind of flexibility in response to novel situations typical of human cognition – situations in which classical systems are relatively “brittle” or “fragile.”
Some philosophers have maintained that connectionism entails that there are no propositional attitudes. Ramsey, Stich and Garon (1990) have argued that if connectionist models of cognition are basically correct, then there are no discrete representational states as conceived in ordinary commonsense psychology and classical cognitive science. Others, however (e.g., Smolensky 1989), hold that certain types of higher-level patterns of activity in a neural network may be roughly identified with the representational states of commonsense psychology. Still others (e.g., Fodor & Pylyshyn 1988, Heil 1991, Horgan and Tienson 1996) argue that language-of-thought style representation is both necessary in general and realizable within connectionist architectures. (MacDonald & MacDonald 1995 collects the central contemporary papers in the classicist/connectionist debate, and provides useful introductory material as well. See also Von Eckardt 2005.)
Whereas Stich (1983) accepts that mental processes are computational, but denies that computations are sequences of mental representations, others accept the notion of mental representation, but deny that CTM provides the correct account of mental states and processes.
Van Gelder (1995) denies that psychological processes are computational. He argues that cognitive systems are dynamic , and that cognitive states are not relations to mental symbols, but quantifiable states of a complex system consisting of (in the case of human beings) a nervous system, a body and the environment in which they are embedded. Cognitive processes are not rule-governed sequences of discrete symbolic states, but continuous, evolving total states of dynamic systems determined by continuous, simultaneous and mutually determining states of the systems’ components. Representation in a dynamic system is essentially information-theoretic, though the bearers of information are not symbols, but state variables or parameters. (See also Port and Van Gelder 1995; Clark 1997a, 1997b, 2008.)
Horst (1996), on the other hand, argues that though computational models may be useful in scientific psychology, they are of no help in achieving a philosophical understanding of the intentionality of commonsense mental states. CTM attempts to reduce the intentionality of such states to the intentionality of the mental symbols they are relations to. But, Horst claims, the relevant notion of symbolic content is essentially bound up with the notions of convention and intention. So CTM involves itself in a vicious circularity: the very properties that are supposed to be reduced are (tacitly) appealed to in the reduction.
See the entries on the computational theory of mind and connectionism .
To say that a mental object has semantic properties is, paradigmatically, to say that it is about , or true or false of, an object or objects, or that it is true or false simpliciter . Suppose I think that democracy is dying. I am thinking about democracy, and if what I think of it (that it is dying) is true of it, then my thought is true. According to RTM such states are to be explained as relations between agents and mental representations. To think that democracy is dying is to token in some way a mental representation whose content is that democracy is dying. On this view, the semantic properties of mental states are the semantic properties of the representations they are relations to.
Linguistic acts seem to share such properties with mental states. Suppose I say that democracy is dying. I am talking about democracy, and if what I say of it (that it is dying) is true of it, then my utterance is true. Now, to say that democracy is dying is (in part) to utter a sentence that means that democracy is dying. Many philosophers have thought that the semantic properties of linguistic expressions are inherited from the intentional mental states they are conventionally used to express (Grice 1957, Fodor 1978, Schiffer1972/1988, Searle 1983). On this view, the semantic properties of linguistic expressions are the semantic properties of the representations that are the mental relata of the states they are conventionally used to express. Fodor has famously argued that these states themselves have a language-like structure. (See the entry on the language of thought hypothesis .)
(Others, however, e.g., Davidson (1975, 1982) have suggested that the kind of thought human beings are capable of is not possible without language, so that the dependency might be reversed, or somehow mutual (see also Sellars 1956). (But see Martin 1987 for a defense of the claim that thought is possible without language. See also Chisholm and Sellars 1958.) Schiffer (1987) subsequently despaired of the success of what he calls “Intention-Based Semantics.”)
It is also widely held that in addition to having such properties as reference, truth-conditions and truth – so-called extensional properties – expressions of natural languages also have intensional properties, in virtue of expressing properties or propositions – i.e., in virtue of having meanings or senses , where two expressions may have the same reference, truth-conditions or truth value, yet express different properties or propositions (Frege 1892/1997). If the semantic properties of natural-language expressions are inherited from the thoughts and concepts they express (or vice versa, or both), then an analogous distinction may be appropriate for mental representations.
- Almog, J., Perry, J. and Wettstein, H. (eds.), 1989, Themes from Kaplan , New York: Oxford University Press.
- Alter, T. and Walter, S. (eds.), 2007, Phenomenal Concepts and Phenomenal Knowledge: New Essays on Consciousness and Physicalism , Oxford: Oxford University Press.
- Aristotle, 1984, De Anima , in The Complete Works of Aristotle: The Revised Oxford Translation , Oxford: Oxford University Press.
- Baker, L. R., 1995, Explaining Attitudes: A Practical Approach to the Mind , Cambridge: Cambridge University Press.
- Ballard, D.H., 1986, “Cortical Connections and Parallel Processing: Structure and Function,” The Behavioral and Brain Sciences , 9: 67–120.
- Bayne, T. and Montague, M. (eds.), 2011, Cognitive Phenomenology , Oxford: Oxford University Press.
- Beaney, M. (ed.), 1997, The Frege Reader , Oxford: Blackwell Publishers.
- Berkeley, G., 1975, Principles of Human Knowledge , in M.R. Ayers (ed.), Berkeley: Philosophical Writings , London: Dent.
- Block, N., 1983, “Mental Pictures and Cognitive Science,” Philosophical Review , 93: 499–542.
- –––, 1986, “Advertisement for a Semantics for Psychology,” in P.A. French, T.E. Uehling and H.K. Wettstein (eds.), Midwest Studies in Philosophy (Volume X), Minneapolis: University of Minnesota Press: 615–678.
- –––, 1996, “Mental Paint and Mental Latex,” in E. Villanueva (ed.), Philosophical Issues , 7 (Perception): 19–49.
- –––, 2003, “Mental Paint,” in M. Hahn and B. Ramberg (eds.), Reflections and Replies: Essays on the Philosophy of Tyler Burge , Cambridge, Mass.: The MIT Press.
- Block, N. (ed.), 1981, Readings in Philosophy of Psychology (Volume 2), Cambridge, Mass.: Harvard University Press.
- ––– (ed.), 1982, Imagery , Cambridge, Mass.: The MIT Press.
- Boghossian, P. A., 1995, “Content,” in J. Kim and E. Sosa (eds.), A Companion to Metaphysics , Oxford: Blackwell: 94–96.
- Brandom, R., 2002, “Non-inferential Knowledge, Perceptual Experience, and Secondary Qualities: Placing McDowell’s Empiricism,” in N.H. Smith (ed.), Reading McDowell: On Mind and World , London: Routledge.
- Burge, T., 1979, “Individualism and the Mental,” in P.A. French, T.E. Uehling and H.K.Wettstein (eds.), Midwest Studies in Philosophy (Volume IV), Minneapolis: University of Minnesota Press: 73–121; reprinted, with Postscript, in Burge 2007.
- –––, 1986a, “Individualism and Psychology,” Philosophical Review , 95: 3–45.
- –––, 1986b, “Intellectual Norms and Foundations of Mind,” The Journal of Philosophy , 83: 697–720.
- –––, 2007, Foundations of Mind: Philosophical Essays (Volume 2), Oxford: Oxford University Press.
- –––, 2010, Origins of Objectivity , Oxford: Oxford University Press.
- Byrne, A and Tye, M., 2006, “Qualia Ain’t in the Head,” Noûs , 40: 241–255.
- Chalmers, D., 1993, “Connectionism and Compositionality: Why Fodor and Pylyshyn Were Wrong,” Philosophical Psychology , 6: 305–319.
- –––, 1996, The Conscious Mind , New York: Oxford University Press.
- –––, 2003, “The Content and Epistemology of Phenomenal Belief,” in Q. Smith & A. Jokic (eds.), Consciousness: New Philosophical Perspectives , Oxford: Oxford University Press: 220–272.
- –––, 2004a, “The Representational Character of Experience,” in B. Leiter (ed.), The Future for Philosophy , Oxford: Oxford University Press: 153–181.
- –––, 2004b, “Phenomenal Concepts and the Knowledge Argument,” in P. Ludlow, Y. Nagasawa and D. Stoljar (eds.), There’s Something About Mary: Essays on Phenomenal Consciousness and Frank Jackson’s Knowledge Argument , Cambridge, Mass.: The MIT Press: 269–298.
- Chisholm, R. and Sellars, W., 1958, “The Chisholm-Sellars Correspondence on Intentionality,” in H. Feigl, M. Scriven and G. Maxwell (eds.), Minnesota Studies in the Philosophy of Science (Volume II), Minneapolis: University of Minnesota Press: 529–539.
- Chomsky, N., 1965, Aspects of the Theory of Syntax , Cambridge, Mass.: The MIT Press.
- Churchland, P.M., 1981, “Eliminative Materialism and the Propositional Attitudes,” Journal of Philosophy , 78: 67–90.
- –––, 1989, “On the Nature of Theories: A Neurocomputational Perspective,” in W. Savage (ed.), Scientific Theories (Minnesota Studies in the Philosophy of Science: Volume 14), Minneapolis: University of Minnesota Press: 59–101.
- Clark, A., 1997a, “The Dynamical Challenge,” Cognitive Science , 21: 461–481.
- –––, 1997b, Being There: Putting Brain, Body and World Together Again , Cambridge, MA: The MIT Press.
- –––, 2001, “Reasons, Robots and the Extended Mind,” Mind and Language , 16: 121–145.
- –––, 2005, “Intrinsic Content, Active Memory, and the Extended Mind,” Analysis , 65: 1–11.
- –––, 2008, Supersizing the Mind , Oxford: Oxford University Press.
- Clark, A., and Chalmers, D., 1998, “The Extended Mind,” Analysis , 58: 7–19.
- Collins, A., 1987, The Nature of Mental Things , Notre Dame: Notre Dame University Press.
- Conee, E., 1994, “Phenomenal Knowledge,” Australasian Journal of Philosophy , 72: 136–150.
- Crane, T., 1995, The Mechanical Mind , London: Penguin Books Ltd.
- Davidson, D., 1973, “Radical Interpretation,” Dialectica 27: 313–328.
- –––, 1974, “Belief and the Basis of Meaning,” Synthese , 27: 309–323.
- –––, 1975, “Thought and Talk,” in S. Guttenplan (ed.), Mind and Language , Oxford: Clarendon Press: 7–23.
- –––, 1982, “Rational Animals,” Dialectica , 4: 317–327.
- Dennett, D., 1969, Content and Consciousness , London: Routledge & Kegan Paul.
- –––, 1981, “The Nature of Images and the Introspective Trap,” in Dennett 1969: 132–141; reprinted in Block 1981: 128–134.
- –––, 1987a, The Intentional Stance , Cambridge, Mass.: The MIT Press.
- –––, 1987b, “True Believers: The Intentional Strategy and Why it Works,” in Dennett 1987: 13–35.
- –––, 1987c, “Reflections: Real Patterns, Deeper Facts, and Empty Questions,” in Dennett 1987: 37–42.
- –––, 1988, “Quining Qualia,” in A.J. Marcel and E. Bisiach (eds.), Consciousness in Contemporary Science , Oxford: Clarendon Press: 42–77.
- –––, 1991, “Real Patterns,” The Journal of Philosophy , 87: 27–51.
- Devitt, M. 1996, Coming to Our Senses: A Naturalistic Program for Semantic Localism , Cambridge: Cambridge University Press.
- Dretske, F. 1969, Seeing and Knowing , Chicago: The University of Chicago Press.
- –––, 1981, Knowledge and the Flow of Information , Cambridge, Mass.: The MIT Press.
- –––, 1988, Explaining Behavior: Reasons in a World of Causes , Cambridge, Mass.: The MIT Press.
- –––, 1995, Naturalizing the Mind , Cambridge, Mass.: The MIT Press.
- –––, 1996, “Phenomenal Externalism, or If Meanings Ain’t in the Head, Where are Qualia?”, in E. Villanueva (ed.), Philosophical Issues , 7 (Perception): 143–158.
- –––, 1998, “Minds, Machines, and Money: What Really Explains Behavior,” in J. Bransen and S. Cuypers (eds.), Human Action, Deliberation and Causation (Philosophical Studies Series: 77), Dordrecht: Kluwer Academic Publishers. Reprinted in Dretske 2000.
- –––, 1999, “The Mind’s Awareness of Itself,” Philosophical Studies , 95: 103–124.
- –––, 2000, Perception, Knowledge and Belief , Cambridge: Cambridge University Press.
- Evans, G., 1982, The Varieties of Reference , Oxford: Oxford University Press.
- Farkas, K., 2008a, The Subject’s Point of View , Oxford: Oxford University Press.
- –––, 2008b, “Phenomenal Intentionality Without Compromise,” The Monist , 91: 273–293.
- Field, H., 1978, “Mental representation,” Erkenntnis , 13: 9–61.
- Flanagan, O., 1992, Consciousness Reconsidered , Cambridge, Mass.: The MIT Press.
- Fodor, J.A., 1975, The Language of Thought , Cambridge, Mass.: Harvard University Press.
- –––, 1978, “Propositional Attitudes,” The Monist 61: 501–523.
- –––, 1981a, Representations , Cambridge, Mass.: The MIT Press.
- –––, 1981b, “Introduction,” in Fodor 1981a: 1–31.
- –––, 1981c, “Methodological Solipsism Considered as a Research Strategy in Cognitive Psychology,” in Fodor 1981a: 225–253.
- –––, 1981d, “The Present Status of the Innateness Controversy,” in Fodor 1981a: 257–316.
- –––, 1982, “Cognitive Science and the Twin-Earth Problem,” Notre Dame Journal of Formal Logic , 23: 98–118.
- –––, 1987, Psychosemantics , Cambridge, Mass.: The MIT Press.
- –––, 1990a, A Theory of Content and Other Essays , Cambridge, Mass.: The MIT Press.
- –––, 1990b, “Psychosemantics or: Where Do Truth Conditions Come From?” in W.G. Lycan (ed.), Mind and Cognition: A Reader , Oxford: Blackwell Publishers: 312–337.
- –––, 1994, The Elm and the Expert , Cambridge, Mass.: The MIT Press.
- –––, 1998, Concepts: Where Cognitive Science Went Wrong , Oxford: Oxford University Press.
- –––, 2000, The Mind Doesn’t Work that Way: The Scope and Limits of Computational Psychology , Cambridge, Mass.: The MIT Press.
- –––, 2003, LOT 2: The Language of Thought Revisited , Oxford: Clarendon Press.
- –––, 2008, The Mind Doesn’t Work that Way: The Scope and Limits of Computational Psychology , Cambridge, Mass.: The MIT Press.
- Fodor, J.A. and Lepore, E., 2002, The Compositionality Papers , Oxford: Clarendon Press.
- Fodor, J.A. and Pylyshyn, Z., 1981, “How Direct is Visual Perception?: Some Reflections on Gibson’s ‘Ecological Approach’,” Cognition , 9: 207–246.
- –––, 1988, “Connectionism and Cognitive Architecture: A Critical Analysis,” Cognition , 28: 3–71.
- Frege, G., 1884, The Foundations of Arithmetic , trans. J.L. Austin, New York: Philosophical Library, 1954.
- –––, 1892, “On Sinn and Bedeutung ”, in Beany 1997: 151–171.
- –––, 1918, “Thought”, in Beany 1997: 325–345.
- Geach, P., 1957, Mental Acts: Their Content and Their Objects , London: Routledge & Kegan Paul.
- Gibson, J.J., 1966, The senses considered as perceptual systems , Boston: Houghton Mifflin.
- –––, 1979, The ecological approach to visual perception , Boston: Houghton Mifflin.
- Goldman, A., 1993, “The Psychology of Folk Psychology,” Behavioral and Brian Sciences , 16: 15–28.
- Goodman, N., 1976, Languages of Art , 2nd edition, Indianapolis: Hackett.
- Grice, H.P., 1957, “Meaning,” Philosophical Review , 66: 377–388; reprinted in Studies in the Way of Words , Cambridge, Mass.: Harvard University Press (1989): 213–223.
- Gunther, Y.H. (ed.), 2003, Essays on Nonconceptual Content , Cambridge, Mass.: The MIT Press.
- Harman, G., 1973, Thought , Princeton: Princeton University Press.
- –––, 1987, “(Non-Solipsistic) Conceptual Role Semantics,” in E. Lepore (ed.), New Directions in Semantics , London: Academic Press: 55–81.
- –––, 1990, “The Intrinsic Quality of Experience,” in J. Tomberlin (ed.), Action Theory and Philosophy of Mind (Philosophical Perspectives: Volume 4), Atascadero: Ridgeview Publishing Company: 31–52.
- Harnish, R., 2002, Minds, Brains, Computers , Malden, Mass.: Blackwell Publishers Inc.
- Haugeland, J., 1981, “Analog and analog,” Philosophical Topics , 12: 213–226.
- Heil, J., 1991, “Being Indiscrete,” in J. Greenwood (ed.), The Future of Folk Psychology , Cambridge: Cambridge University Press: 120–134.
- Horgan, T. and Tienson, J. (1996), Connectionism and the Philosophy of Psychology , Cambridge, Mass: The MIT Press.
- –––, 2002, “The Intentionality of Phenomenology and the Phenomenology of Intentionality,” in D.J. Chalmers (ed.), Philosophy of Mind , Oxford: Oxford University Press: 520–533 .
- Horst, S., 1996, Symbols, Computation, and Intentionality , Berkeley: University of California Press.
- Hume, D., 1739, A Treatise of Human Nature , L.A. Selby-Bigg (ed.), rev. P.H. Nidditch, Oxford: Oxford University Press, 1978.
- Jackendoff, R., 1987, Computation and Cognition , Cambridge, Mass.: The MIT Press.
- Jackson, F., 1982, “Epiphenomenal Qualia,” Philosophical Quarterly , 32: 127–136.
- –––, 1986, “What Mary Didn’t Know,” Journal of Philosophy , 83: 291–295.
- Johnson-Laird, P.N., 1983, Mental Models , Cambridge, Mass.: Harvard University Press.
- Johnson-Laird, P.N. and Wason, P.C., 1977, Thinking: Readings in Cognitive Science , Cambridge University Press.
- Kaplan, D., 1989, “Demonstratives,” in Almog, Perry and Wettstein 1989: 481–614.
- Kosslyn, S.M., 1980, Image and Mind , Cambridge, Mass.: Harvard University Press.
- –––, 1982, “The Medium and the Message in Mental Imagery,” in Block 1982: 207–246.
- –––, 1983, Ghosts in the Mind’s Machine , New York: W.W. Norton & Co.
- Kosslyn, S.M. and Pomerantz, J.R., 1977, “Imagery, Propositions, and the Form of Internal Representations,” Cognitive Psychology , 9: 52–76.
- Kriegel, U., 2011, The Sources of Intentionality , Oxford: Oxford University Press.
- Kriegel, U. (ed.), 2013, Phenomenal Intentionality: New Essays , Oxford: Oxford University Press.
- Leeds, S., 1993, “Qualia, Awareness, Sellars,” Noûs XXVII: 303–329.
- Lerdahl, F. and Jackendoff, R., 1983, A Generative Theory of Tonal Music , Cambridge, Mass.: The MIT Press.
- Levine, J., 1993, “On Leaving Out What It’s Like,” in M. Davies and G. Humphreys (eds.), Consciousness , Oxford: Blackwell Publishers: 121–136.
- –––, 1995, “On What It Is Like to Grasp a Concept,” in E. Villanueva (ed.), Content (Philosophical Issues: Volume 6), Atascadero: Ridgeview Publishing Company: 38–43.
- –––, 2001, Purple Haze , Oxford: Oxford University Press.
- Lewis, D., 1971, “Analog and Digital,” Noûs , 5: 321–328.
- –––, 1974, “Radical Interpretation,” Synthese , 27: 331–344.
- Loar, B., 1981, Mind and Meaning , Cambridge: Cambridge University Press.
- –––, 1996, “Phenomenal States” (Revised Version), in N. Block, O. Flanagan and G. Güzeldere (eds.), The Nature of Consciousness , Cambridge, Mass.: The MIT Press: 597–616.
- –––, 2003a, “Transparent Experience and the Availability of Qualia,” in Q. Smith and A. Jokic (eds.), Consciousness: New Philosophical Perspectives , Oxford: Clarendon Press: 77–96.
- –––, 2003b, “Phenomenal Intentionality as the Basis of Mental Content,” in M. Hahn and B. Ramberg (eds.), Reflections and Replies: Essays on the Philosophy of Tyler Burge , Cambridge, Mass.: The MIT Press.
- Locke, J., 1689, An Essay Concerning Human Understanding , P.H. Nidditch (ed.), Oxford: Oxford University Press, 1975.
- Lycan, W.G., 1987, Consciousness , Cambridge, Mass.: The MIT Press.
- –––, 1986, Consciousness and Experience , Cambridge, Mass.: The MIT Press.
- MacDonald, C. and MacDonald, G., 1995, Connectionism: Debates on Psychological Explanation , Oxford: Blackwell Publishers.
- Marr, D., 1982, Vision , New York: W.H. Freeman and Company.
- Martin, C.B., 1987, “Proto-Language,” Australasian Journal of Philosophy , 65: 277–289.
- McCulloch, W.S. and Pitts, W., 1943, “A Logical Calculus of the Ideas Immanent in Nervous Activity,” Bulletin of Mathematical Biophysics , 5: 115–33.
- McDowell, J., 1986, “Singular Thought and the Extent of Inner Space,” in P. Pettit and J. McDowell (eds.), Subject, Thought, and Context , Oxford: Clarendon Press: 137–168.
- –––, 1994, Mind and World , Cambridge, Mass.: Harvard University Press.
- McGinn, C., 1977, “Charity, Interpretation, and Belief,” Journal of Philosophy , 74: 521–535.
- –––, 1982, “The Structure of Content,” in A. Woodfield (ed.), Thought and Content , Oxford: Oxford University Press: 207–258.
- –––, 1989, Mental Content , Oxford: Blackwell Publishers.
- –––, 1991a, The Problem of Consciousness , Oxford: Blackwell Publishers.
- –––, 1991b, “Content and Consciousness,” in McGinn 1991: 23–43.
- –––, 1991c, “Can We Solve the Mind-Body Problem?” in McGinn 1991: 1–22.
- –––, 2004, Mindsight: Image, Dream, Meaning , Cambridge, Mass.: Harvard University Press.
- Mendelovici, A., 2018, The Phenomenal Basis of Intentionality , Oxford: Oxford University Press.
- Millikan, R., 1984, Language, Thought and other Biological Categories , Cambridge, Mass.: The MIT Press.
- Menary, R. (ed.), 2010, The Extended Mind , Cambridge, Mass.: The MIT Press.
- Minsky, M., 1974, “A Framework for Representing Knowledge,” MIT-AI Laboratory Memo 306 June; abridged version in J. Haugeland (ed.), Mind Design II , Cambridge, Mass.: The MIT Press, 1997.
- Montague, M., 2016, The Given: Experience and Its Content, Oxford: Oxford University Press.
- Nagel, T., 1974, “What Is It Like to Be a Bat?” Philosophical Review , 83: 435–450.
- Neander, K., 2017, A Mark of the Mental: in Defense of Informational Teleosemantics Cambridge, Mass.: The MIT Press.
- Newell, A. and Simon, H.A., 1972, Human Problem Solving , New York: Prentice-Hall.
- –––, 1976, “Computer Science as Empirical Inquiry: Symbols and Search,” Communications of the Association for Computing Machinery , 19: 113–126.
- O’Callaghan, C., 2007, Sounds , Oxford: Oxford University Press.
- Osherson, D.N., Kosslyn, S.M. and Hollerbach, J.M., 1990, Visual Cognition and Action: An Invitation to Cognitive Science (Volume 2), Cambridge, Mass.: The MIT Press.
- Papineau, D., 1987, Reality and Representation , Oxford: Blackwell Publishers.
- Peacocke, C., 1983, Sense and Content , Oxford: Clarendon Press.
- –––, 1989, “Perceptual Content,” in Almog, Perry and Wettstein 1989: 297–329.
- –––, 1992, “Scenarios, Concepts and Perception,” in T. Crane (ed.), The Contents of Experience , Cambridge: Cambridge University Press: 105–35.
- –––, 2001, “Does Perception Have a Nonconceptual Content?” Journal of Philosophy , 99: 239–264.
- Pinker, S., 1989, Learnability and Cognition , Cambridge, Mass.: The MIT Press.
- Pitt, D., 2004, “The Phenomenology of Cognition, Or, What Is it Like to Think That P?” Philosophy and Phenomenological Research , 69: 1–36.
- –––, 2009, “Intentional Psychologism” Philosophical Studies , 146: 117–138.
- –––, 2011, “Introspection, Phenomenality and the Availability of Intentional Content,” in Bayne and Montague 2011: 141–173.
- –––, 2013, “Indexical Thought,” in Kriegel (ed.) Phenomenal Intentionality , Oxford: Oxford University Press: 49–70.
- –––, 2017, “The Paraphenomenal Hypothesis,” Analysis , 77: 735–741.
- –––, 2019, “Acquaintance and Phenomenal Concepts,” in S. Coleman (ed.), Cambridge Classic Arguments Series: the Knowledge Argument : 87–101.
- Port, R., and Van Gelder, T., 1995, Mind as Motion: Explorations in the Dynamics of Cognition , Cambridge, Mass.: The MIT Press.
- Putnam, H., 1975, “The Meaning of ‘Meaning’,” in Philosophical Papers, Vol. 2 , Cambridge: Cambridge University Press: 215–71.
- Pylyshyn, Z., 1979, “The Rate of ‘Mental Rotation’ of Images: A Test of a Holistic Analogue Hypothesis,” Memory and Cognition , 7: 19–28.
- –––, 1981a, “Imagery and Artificial Intelligence,” in Block 1981: 170–194.
- –––, 1981b, “The Imagery Debate: Analog Media versus Tacit Knowledge,” Psychological Review , 88: 16–45.
- –––, 1984, Computation and Cognition , Cambridge, Mass.: The MIT Press.
- –––, 2003, Seeing and Visualizing: It’s Not What You Think , Cambridge, Mass.: The MIT Press.
- Raffman, D., 1995, “The Persistence of Phenomenology,” in T. Metzinger (ed.), Conscious Experience , Paderborn: Schönigh/Imprint Academic: 293–308.
- Ramsey, W., Stich, S. and Garon, J., 1990, “Connectionism, Eliminativism and the Future of Folk Psychology,” Philosophical Perspectives , 4: 499–533.
- Reid, T., 1764, An Inquiry into the Human Mind , D.R. Brooks (ed.), Edinburgh: Edinburgh University Press, 1997.
- Rey, G., 1981, “Introduction: What Are Mental Images?” in Block 1981: 117–127.
- –––, 1991, “Sensations in a Language of Thought,” in E. Villaneuva (ed.), Consciousness (Philosophical Issues: Volume 1), Atascadero: Ridgeview Publishing Company: 73–112.
- Robinson, H., 1994, Perception , New York: Routledge.
- Rumelhart, D.E., 1989, “The Architecture of the Mind: A Connectionist Approach,” in M.I. Posner (ed.), Foundations of Cognitive Science , Cambridge, Mass.: The MIT Press: 133–159.
- Rumelhart, D.E. and McClelland, J.L., 1986. Parallel Distributed Processing (Volume I), Cambridge, Mass.: The MIT Press.
- Schiffer, S., 1987, Remnants of Meaning , Cambridge, Mass.: The MIT Press.
- –––, 1972, “Introduction” in Meaning , Oxford: Clarendon Press, 1988, xi-xxix.
- Searle, J.R., 1980, “Minds, Brains, and Programs,” Behavioral and Brain Sciences , 3: 417–424.
- –––, 1983, Intentionality , Cambridge: Cambridge University Press.
- –––, 1984, Minds, Brains, and Science , Cambridge: Harvard University Press.
- –––, 1992, The Rediscovery of the Mind , Cambridge, Mass.: The MIT Press.
- Sellars, W., 1956, “Empiricism and the Philosophy of Mind,” in K. Gunderson (ed.), Minnesota Studies in the Philosophy of Science (Volume I), Minneapolis: University of Minnesota Press: 253–329.
- Shepard, R.N. and Cooper, L., 198), Mental Images and their Transformations , Cambridge, Mass.: The MIT Press.
- Shoemaker, S., 1990, “Qualities and Qualia: What’s in the Mind?” Philosophy and Phenomenological Research , 50: 109–31.
- Siegel, S., 2010, The Contents of Visual Experience, New York: Oxford University Press.
- Siewert, C., 1998, The Significance of Consciousness , Princeton: Princeton University Press.
- –––, 2011, “Phenomenal Thought,”, in Bayne and Montague 2011: 236–267.
- Smithies, D., 2013a, “The Significance of Cognitive Phenomenology,”, Philosophy Compass , 8: 731–743.
- –––, 2013b, “The Nature of Cognitive Phenomenology,” Philosophy Compass , 8: 744–754.
- –––, 2019, The Epistemic Role of Consciousness , New York: Oxford University Press.
- Smolensky, P., 1988, “On the Proper Treatment of Connectionism,” Behavioral and Brain Sciences , 11: 1–74.
- –––, 1989, “Connectionist Modeling: Neural Computation/Mental Connections,” in L. Nadel, L.A. Cooper, P. Culicover and R.M. Harnish (eds.), Neural Connections, Mental Computation , Cambridge, Mass.:The MIT Press: 49–67.
- –––, 1991, “Connectionism and the Language of Thought,” in B. Loewer and G. Rey (eds.), Meaning in Mind: Fodor and His Critics , Oxford: Basil Blackwell Ltd.: 201–227.
- Sterelny, K., 1989, “Fodor’s Nativism,” Philosophical Studies , 55: 119–141.
- Stich, S., 1983, From Folk Psychology to Cognitive Science , Cambridge, Mass.: The MIT Press.
- ––– (1996), Deconstructing the Mind , New York: Oxford University Press.
- Strawson, G., 1994, Mental Reality , Cambridge, Mass.: The MIT Press.
- ––– (2008), Real Materialism and Other Essays , Oxford: Oxford University Press.
- Thompson, B., 2008, “Representationalism and the Argument from Hallucination,” Pacific Philosophical Quarterly , 89: 384–412.
- Thau, M., 2002, Consciousness and Cognition , Oxford: Oxford University Press.
- Turing, A., 1950, “Computing Machinery and Intelligence,” Mind , 59: 433–460.
- Tye, M., 1991, The Imagery Debate , Cambridge, Mass.: The MIT Press.
- –––, 1995, Ten Problems of Consciousness , Cambridge, Mass.: The MIT Press.
- –––, 2000, Consciousness, Color, and Content , Cambridge, Mass.: The MIT Press.
- –––, 2009, Consciousness Revisited , Cambridge, Mass.: The MIT Press.
- –––, 2015a, “Yes, Phenomenal Character Really is Out There in the World,” Philosophy and Phenomenological Research , XCI: 483–488.
- –––, 2015b, “Transparency, Qualia Realism and Representationalism,” Philosophical Studies , 170: 39–57.
- Van Gelder, T., 1995, “What Might Cognition Be, if not Computation?”, Journal of Philosophy , XCI: 345–381.
- Von Eckardt, B., 1993, What Is Cognitive Science? , Cambridge, Mass.: The MIT Press.
- –––, 2005, “Connectionism and the Propositional Attitudes,” in C.E. Erneling and D.M. Johnson (eds.), The Mind as a Scientific Object: Between Brain and Culture , Oxford: Oxford University Press.
- Wittgenstein, L., 1953, Philosophical Investigations , trans. G.E.M. Anscombe, Oxford: Blackwell Publishers.
How to cite this entry . Preview the PDF version of this entry at the Friends of the SEP Society . Look up topics and thinkers related to this entry at the Internet Philosophy Ontology Project (InPhO). Enhanced bibliography for this entry at PhilPapers , with links to its database.
[Please contact the author with suggestions.]
- Consciousness and Content on PhilPapers (edited by David Bourget).
- Intentionality on PhilPapers (edited by Robert Rupert).
- Perception on PhilPapers (edited by Benj Hellie).
artificial intelligence: logic-based | cognition: embodied | cognitive science | concepts | connectionism | consciousness: and intentionality | consciousness: representational theories of | externalism about the mind | folk psychology: as mental simulation | information: semantic conceptions of | intentionality | intentionality: phenomenal | language of thought hypothesis | materialism: eliminative | mental content: causal theories of | mental content: narrow | mental content: teleological theories of | mental imagery | mental representation: in medieval philosophy | mind: computational theory of | neuroscience, philosophy of | perception: the contents of | perception: the problem of | qualia | reference
Acknowledgments
Thanks to Brad Armour-Garb, Mark Balaguer, Dave Chalmers, Jim Garson, John Heil, Jeff Poland, Bill Robinson, Galen Strawson, Adam Vinueza and (especially) Barbara Von Eckardt for comments on earlier versions of this entry.
Copyright © 2020 by David Pitt < dalanpitt @ gmail . com >
- Accessibility
Support SEP
Mirror sites.
View this site from another server:
- Info about mirror sites
The Stanford Encyclopedia of Philosophy is copyright © 2023 by The Metaphysics Research Lab , Department of Philosophy, Stanford University
Library of Congress Catalog Data: ISSN 1095-5054
Physical Review Physics Education Research
- Collections
- Editorial Team
- Open Access
Role of mental representations in problem solving: Students’ approaches to nondirected tasks
Bashirah ibrahim and n. sanjay rebello, phys. rev. st phys. educ. res. 9 , 020106 – published 10 july 2013.
- Citing Articles (13)
- INTRODUCTION
- THEORETICAL FRAMEWORK
- DISCUSSION AND CONCLUSION
- ACKNOWLEDGEMENTS
In this paper, we report on a project concerned with the role of cognition during problem solving. We specifically explore the categories of mental representations that students work with during problem solving of different representational task formats. The sample, consisting of 19 engineering students taking a calculus-based physics course, attempted tasks from the topics of kinematics and work. Profiles were designed to capture the overall actions and reasoning of individual students across the various tasks. Two main profiles emerged from each topic under consideration. They were related to the Johnson-Laird cognitive framework to infer about the kinds of mental constructs. The results indicate that, for both topics, students work primarily at the level of propositional mental representation. When handling the kinematics tasks, a few students construct mental images and none of them can be categorized as using a mental model. In contrast, in the context of work, none of the participants generated a mental image while a minority of the sample was classified as using a mental model. Moreover, a comparison across the two topics indicates the predominance of propositional mental representation.
- Received 17 October 2012
DOI: https://doi.org/10.1103/PhysRevSTPER.9.020106

This article is available under the terms of the Creative Commons Attribution 3.0 License . Further distribution of this work must maintain attribution to the author(s) and the published article’s title, journal citation, and DOI.
Published by the American Physical Society
Authors & Affiliations
- Department of Physics, Kansas State University, 116 Cardwell Hall, Manhattan, Kansas 66506-2601, USA
Article Text
Vol. 9, Iss. 2 — July - December 2013
Access Options
Article part of CHORUS
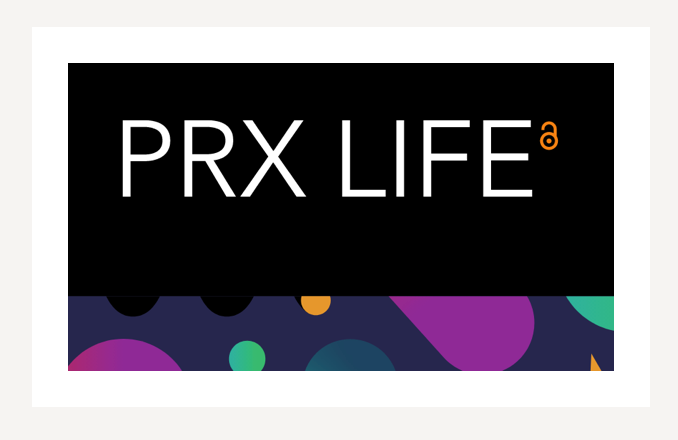
Authorization Required
Other options.
- Buy Article »
- Find an Institution with the Article »
Download & Share
Sign up to receive regular email alerts from Physical Review Physics Education Research
Reuse & Permissions
It is not necessary to obtain permission to reuse this article or its components as it is available under the terms of the Creative Commons Attribution 3.0 License . This license permits unrestricted use, distribution, and reproduction in any medium, provided attribution to the author(s) and the published article's title, journal citation, and DOI are maintained. Please note that some figures may have been included with permission from other third parties. It is your responsibility to obtain the proper permission from the rights holder directly for these figures.
- Forgot your username/password?
- Create an account
Article Lookup
Paste a citation or doi, enter a citation.
Piaget’s Theory and Stages of Cognitive Development
Saul Mcleod, PhD
Editor-in-Chief for Simply Psychology
BSc (Hons) Psychology, MRes, PhD, University of Manchester
Saul Mcleod, PhD., is a qualified psychology teacher with over 18 years of experience in further and higher education. He has been published in peer-reviewed journals, including the Journal of Clinical Psychology.
Learn about our Editorial Process
Olivia Guy-Evans, MSc
Associate Editor for Simply Psychology
BSc (Hons) Psychology, MSc Psychology of Education
Olivia Guy-Evans is a writer and associate editor for Simply Psychology. She has previously worked in healthcare and educational sectors.
On This Page:
Key Takeaways
- Jean Piaget is famous for his theories regarding changes in cognitive development that occur as we move from infancy to adulthood.
- Cognitive development results from the interplay between innate capabilities (nature) and environmental influences (nurture).
- Children progress through four distinct stages , each representing varying cognitive abilities and world comprehension: the sensorimotor stage (birth to 2 years), the preoperational stage (2 to 7 years), the concrete operational stage (7 to 11 years), and the formal operational stage (11 years and beyond).
- A child’s cognitive development is not just about acquiring knowledge, the child has to develop or construct a mental model of the world, which is referred to as a schema .
- Piaget emphasized the role of active exploration and interaction with the environment in shaping cognitive development, highlighting the importance of assimilation and accommodation in constructing mental schemas.
Stages of Development
Jean Piaget’s theory of cognitive development suggests that children move through four different stages of intellectual development which reflect the increasing sophistication of children’s thought
Each child goes through the stages in the same order (but not all at the same rate), and child development is determined by biological maturation and interaction with the environment.
At each stage of development, the child’s thinking is qualitatively different from the other stages, that is, each stage involves a different type of intelligence.
Although no stage can be missed out, there are individual differences in the rate at which children progress through stages, and some individuals may never attain the later stages.
Piaget did not claim that a particular stage was reached at a certain age – although descriptions of the stages often include an indication of the age at which the average child would reach each stage.
The Sensorimotor Stage
Ages: Birth to 2 Years
The first stage is the sensorimotor stage , during which the infant focuses on physical sensations and learning to coordinate its body.
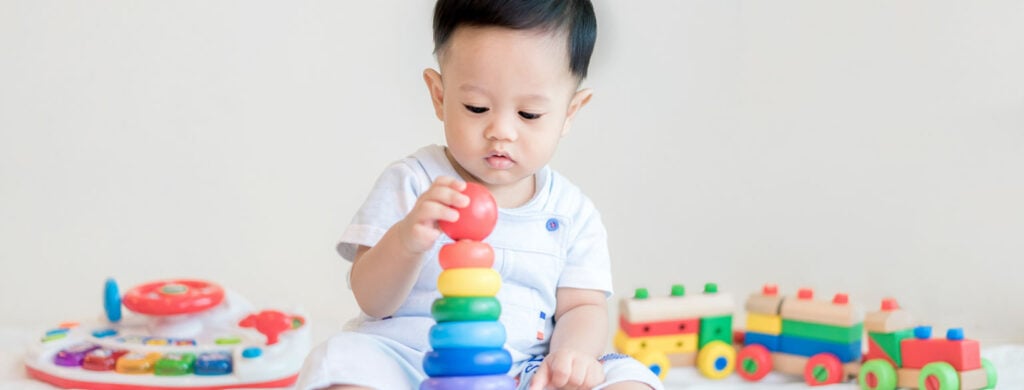
Major Characteristics and Developmental Changes:
- The infant learns about the world through their senses and through their actions (moving around and exploring their environment).
- During the sensorimotor stage, a range of cognitive abilities develop. These include: object permanence; self-recognition (the child realizes that other people are separate from them); deferred imitation; and representational play.
- They relate to the emergence of the general symbolic function, which is the capacity to represent the world mentally
- At about 8 months, the infant will understand the permanence of objects and that they will still exist even if they can’t see them and the infant will search for them when they disappear.
During the beginning of this stage, the infant lives in the present. It does not yet have a mental picture of the world stored in its memory therefore it does not have a sense of object permanence.
If it cannot see something, then it does not exist. This is why you can hide a toy from an infant, while it watches, but it will not search for the object once it has gone out of sight.
The main achievement during this stage is object permanence – knowing that an object still exists, even if it is hidden. It requires the ability to form a mental representation (i.e., a schema) of the object.
Towards the end of this stage the general symbolic function begins to appear where children show in their play that they can use one object to stand for another. Language starts to appear because they realise that words can be used to represent objects and feelings.
The child begins to be able to store information that it knows about the world, recall it, and label it.
Individual Differences
- Cultural Practices : In some cultures, babies are carried on their mothers’ backs throughout the day. This constant physical contact and varied stimuli can influence how a child perceives their environment and their sense of object permanence.
- Gender Norms : Toys assigned to babies can differ based on gender expectations. A boy might be given more cars or action figures, while a girl might receive dolls or kitchen sets. This can influence early interactions and sensory explorations.
Learn More: The Sensorimotor Stage of Cognitive Development
The Preoperational Stage
Ages: 2 – 7 Years
Piaget’s second stage of intellectual development is the preoperational stage . It takes place between 2 and 7 years. At the beginning of this stage, the child does not use operations, so the thinking is influenced by the way things appear rather than logical reasoning.
A child cannot conserve which means that the child does not understand that quantity remains the same even if the appearance changes.
Furthermore, the child is egocentric; he assumes that other people see the world as he does. This has been shown in the three mountains study.
As the preoperational stage develops, egocentrism declines, and children begin to enjoy the participation of another child in their games, and let’s pretend play becomes more important.
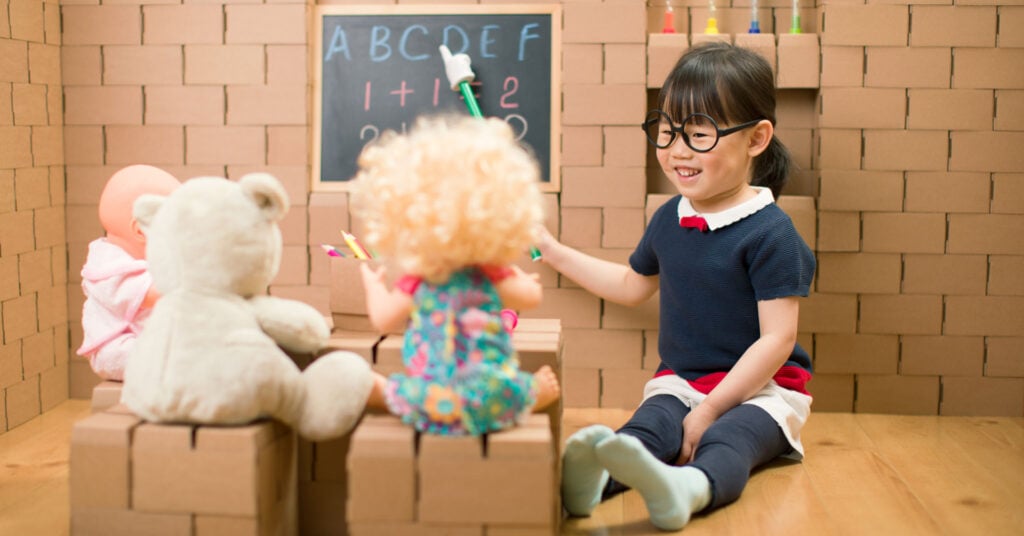
Toddlers often pretend to be people they are not (e.g. superheroes, policemen), and may play these roles with props that symbolize real-life objects. Children may also invent an imaginary playmate.
- Toddlers and young children acquire the ability to internally represent the world through language and mental imagery.
- During this stage, young children can think about things symbolically. This is the ability to make one thing, such as a word or an object, stand for something other than itself.
- A child’s thinking is dominated by how the world looks, not how the world is. It is not yet capable of logical (problem-solving) type of thought.
- Moreover, the child has difficulties with class inclusion; he can classify objects but cannot include objects in sub-sets, which involves classifying objects as belonging to two or more categories simultaneously.
- Infants at this stage also demonstrate animism. This is the tendency for the child to think that non-living objects (such as toys) have life and feelings like a person’s.
By 2 years, children have made some progress toward detaching their thoughts from the physical world. However, have not yet developed logical (or “operational”) thought characteristics of later stages.
Thinking is still intuitive (based on subjective judgments about situations) and egocentric (centered on the child’s own view of the world).
- Cultural Storytelling : Different cultures have unique stories, myths, and folklore. Children from diverse backgrounds might understand and interpret symbolic elements differently based on their cultural narratives.
- Race & Representation : A child’s racial identity can influence how they engage in pretend play. For instance, a lack of diverse representation in media and toys might lead children of color to recreate scenarios that don’t reflect their experiences or background.
Learn More: The Preoperational Stage of Cognitive Development
The Concrete Operational Stage
Ages: 7 – 11 Years
By the beginning of the concrete operational stage , the child can use operations (a set of logical rules) so they can conserve quantities, realize that people see the world in a different way (decentring), and demonstrate improvement in inclusion tasks. Children still have difficulties with abstract thinking.
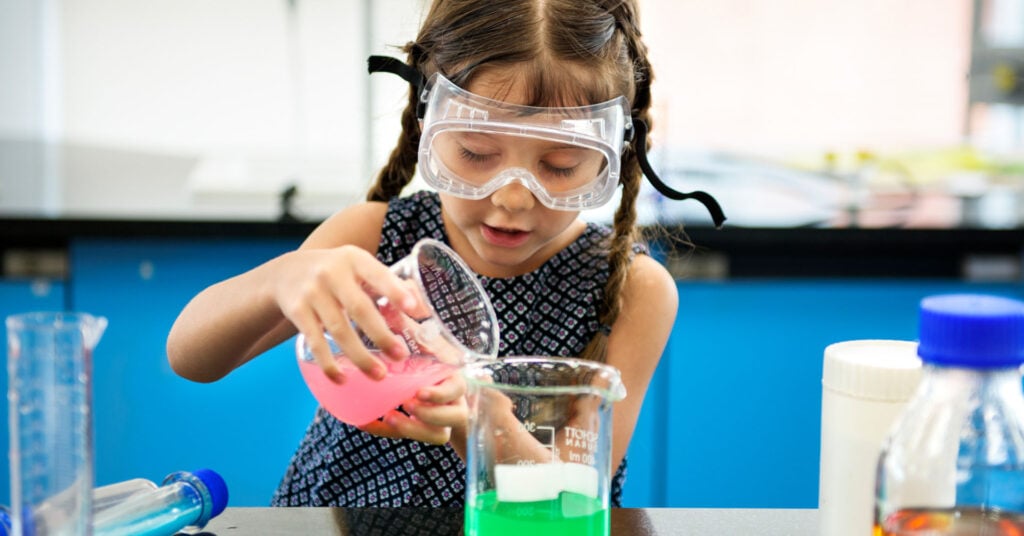
- During this stage, children begin to think logically about concrete events.
- Children begin to understand the concept of conservation; understanding that, although things may change in appearance, certain properties remain the same.
- During this stage, children can mentally reverse things (e.g., picture a ball of plasticine returning to its original shape).
- During this stage, children also become less egocentric and begin to think about how other people might think and feel.
The stage is called concrete because children can think logically much more successfully if they can manipulate real (concrete) materials or pictures of them.
Piaget considered the concrete stage a major turning point in the child’s cognitive development because it marks the beginning of logical or operational thought. This means the child can work things out internally in their head (rather than physically try things out in the real world).
Children can conserve number (age 6), mass (age 7), and weight (age 9). Conservation is the understanding that something stays the same in quantity even though its appearance changes.
But operational thought is only effective here if the child is asked to reason about materials that are physically present. Children at this stage will tend to make mistakes or be overwhelmed when asked to reason about abstract or hypothetical problems.
- Cultural Context in Conservation Tasks : In a society where resources are scarce, children might demonstrate conservation skills earlier due to the cultural emphasis on preserving and reusing materials.
- Gender & Learning : Stereotypes about gender abilities, like “boys are better at math,” can influence how children approach logical problems or classify objects based on perceived gender norms.
Learn More: The Concrete Operational Stage of Development
The Formal Operational Stage
Ages: 12 and Over
The formal operational period begins at about age 11. As adolescents enter this stage, they gain the ability to think in an abstract manner, the ability to combine and classify items in a more sophisticated way, and the capacity for higher-order reasoning.
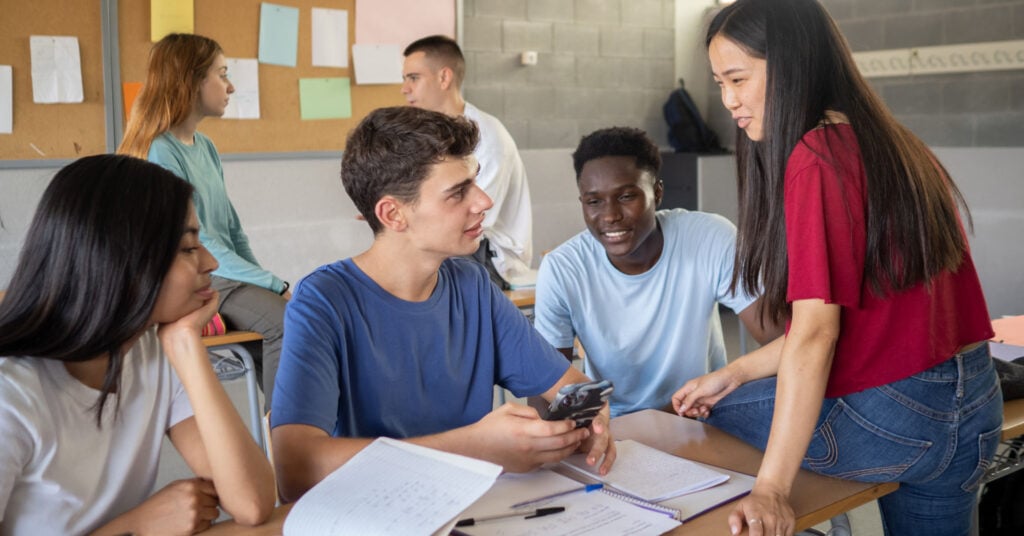
Adolescents can think systematically and reason about what might be as well as what is (not everyone achieves this stage). This allows them to understand politics, ethics, and science fiction, as well as to engage in scientific reasoning.
Adolescents can deal with abstract ideas: e.g. they can understand division and fractions without having to actually divide things up, and solve hypothetical (imaginary) problems.
- Concrete operations are carried out on things whereas formal operations are carried out on ideas. Formal operational thought is entirely freed from physical and perceptual constraints.
- During this stage, adolescents can deal with abstract ideas (e.g. no longer needing to think about slicing up cakes or sharing sweets to understand division and fractions).
- They can follow the form of an argument without having to think in terms of specific examples.
- Adolescents can deal with hypothetical problems with many possible solutions. E.g. if asked ‘What would happen if money were abolished in one hour’s time? they could speculate about many possible consequences.
From about 12 years children can follow the form of a logical argument without reference to its content. During this time, people develop the ability to think about abstract concepts, and logically test hypotheses.
This stage sees the emergence of scientific thinking, formulating abstract theories and hypotheses when faced with a problem.
- Culture & Abstract Thinking : Cultures emphasize different kinds of logical or abstract thinking. For example, in societies with a strong oral tradition, the ability to hold complex narratives might develop prominently.
- Gender & Ethics : Discussions about morality and ethics can be influenced by gender norms. For instance, in some cultures, girls might be encouraged to prioritize community harmony, while boys might be encouraged to prioritize individual rights.
Learn More: The Formal Operational Stage of Development
Piaget’s Theory
- Piaget’s theory places a strong emphasis on the active role that children play in their own cognitive development.
- According to Piaget, children are not passive recipients of information; instead, they actively explore and interact with their surroundings.
- This active engagement with the environment is crucial because it allows them to gradually build their understanding of the world.
1. How Piaget Developed the Theory
Piaget was employed at the Binet Institute in the 1920s, where his job was to develop French versions of questions on English intelligence tests. He became intrigued with the reasons children gave for their wrong answers to the questions that required logical thinking.
He believed that these incorrect answers revealed important differences between the thinking of adults and children.
Piaget branched out on his own with a new set of assumptions about children’s intelligence:
- Children’s intelligence differs from an adult’s in quality rather than in quantity. This means that children reason (think) differently from adults and see the world in different ways.
- Children actively build up their knowledge about the world . They are not passive creatures waiting for someone to fill their heads with knowledge.
- The best way to understand children’s reasoning is to see things from their point of view.
Piaget did not want to measure how well children could count, spell or solve problems as a way of grading their I.Q. What he was more interested in was the way in which fundamental concepts like the very idea of number , time, quantity, causality , justice , and so on emerged.
Piaget studied children from infancy to adolescence using naturalistic observation of his own three babies and sometimes controlled observation too. From these, he wrote diary descriptions charting their development.
He also used clinical interviews and observations of older children who were able to understand questions and hold conversations.
2. Piaget’s Theory Differs From Others In Several Ways:
Piaget’s (1936, 1950) theory of cognitive development explains how a child constructs a mental model of the world. He disagreed with the idea that intelligence was a fixed trait, and regarded cognitive development as a process that occurs due to biological maturation and interaction with the environment.
Children’s ability to understand, think about, and solve problems in the world develops in a stop-start, discontinuous manner (rather than gradual changes over time).
- It is concerned with children, rather than all learners.
- It focuses on development, rather than learning per se, so it does not address learning of information or specific behaviors.
- It proposes discrete stages of development, marked by qualitative differences, rather than a gradual increase in number and complexity of behaviors, concepts, ideas, etc.
The goal of the theory is to explain the mechanisms and processes by which the infant, and then the child, develops into an individual who can reason and think using hypotheses.
To Piaget, cognitive development was a progressive reorganization of mental processes as a result of biological maturation and environmental experience.
Children construct an understanding of the world around them, then experience discrepancies between what they already know and what they discover in their environment.
Piaget claimed that knowledge cannot simply emerge from sensory experience; some initial structure is necessary to make sense of the world.
According to Piaget, children are born with a very basic mental structure (genetically inherited and evolved) on which all subsequent learning and knowledge are based.
Schemas are the basic building blocks of such cognitive models, and enable us to form a mental representation of the world.
Piaget (1952, p. 7) defined a schema as: “a cohesive, repeatable action sequence possessing component actions that are tightly interconnected and governed by a core meaning.”
In more simple terms, Piaget called the schema the basic building block of intelligent behavior – a way of organizing knowledge. Indeed, it is useful to think of schemas as “units” of knowledge, each relating to one aspect of the world, including objects, actions, and abstract (i.e., theoretical) concepts.
Wadsworth (2004) suggests that schemata (the plural of schema) be thought of as “index cards” filed in the brain, each one telling an individual how to react to incoming stimuli or information.
When Piaget talked about the development of a person’s mental processes, he was referring to increases in the number and complexity of the schemata that a person had learned.
When a child’s existing schemas are capable of explaining what it can perceive around it, it is said to be in a state of equilibrium, i.e., a state of cognitive (i.e., mental) balance.
Operations are more sophisticated mental structures which allow us to combine schemas in a logical (reasonable) way.
As children grow they can carry out more complex operations and begin to imagine hypothetical (imaginary) situations.
Apart from the schemas we are born with schemas and operations are learned through interaction with other people and the environment.
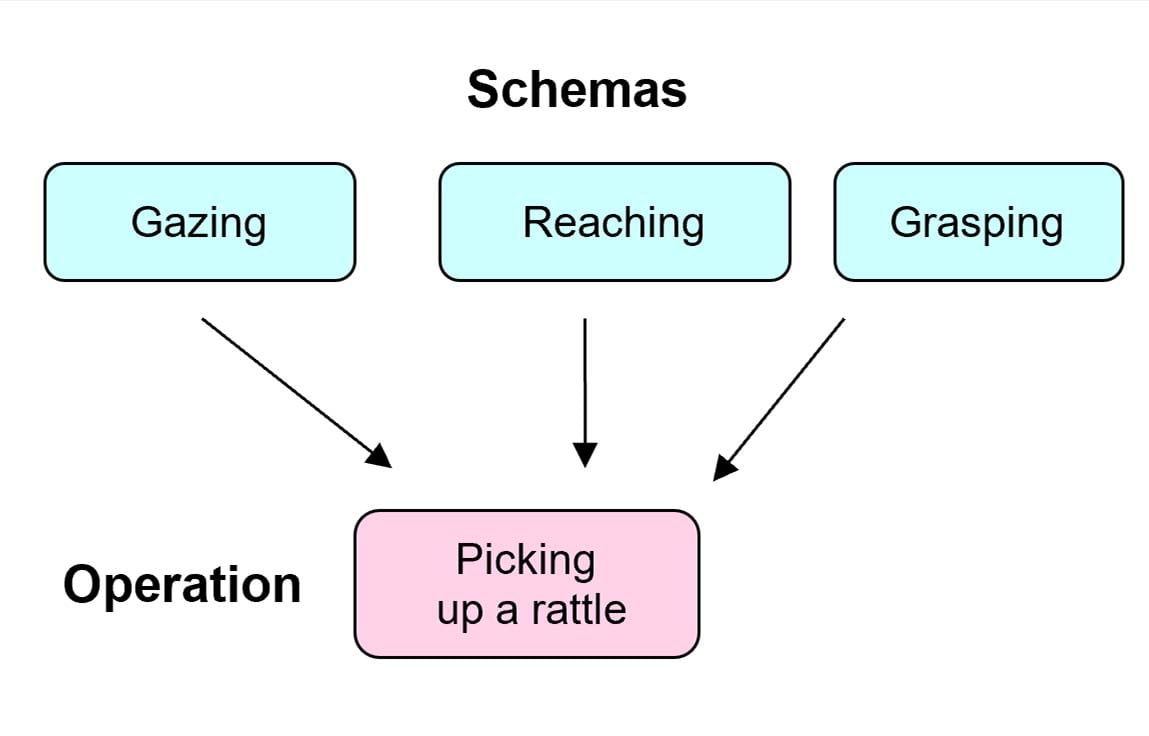
Piaget emphasized the importance of schemas in cognitive development and described how they were developed or acquired.
A schema can be defined as a set of linked mental representations of the world, which we use both to understand and to respond to situations. The assumption is that we store these mental representations and apply them when needed.
Examples of Schemas
A person might have a schema about buying a meal in a restaurant. The schema is a stored form of the pattern of behavior which includes looking at a menu, ordering food, eating it and paying the bill.
This is an example of a schema called a “script.” Whenever they are in a restaurant, they retrieve this schema from memory and apply it to the situation.
The schemas Piaget described tend to be simpler than this – especially those used by infants. He described how – as a child gets older – his or her schemas become more numerous and elaborate.
Piaget believed that newborn babies have a small number of innate schemas – even before they have had many opportunities to experience the world. These neonatal schemas are the cognitive structures underlying innate reflexes. These reflexes are genetically programmed into us.
For example, babies have a sucking reflex, which is triggered by something touching the baby’s lips. A baby will suck a nipple, a comforter (dummy), or a person’s finger. Piaget, therefore, assumed that the baby has a “sucking schema.”
Similarly, the grasping reflex which is elicited when something touches the palm of a baby’s hand, or the rooting reflex, in which a baby will turn its head towards something which touches its cheek, are innate schemas. Shaking a rattle would be the combination of two schemas, grasping and shaking.
4. The Process of Adaptation
Piaget also believed that a child developed as a result of two different influences: maturation, and interaction with the environment. The child develops mental structures (schemata) which enables him to solve problems in the environment.
Adaptation is the process by which the child changes its mental models of the world to match more closely how the world actually is.
Adaptation is brought about by the processes of assimilation (solving new experiences using existing schemata) and accommodation (changing existing schemata in order to solve new experiences).
The importance of this viewpoint is that the child is seen as an active participant in its own development rather than a passive recipient of either biological influences (maturation) or environmental stimulation.
When our existing schemas can explain what we perceive around us, we are in a state of equilibration . However, when we meet a new situation that we cannot explain it creates disequilibrium, this is an unpleasant sensation which we try to escape, and this gives us the motivation to learn.
According to Piaget, reorganization to higher levels of thinking is not accomplished easily. The child must “rethink” his or her view of the world. An important step in the process is the experience of cognitive conflict.
In other words, the child becomes aware that he or she holds two contradictory views about a situation and they both cannot be true. This step is referred to as disequilibrium .
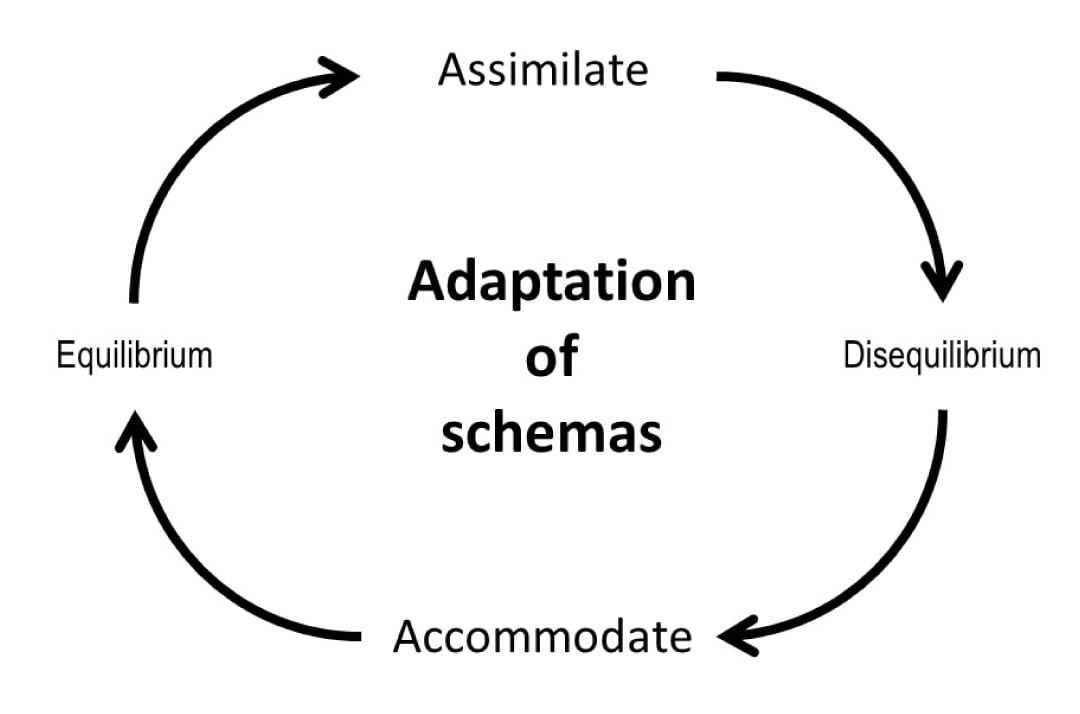
Jean Piaget (1952; see also Wadsworth, 2004) viewed intellectual growth as a process of adaptation (adjustment) to the world. This happens through assimilation, accommodation, and equilibration.
To get back to a state of equilibration, we need to modify our existing schemas to learn and adapt to the new situation.
This is done through the processes of accommodation and assimilation . This is how our schemas evolve and become more sophisticated. The processes of assimilation and accommodation are continuous and interactive.
5. Assimilation
Piaget defined assimilation as the cognitive process of fitting new information into existing cognitive schemas, perceptions, and understanding. Overall beliefs and understanding of the world do not change as a result of the new information.
Assimilation occurs when the new experience is not very different from previous experiences of a particular object or situation we assimilate the new situation by adding information to a previous schema.
This means that when you are faced with new information, you make sense of this information by referring to information you already have (information processed and learned previously) and trying to fit the new information into the information you already have.
- Imagine a young child who has only ever seen small, domesticated dogs. When the child sees a cat for the first time, they might refer to it as a “dog” because it has four legs, fur, and a tail – features that fit their existing schema of a dog.
- A person who has always believed that all birds can fly might label penguins as birds that can fly. This is because their existing schema or understanding of birds includes the ability to fly.
- A 2-year-old child sees a man who is bald on top of his head and has long frizzy hair on the sides. To his father’s horror, the toddler shouts “Clown, clown” (Siegler et al., 2003).
- If a baby learns to pick up a rattle he or she will then use the same schema (grasping) to pick up other objects.
6. Accommodation
Accommodation: when the new experience is very different from what we have encountered before we need to change our schemas in a very radical way or create a whole new schema.
Psychologist Jean Piaget defined accommodation as the cognitive process of revising existing cognitive schemas, perceptions, and understanding so that new information can be incorporated.
This happens when the existing schema (knowledge) does not work, and needs to be changed to deal with a new object or situation.
In order to make sense of some new information, you actually adjust information you already have (schemas you already have, etc.) to make room for this new information.
- A baby tries to use the same schema for grasping to pick up a very small object. It doesn’t work. The baby then changes the schema by now using the forefinger and thumb to pick up the object.
- A child may have a schema for birds (feathers, flying, etc.) and then they see a plane, which also flies, but would not fit into their bird schema.
- In the “clown” incident, the boy’s father explained to his son that the man was not a clown and that even though his hair was like a clown’s, he wasn’t wearing a funny costume and wasn’t doing silly things to make people laugh. With this new knowledge, the boy was able to change his schema of “clown” and make this idea fit better to a standard concept of “clown”.
- A person who grew up thinking all snakes are dangerous might move to an area where garden snakes are common and harmless. Over time, after observing and learning, they might accommodate their previous belief to understand that not all snakes are harmful.
7. Equilibration
Piaget believed that all human thought seeks order and is uncomfortable with contradictions and inconsistencies in knowledge structures. In other words, we seek “equilibrium” in our cognitive structures.
Equilibrium occurs when a child’s schemas can deal with most new information through assimilation. However, an unpleasant state of disequilibrium occurs when new information cannot be fitted into existing schemas (assimilation).
Piaget believed that cognitive development did not progress at a steady rate, but rather in leaps and bounds. Equilibration is the force which drives the learning process as we do not like to be frustrated and will seek to restore balance by mastering the new challenge (accommodation).
Once the new information is acquired the process of assimilation with the new schema will continue until the next time we need to make an adjustment to it.
Equilibration is a regulatory process that maintains a balance between assimilation and accommodation to facilitate cognitive growth. Think of it this way: We can’t merely assimilate all the time; if we did, we would never learn any new concepts or principles.
Everything new we encountered would just get put in the same few “slots” we already had. Neither can we accommodate all the time; if we did, everything we encountered would seem new; there would be no recurring regularities in our world. We’d be exhausted by the mental effort!
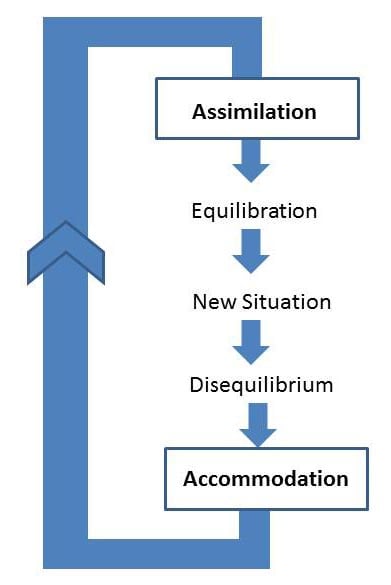
Applications to Education
Think of old black and white films that you’ve seen in which children sat in rows at desks, with ink wells, would learn by rote, all chanting in unison in response to questions set by an authoritarian old biddy like Matilda!
Children who were unable to keep up were seen as slacking and would be punished by variations on the theme of corporal punishment. Yes, it really did happen and in some parts of the world still does today.
Piaget is partly responsible for the change that occurred in the 1960s and for your relatively pleasurable and pain-free school days!
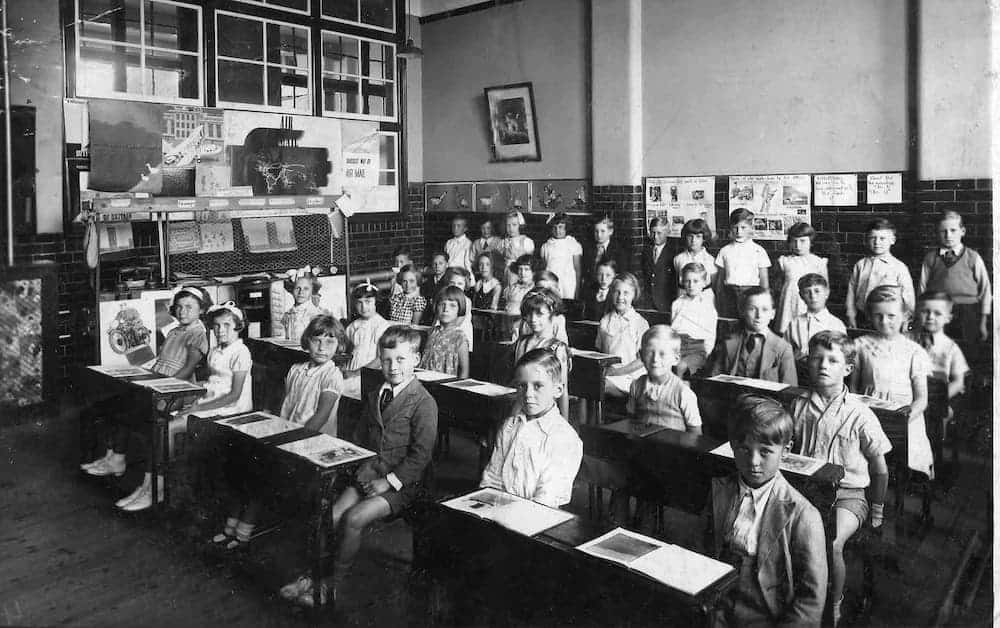
“Children should be able to do their own experimenting and their own research. Teachers, of course, can guide them by providing appropriate materials, but the essential thing is that in order for a child to understand something, he must construct it himself, he must re-invent it. Every time we teach a child something, we keep him from inventing it himself. On the other hand that which we allow him to discover by himself will remain with him visibly”. Piaget (1972, p. 27)
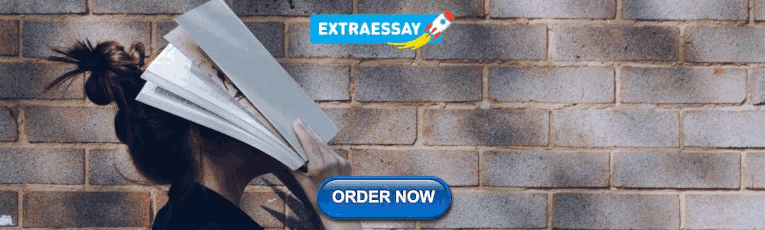
Plowden Report
Piaget (1952) did not explicitly relate his theory to education, although later researchers have explained how features of Piaget’s theory can be applied to teaching and learning.
Piaget has been extremely influential in developing educational policy and teaching practice. For example, a review of primary education by the UK government in 1966 was based strongly on Piaget’s theory. The result of this review led to the publication of the Plowden Report (1967).
In the 1960s the Plowden Committee investigated the deficiencies in education and decided to incorporate many of Piaget’s ideas into its final report published in 1967, even though Piaget’s work was not really designed for education.
The report makes three Piaget-associated recommendations:
- Children should be given individual attention and it should be realized that they need to be treated differently.
- Children should only be taught things that they are capable of learning
- Children mature at different rates and the teacher needs to be aware of the stage of development of each child so teaching can be tailored to their individual needs.
“The report’s recurring themes are individual learning, flexibility in the curriculum, the centrality of play in children’s learning, the use of the environment, learning by discovery and the importance of the evaluation of children’s progress – teachers should “not assume that only what is measurable is valuable.”
Discovery learning – the idea that children learn best through doing and actively exploring – was seen as central to the transformation of the primary school curriculum.
How to teach
Within the classroom learning should be student-centered and accomplished through active discovery learning. The role of the teacher is to facilitate learning, rather than direct tuition.
Because Piaget’s theory is based upon biological maturation and stages, the notion of “readiness” is important. Readiness concerns when certain information or concepts should be taught.
According to Piaget’s theory, children should not be taught certain concepts until they have reached the appropriate stage of cognitive development.
According to Piaget (1958), assimilation and accommodation require an active learner, not a passive one, because problem-solving skills cannot be taught, they must be discovered.
Therefore, teachers should encourage the following within the classroom:
- Educational programs should be designed to correspond to Piaget’s stages of development. Children in the concrete operational stage should be given concrete means to learn new concepts e.g. tokens for counting.
- Devising situations that present useful problems, and create disequilibrium in the child.
- Focus on the process of learning, rather than the end product of it. Instead of checking if children have the right answer, the teacher should focus on the student’s understanding and the processes they used to get to the answer.
- Child-centered approach. Learning must be active (discovery learning). Children should be encouraged to discover for themselves and to interact with the material instead of being given ready-made knowledge.
- Accepting that children develop at different rates so arrange activities for individual children or small groups rather than assume that all the children can cope with a particular activity.
- Using active methods that require rediscovering or reconstructing “truths.”
- Using collaborative, as well as individual activities (so children can learn from each other).
- Evaluate the level of the child’s development so suitable tasks can be set.
- Adapt lessons to suit the needs of the individual child (i.e. differentiated teaching).
- Be aware of the child’s stage of development (testing).
- Teach only when the child is ready. i.e. has the child reached the appropriate stage.
- Providing support for the “spontaneous research” of the child.
- Using collaborative, as well as individual activities.
- Educators may use Piaget’s stages to design age-appropriate assessment tools and strategies.
Classroom Activities
Sensorimotor stage (0-2 years):.
Although most kids in this age range are not in a traditional classroom setting, they can still benefit from games that stimulate their senses and motor skills.
- Object Permanence Games : Play peek-a-boo or hide toys under a blanket to help babies understand that objects still exist even when they can’t see them.
- Sensory Play : Activities like water play, sand play, or playdough encourage exploration through touch.
- Imitation : Children at this age love to imitate adults. Use imitation as a way to teach new skills.
Preoperational Stage (2-7 years):
- Role Playing : Set up pretend play areas where children can act out different scenarios, such as a kitchen, hospital, or market.
- Use of Symbols : Encourage drawing, building, and using props to represent other things.
- Hands-on Activities : Children should interact physically with their environment, so provide plenty of opportunities for hands-on learning.
- Egocentrism Activities : Use exercises that highlight different perspectives. For instance, having two children sit across from each other with an object in between and asking them what the other sees.
Concrete Operational Stage (7-11 years):
- Classification Tasks : Provide objects or pictures to group, based on various characteristics.
- Hands-on Experiments : Introduce basic science experiments where they can observe cause and effect, like a simple volcano with baking soda and vinegar.
- Logical Games : Board games, puzzles, and logic problems help develop their thinking skills.
- Conservation Tasks : Use experiments to showcase that quantity doesn’t change with alterations in shape, such as the classic liquid conservation task using different shaped glasses.
Formal Operational Stage (11 years and older):
- Hypothesis Testing : Encourage students to make predictions and test them out.
- Abstract Thinking : Introduce topics that require abstract reasoning, such as algebra or ethical dilemmas.
- Problem Solving : Provide complex problems and have students work on solutions, integrating various subjects and concepts.
- Debate and Discussion : Encourage group discussions and debates on abstract topics, highlighting the importance of logic and evidence.
- Feedback and Questioning : Use open-ended questions to challenge students and promote higher-order thinking. For instance, rather than asking, “Is this the right answer?”, ask, “How did you arrive at this conclusion?”
While Piaget’s stages offer a foundational framework, they are not universally experienced in the same way by all children.
Social identities play a critical role in shaping cognitive development, necessitating a more nuanced and culturally responsive approach to understanding child development.
Piaget’s stages may manifest differently based on social identities like race, gender, and culture:
- Race & Teacher Interactions : A child’s race can influence teacher expectations and interactions. For example, racial biases can lead to children of color being perceived as less capable or more disruptive, influencing their cognitive challenges and supports.
- Racial and Cultural Stereotypes : These can affect a child’s self-perception and self-efficacy . For instance, stereotypes about which racial or cultural groups are “better” at certain subjects can influence a child’s self-confidence and, subsequently, their engagement in that subject.
- Gender & Peer Interactions : Children learn gender roles from their peers. Boys might be mocked for playing “girl games,” and girls might be excluded from certain activities, influencing their cognitive engagements.
- Language : Multilingual children might navigate the stages differently, especially if their home language differs from their school language. The way concepts are framed in different languages can influence cognitive processing. Cultural idioms and metaphors can shape a child’s understanding of concepts and their ability to use symbolic representation, especially in the pre-operational stage.
Curriculum Development
According to Piaget, children’s cognitive development is determined by a process of maturation which cannot be altered by tuition so education should be stage-specific.
For example, a child in the concrete operational stage should not be taught abstract concepts and should be given concrete aid such as tokens to count with.
According to Piaget children learn through the process of accommodation and assimilation so the role of the teacher should be to provide opportunities for these processes to occur such as new material and experiences that challenge the children’s existing schemas.
Furthermore, according to this theory, children should be encouraged to discover for themselves and to interact with the material instead of being given ready-made knowledge.
Curricula need to be developed that take into account the age and stage of thinking of the child. For example there is no point in teaching abstract concepts such as algebra or atomic structure to children in primary school.
Curricula also need to be sufficiently flexible to allow for variations in the ability of different students of the same age. In Britain, the National Curriculum and Key Stages broadly reflect the stages that Piaget laid down.
For example, egocentrism dominates a child’s thinking in the sensorimotor and preoperational stages. Piaget would therefore predict that using group activities would not be appropriate since children are not capable of understanding the views of others.
However, Smith et al. (1998), point out that some children develop earlier than Piaget predicted and that by using group work children can learn to appreciate the views of others in preparation for the concrete operational stage.
The national curriculum emphasizes the need to use concrete examples in the primary classroom.
Shayer (1997), reported that abstract thought was necessary for success in secondary school (and co-developed the CASE system of teaching science). Recently the National curriculum has been updated to encourage the teaching of some abstract concepts towards the end of primary education, in preparation for secondary courses. (DfEE, 1999).
Child-centered teaching is regarded by some as a child of the ‘liberal sixties.’ In the 1980s the Thatcher government introduced the National Curriculum in an attempt to move away from this and bring more central government control into the teaching of children.
So, although the British National Curriculum in some ways supports the work of Piaget, (in that it dictates the order of teaching), it can also be seen as prescriptive to the point where it counters Piaget’s child-oriented approach.
However, it does still allow for flexibility in teaching methods, allowing teachers to tailor lessons to the needs of their students.
Social Media (Digital Learning)
Jean Piaget could not have anticipated the expansive digital age we now live in.
Today, knowledge dissemination and creation are democratized by the Internet, with platforms like blogs, wikis, and social media allowing for vast collaboration and shared knowledge. This development has prompted a reimagining of the future of education.
Classrooms, traditionally seen as primary sites of learning, are being overshadowed by the rise of mobile technologies and platforms like MOOCs (Passey, 2013).
The millennial generation, defined as the first to grow up with cable TV, the internet, and cell phones, relies heavily on technology.
They view it as an integral part of their identity, with most using it extensively in their daily lives, from keeping in touch with loved ones to consuming news and entertainment (Nielsen, 2014).
Social media platforms offer a dynamic environment conducive to Piaget’s principles. These platforms allow for interactions that nurture knowledge evolution through cognitive processes like assimilation and accommodation.
They emphasize communal interaction and shared activity, fostering both cognitive and socio-cultural constructivism. This shared activity promotes understanding and exploration beyond individual perspectives, enhancing social-emotional learning (Gehlbach, 2010).
A standout advantage of social media in an educational context is its capacity to extend beyond traditional classroom confines. As the material indicates, these platforms can foster more inclusive learning, bridging diverse learner groups.
This inclusivity can equalize learning opportunities, potentially diminishing biases based on factors like race or socio-economic status, resonating with Kegan’s (1982) concept of “recruitability.”
However, there are challenges. While the potential of social media in learning is vast, its practical application necessitates intention and guidance. Cuban, Kirkpatrick, and Peck (2001) note that certain educators and students are hesitant about integrating social media into educational contexts.
This hesitancy can stem from technological complexities or potential distractions. Yet, when harnessed effectively, social media can provide a rich environment for collaborative learning and interpersonal development, fostering a deeper understanding of content.
In essence, the rise of social media aligns seamlessly with constructivist philosophies. Social media platforms act as tools for everyday cognition, merging daily social interactions with the academic world, and providing avenues for diverse, interactive, and engaging learning experiences.
Applications to Parenting
Parents can use Piaget’s stages to have realistic developmental expectations of their children’s behavior and cognitive capabilities.
For instance, understanding that a toddler is in the pre-operational stage can help parents be patient when the child is egocentric.
Play Activities
Recognizing the importance of play in cognitive development, many parents provide toys and games suited for their child’s developmental stage.
Parents can offer activities that are slightly beyond their child’s current abilities, leveraging Vygotsky’s concept of the “Zone of Proximal Development,” which complements Piaget’s ideas.
- Peek-a-boo : Helps with object permanence.
- Texture Touch : Provide different textured materials (soft, rough, bumpy, smooth) for babies to touch and feel.
- Sound Bottles : Fill small bottles with different items like rice, beans, bells, and have children shake and listen to the different sounds.
- Memory Games : Using cards with pictures, place them face down, and ask students to find matching pairs.
- Role Playing and Pretend Play : Let children act out roles or stories that enhance symbolic thinking. Encourage symbolic play with dress-up clothes, playsets, or toy cash registers. Provide prompts or scenarios to extend their imagination.
- Story Sequencing : Give children cards with parts of a story and have them arranged in the correct order.
- Number Line Jumps : Create a number line on the floor with tape. Ask students to jump to the correct answer for math problems.
- Classification Games : Provide a mix of objects and ask students to classify them based on different criteria (e.g., color, size, shape).
- Logical Puzzle Games : Games that involve problem-solving using logic, such as simple Sudoku puzzles or logic grid puzzles.
- Debate and Discussion : Provide a topic and let students debate on pros and cons. This promotes abstract thinking and logical reasoning.
- Hypothesis Testing Games : Present a scenario and have students come up with hypotheses and ways to test them.
- Strategy Board Games : Games like chess, checkers, or Settlers of Catan can help in developing strategic and forward-thinking skills.
Critical Evaluation
- The influence of Piaget’s ideas on developmental psychology has been enormous. He changed how people viewed the child’s world and their methods of studying children.
He was an inspiration to many who came after and took up his ideas. Piaget’s ideas have generated a huge amount of research which has increased our understanding of cognitive development.
- Piaget (1936) was one of the first psychologists to make a systematic study of cognitive development. His contributions include a stage theory of child cognitive development, detailed observational studies of cognition in children, and a series of simple but ingenious tests to reveal different cognitive abilities.
- His ideas have been of practical use in understanding and communicating with children, particularly in the field of education (re: Discovery Learning). Piaget’s theory has been applied across education.
- According to Piaget’s theory, educational programs should be designed to correspond to the stages of development.
- Are the stages real? Vygotsky and Bruner would rather not talk about stages at all, preferring to see development as a continuous process. Others have queried the age ranges of the stages. Some studies have shown that progress to the formal operational stage is not guaranteed.
For example, Keating (1979) reported that 40-60% of college students fail at formal operation tasks, and Dasen (1994) states that only one-third of adults ever reach the formal operational stage.
The fact that the formal operational stage is not reached in all cultures and not all individuals within cultures suggests that it might not be biologically based.
- According to Piaget, the rate of cognitive development cannot be accelerated as it is based on biological processes however, direct tuition can speed up the development which suggests that it is not entirely based on biological factors.
- Because Piaget concentrated on the universal stages of cognitive development and biological maturation, he failed to consider the effect that the social setting and culture may have on cognitive development.
Cross-cultural studies show that the stages of development (except the formal operational stage) occur in the same order in all cultures suggesting that cognitive development is a product of a biological process of maturation.
However, the age at which the stages are reached varies between cultures and individuals which suggests that social and cultural factors and individual differences influence cognitive development.
Dasen (1994) cites studies he conducted in remote parts of the central Australian desert with 8-14-year-old Indigenous Australians. He gave them conservation of liquid tasks and spatial awareness tasks. He found that the ability to conserve came later in the Aboriginal children, between ages of 10 and 13 (as opposed to between 5 and 7, with Piaget’s Swiss sample).
However, he found that spatial awareness abilities developed earlier amongst the Aboriginal children than the Swiss children. Such a study demonstrates cognitive development is not purely dependent on maturation but on cultural factors too – spatial awareness is crucial for nomadic groups of people.
Vygotsky , a contemporary of Piaget, argued that social interaction is crucial for cognitive development. According to Vygotsky the child’s learning always occurs in a social context in cooperation with someone more skillful (MKO). This social interaction provides language opportunities and Vygotsky considered language the foundation of thought.
- Piaget’s methods (observation and clinical interviews) are more open to biased interpretation than other methods. Piaget made careful, detailed naturalistic observations of children, and from these, he wrote diary descriptions charting their development. He also used clinical interviews and observations of older children who were able to understand questions and hold conversations.
Because Piaget conducted the observations alone the data collected are based on his own subjective interpretation of events. It would have been more reliable if Piaget conducted the observations with another researcher and compared the results afterward to check if they are similar (i.e., have inter-rater reliability).
Although clinical interviews allow the researcher to explore data in more depth, the interpretation of the interviewer may be biased.
For example, children may not understand the question/s, they have short attention spans, they cannot express themselves very well, and may be trying to please the experimenter. Such methods meant that Piaget may have formed inaccurate conclusions.
- As several studies have shown Piaget underestimated the abilities of children because his tests were sometimes confusing or difficult to understand (e.g., Hughes , 1975).
Piaget failed to distinguish between competence (what a child is capable of doing) and performance (what a child can show when given a particular task). When tasks were altered, performance (and therefore competence) was affected. Therefore, Piaget might have underestimated children’s cognitive abilities.
For example, a child might have object permanence (competence) but still not be able to search for objects (performance). When Piaget hid objects from babies he found that it wasn’t till after nine months that they looked for it.
However, Piaget relied on manual search methods – whether the child was looking for the object or not.
Later, researchers such as Baillargeon and Devos (1991) reported that infants as young as four months looked longer at a moving carrot that didn’t do what it expected, suggesting they had some sense of permanence, otherwise they wouldn’t have had any expectation of what it should or shouldn’t do.
- The concept of schema is incompatible with the theories of Bruner (1966) and Vygotsky (1978). Behaviorism would also refute Piaget’s schema theory because is cannot be directly observed as it is an internal process. Therefore, they would claim it cannot be objectively measured.
- Piaget studied his own children and the children of his colleagues in Geneva to deduce general principles about the intellectual development of all children. His sample was very small and composed solely of European children from families of high socio-economic status. Researchers have, therefore, questioned the generalisability of his data.
- For Piaget, language is considered secondary to action, i.e., thought precedes language. The Russian psychologist Lev Vygotsky (1978) argues that the development of language and thought go together and that the origin of reasoning has more to do with our ability to communicate with others than with our interaction with the material world.
Piaget’s Theory vs Vygotsky
Piaget maintains that cognitive development stems largely from independent explorations in which children construct knowledge of their own.
Whereas Vygotsky argues that children learn through social interactions, building knowledge by learning from more knowledgeable others such as peers and adults. In other words, Vygotsky believed that culture affects cognitive development.
These factors lead to differences in the education style they recommend: Piaget would argue for the teacher to provide opportunities that challenge the children’s existing schemas and for children to be encouraged to discover for themselves.
Alternatively, Vygotsky would recommend that teachers assist the child to progress through the zone of proximal development by using scaffolding.
However, both theories view children as actively constructing their own knowledge of the world; they are not seen as just passively absorbing knowledge.
They also agree that cognitive development involves qualitative changes in thinking, not only a matter of learning more things.
What is cognitive development?
Cognitive development is how a person’s ability to think, learn, remember, problem-solve, and make decisions changes over time.
This includes the growth and maturation of the brain, as well as the acquisition and refinement of various mental skills and abilities.
Cognitive development is a major aspect of human development, and both genetic and environmental factors heavily influence it. Key domains of cognitive development include attention, memory, language skills, logical reasoning, and problem-solving.
Various theories, such as those proposed by Jean Piaget and Lev Vygotsky, provide different perspectives on how this complex process unfolds from infancy through adulthood.
What are the 4 stages of Piaget’s theory?
Piaget divided children’s cognitive development into four stages; each of the stages represents a new way of thinking and understanding the world.
He called them (1) sensorimotor intelligence , (2) preoperational thinking , (3) concrete operational thinking , and (4) formal operational thinking . Each stage is correlated with an age period of childhood, but only approximately.
According to Piaget, intellectual development takes place through stages that occur in a fixed order and which are universal (all children pass through these stages regardless of social or cultural background).
Development can only occur when the brain has matured to a point of “readiness”.
What are some of the weaknesses of Piaget’s theory?
Cross-cultural studies show that the stages of development (except the formal operational stage) occur in the same order in all cultures suggesting that cognitive development is a product of a biological maturation process.
However, the age at which the stages are reached varies between cultures and individuals, suggesting that social and cultural factors and individual differences influence cognitive development.
What are Piaget’s concepts of schemas?
Schemas are mental structures that contain all of the information relating to one aspect of the world around us.
According to Piaget, we are born with a few primitive schemas, such as sucking, which give us the means to interact with the world.
These are physical, but as the child develops, they become mental schemas. These schemas become more complex with experience.
Baillargeon, R., & DeVos, J. (1991). Object permanence in young infants: Further evidence . Child development , 1227-1246.
Bruner, J. S. (1966). Toward a theory of instruction. Cambridge, Mass.: Belkapp Press.
Cuban, L., Kirkpatrick, H., & Peck, C. (2001). High access and low use of technologies in high school classrooms: Explaining an apparent paradox. American Educational Research Journal , 38 (4), 813-834.
Dasen, P. (1994). Culture and cognitive development from a Piagetian perspective. In W .J. Lonner & R.S. Malpass (Eds.), Psychology and culture (pp. 145–149). Boston, MA: Allyn and Bacon.
Gehlbach, H. (2010). The social side of school: Why teachers need social psychology. Educational Psychology Review , 22 , 349-362.
Hughes, M. (1975). Egocentrism in preschool children . Unpublished doctoral dissertation. Edinburgh University.
Inhelder, B., & Piaget, J. (1958). The growth of logical thinking from childhood to adolescence . New York: Basic Books.
Keating, D. (1979). Adolescent thinking. In J. Adelson (Ed.), Handbook of adolescent psychology (pp. 211-246). New York: Wiley.
Kegan, R. (1982). The evolving self: Problem and process in human development . Harvard University Press.
Nielsen. 2014. “Millennials: Technology = Social Connection.” http://www.nielsen.com/content/corporate/us/en/insights/news/2014/millennials-technology-social-connecti on.html.
Passey, D. (2013). Inclusive technology enhanced learning: Overcoming cognitive, physical, emotional, and geographic challenges . Routledge.
Piaget, J. (1932). The moral judgment of the child . London: Routledge & Kegan Paul.
Piaget, J. (1936). Origins of intelligence in the child. London: Routledge & Kegan Paul.
Piaget, J. (1945). Play, dreams and imitation in childhood . London: Heinemann.
Piaget, J. (1957). Construction of reality in the child. London: Routledge & Kegan Paul.
Piaget, J., & Cook, M. T. (1952). The origins of intelligence in children . New York, NY: International University Press.
Piaget, J. (1981). Intelligence and affectivity: Their relationship during child development.(Trans & Ed TA Brown & CE Kaegi) . Annual Reviews.
Plowden, B. H. P. (1967). Children and their primary schools: A report (Research and Surveys). London, England: HM Stationery Office.
Siegler, R. S., DeLoache, J. S., & Eisenberg, N. (2003). How children develop . New York: Worth.
Vygotsky, L. S. (1978). Mind in society: The development of higher psychological processes . Cambridge, MA: Harvard University Press.
Wadsworth, B. J. (2004). Piaget’s theory of cognitive and affective development: Foundations of constructivism . New York: Longman.
Further Reading
- BBC Radio Broadcast about the Three Mountains Study
- Piagetian stages: A critical review
- Bronfenbrenner’s Ecological Systems Theory

- Search Menu
- Browse content in Arts and Humanities
- Browse content in Archaeology
- Anglo-Saxon and Medieval Archaeology
- Archaeological Methodology and Techniques
- Archaeology by Region
- Archaeology of Religion
- Archaeology of Trade and Exchange
- Biblical Archaeology
- Contemporary and Public Archaeology
- Environmental Archaeology
- Historical Archaeology
- History and Theory of Archaeology
- Industrial Archaeology
- Landscape Archaeology
- Mortuary Archaeology
- Prehistoric Archaeology
- Underwater Archaeology
- Urban Archaeology
- Zooarchaeology
- Browse content in Architecture
- Architectural Structure and Design
- History of Architecture
- Residential and Domestic Buildings
- Theory of Architecture
- Browse content in Art
- Art Subjects and Themes
- History of Art
- Industrial and Commercial Art
- Theory of Art
- Biographical Studies
- Byzantine Studies
- Browse content in Classical Studies
- Classical Literature
- Classical Reception
- Classical History
- Classical Philosophy
- Classical Mythology
- Classical Art and Architecture
- Classical Oratory and Rhetoric
- Greek and Roman Papyrology
- Greek and Roman Archaeology
- Greek and Roman Epigraphy
- Greek and Roman Law
- Late Antiquity
- Religion in the Ancient World
- Digital Humanities
- Browse content in History
- Colonialism and Imperialism
- Diplomatic History
- Environmental History
- Genealogy, Heraldry, Names, and Honours
- Genocide and Ethnic Cleansing
- Historical Geography
- History by Period
- History of Emotions
- History of Agriculture
- History of Education
- History of Gender and Sexuality
- Industrial History
- Intellectual History
- International History
- Labour History
- Legal and Constitutional History
- Local and Family History
- Maritime History
- Military History
- National Liberation and Post-Colonialism
- Oral History
- Political History
- Public History
- Regional and National History
- Revolutions and Rebellions
- Slavery and Abolition of Slavery
- Social and Cultural History
- Theory, Methods, and Historiography
- Urban History
- World History
- Browse content in Language Teaching and Learning
- Language Learning (Specific Skills)
- Language Teaching Theory and Methods
- Browse content in Linguistics
- Applied Linguistics
- Cognitive Linguistics
- Computational Linguistics
- Forensic Linguistics
- Grammar, Syntax and Morphology
- Historical and Diachronic Linguistics
- History of English
- Language Evolution
- Language Reference
- Language Variation
- Language Families
- Language Acquisition
- Lexicography
- Linguistic Anthropology
- Linguistic Theories
- Linguistic Typology
- Phonetics and Phonology
- Psycholinguistics
- Sociolinguistics
- Translation and Interpretation
- Writing Systems
- Browse content in Literature
- Bibliography
- Children's Literature Studies
- Literary Studies (Romanticism)
- Literary Studies (American)
- Literary Studies (Modernism)
- Literary Studies (Asian)
- Literary Studies (European)
- Literary Studies (Eco-criticism)
- Literary Studies - World
- Literary Studies (1500 to 1800)
- Literary Studies (19th Century)
- Literary Studies (20th Century onwards)
- Literary Studies (African American Literature)
- Literary Studies (British and Irish)
- Literary Studies (Early and Medieval)
- Literary Studies (Fiction, Novelists, and Prose Writers)
- Literary Studies (Gender Studies)
- Literary Studies (Graphic Novels)
- Literary Studies (History of the Book)
- Literary Studies (Plays and Playwrights)
- Literary Studies (Poetry and Poets)
- Literary Studies (Postcolonial Literature)
- Literary Studies (Queer Studies)
- Literary Studies (Science Fiction)
- Literary Studies (Travel Literature)
- Literary Studies (War Literature)
- Literary Studies (Women's Writing)
- Literary Theory and Cultural Studies
- Mythology and Folklore
- Shakespeare Studies and Criticism
- Browse content in Media Studies
- Browse content in Music
- Applied Music
- Dance and Music
- Ethics in Music
- Ethnomusicology
- Gender and Sexuality in Music
- Medicine and Music
- Music Cultures
- Music and Media
- Music and Culture
- Music and Religion
- Music Education and Pedagogy
- Music Theory and Analysis
- Musical Scores, Lyrics, and Libretti
- Musical Structures, Styles, and Techniques
- Musicology and Music History
- Performance Practice and Studies
- Race and Ethnicity in Music
- Sound Studies
- Browse content in Performing Arts
- Browse content in Philosophy
- Aesthetics and Philosophy of Art
- Epistemology
- Feminist Philosophy
- History of Western Philosophy
- Metaphysics
- Moral Philosophy
- Non-Western Philosophy
- Philosophy of Language
- Philosophy of Mind
- Philosophy of Perception
- Philosophy of Action
- Philosophy of Law
- Philosophy of Religion
- Philosophy of Science
- Philosophy of Mathematics and Logic
- Practical Ethics
- Social and Political Philosophy
- Browse content in Religion
- Biblical Studies
- Christianity
- East Asian Religions
- History of Religion
- Judaism and Jewish Studies
- Qumran Studies
- Religion and Education
- Religion and Health
- Religion and Politics
- Religion and Science
- Religion and Law
- Religion and Art, Literature, and Music
- Religious Studies
- Browse content in Society and Culture
- Cookery, Food, and Drink
- Cultural Studies
- Customs and Traditions
- Ethical Issues and Debates
- Hobbies, Games, Arts and Crafts
- Lifestyle, Home, and Garden
- Natural world, Country Life, and Pets
- Popular Beliefs and Controversial Knowledge
- Sports and Outdoor Recreation
- Technology and Society
- Travel and Holiday
- Visual Culture
- Browse content in Law
- Arbitration
- Browse content in Company and Commercial Law
- Commercial Law
- Company Law
- Browse content in Comparative Law
- Systems of Law
- Competition Law
- Browse content in Constitutional and Administrative Law
- Government Powers
- Judicial Review
- Local Government Law
- Military and Defence Law
- Parliamentary and Legislative Practice
- Construction Law
- Contract Law
- Browse content in Criminal Law
- Criminal Procedure
- Criminal Evidence Law
- Sentencing and Punishment
- Employment and Labour Law
- Environment and Energy Law
- Browse content in Financial Law
- Banking Law
- Insolvency Law
- History of Law
- Human Rights and Immigration
- Intellectual Property Law
- Browse content in International Law
- Private International Law and Conflict of Laws
- Public International Law
- IT and Communications Law
- Jurisprudence and Philosophy of Law
- Law and Society
- Law and Politics
- Browse content in Legal System and Practice
- Courts and Procedure
- Legal Skills and Practice
- Primary Sources of Law
- Regulation of Legal Profession
- Medical and Healthcare Law
- Browse content in Policing
- Criminal Investigation and Detection
- Police and Security Services
- Police Procedure and Law
- Police Regional Planning
- Browse content in Property Law
- Personal Property Law
- Study and Revision
- Terrorism and National Security Law
- Browse content in Trusts Law
- Wills and Probate or Succession
- Browse content in Medicine and Health
- Browse content in Allied Health Professions
- Arts Therapies
- Clinical Science
- Dietetics and Nutrition
- Occupational Therapy
- Operating Department Practice
- Physiotherapy
- Radiography
- Speech and Language Therapy
- Browse content in Anaesthetics
- General Anaesthesia
- Neuroanaesthesia
- Clinical Neuroscience
- Browse content in Clinical Medicine
- Acute Medicine
- Cardiovascular Medicine
- Clinical Genetics
- Clinical Pharmacology and Therapeutics
- Dermatology
- Endocrinology and Diabetes
- Gastroenterology
- Genito-urinary Medicine
- Geriatric Medicine
- Infectious Diseases
- Medical Toxicology
- Medical Oncology
- Pain Medicine
- Palliative Medicine
- Rehabilitation Medicine
- Respiratory Medicine and Pulmonology
- Rheumatology
- Sleep Medicine
- Sports and Exercise Medicine
- Community Medical Services
- Critical Care
- Emergency Medicine
- Forensic Medicine
- Haematology
- History of Medicine
- Browse content in Medical Skills
- Clinical Skills
- Communication Skills
- Nursing Skills
- Surgical Skills
- Medical Ethics
- Browse content in Medical Dentistry
- Oral and Maxillofacial Surgery
- Paediatric Dentistry
- Restorative Dentistry and Orthodontics
- Surgical Dentistry
- Medical Statistics and Methodology
- Browse content in Neurology
- Clinical Neurophysiology
- Neuropathology
- Nursing Studies
- Browse content in Obstetrics and Gynaecology
- Gynaecology
- Occupational Medicine
- Ophthalmology
- Otolaryngology (ENT)
- Browse content in Paediatrics
- Neonatology
- Browse content in Pathology
- Chemical Pathology
- Clinical Cytogenetics and Molecular Genetics
- Histopathology
- Medical Microbiology and Virology
- Patient Education and Information
- Browse content in Pharmacology
- Psychopharmacology
- Browse content in Popular Health
- Caring for Others
- Complementary and Alternative Medicine
- Self-help and Personal Development
- Browse content in Preclinical Medicine
- Cell Biology
- Molecular Biology and Genetics
- Reproduction, Growth and Development
- Primary Care
- Professional Development in Medicine
- Browse content in Psychiatry
- Addiction Medicine
- Child and Adolescent Psychiatry
- Forensic Psychiatry
- Learning Disabilities
- Old Age Psychiatry
- Psychotherapy
- Browse content in Public Health and Epidemiology
- Epidemiology
- Public Health
- Browse content in Radiology
- Clinical Radiology
- Interventional Radiology
- Nuclear Medicine
- Radiation Oncology
- Reproductive Medicine
- Browse content in Surgery
- Cardiothoracic Surgery
- Gastro-intestinal and Colorectal Surgery
- General Surgery
- Neurosurgery
- Paediatric Surgery
- Peri-operative Care
- Plastic and Reconstructive Surgery
- Surgical Oncology
- Transplant Surgery
- Trauma and Orthopaedic Surgery
- Vascular Surgery
- Browse content in Science and Mathematics
- Browse content in Biological Sciences
- Aquatic Biology
- Biochemistry
- Bioinformatics and Computational Biology
- Developmental Biology
- Ecology and Conservation
- Evolutionary Biology
- Genetics and Genomics
- Microbiology
- Molecular and Cell Biology
- Natural History
- Plant Sciences and Forestry
- Research Methods in Life Sciences
- Structural Biology
- Systems Biology
- Zoology and Animal Sciences
- Browse content in Chemistry
- Analytical Chemistry
- Computational Chemistry
- Crystallography
- Environmental Chemistry
- Industrial Chemistry
- Inorganic Chemistry
- Materials Chemistry
- Medicinal Chemistry
- Mineralogy and Gems
- Organic Chemistry
- Physical Chemistry
- Polymer Chemistry
- Study and Communication Skills in Chemistry
- Theoretical Chemistry
- Browse content in Computer Science
- Artificial Intelligence
- Computer Architecture and Logic Design
- Game Studies
- Human-Computer Interaction
- Mathematical Theory of Computation
- Programming Languages
- Software Engineering
- Systems Analysis and Design
- Virtual Reality
- Browse content in Computing
- Business Applications
- Computer Games
- Computer Security
- Computer Networking and Communications
- Digital Lifestyle
- Graphical and Digital Media Applications
- Operating Systems
- Browse content in Earth Sciences and Geography
- Atmospheric Sciences
- Environmental Geography
- Geology and the Lithosphere
- Maps and Map-making
- Meteorology and Climatology
- Oceanography and Hydrology
- Palaeontology
- Physical Geography and Topography
- Regional Geography
- Soil Science
- Urban Geography
- Browse content in Engineering and Technology
- Agriculture and Farming
- Biological Engineering
- Civil Engineering, Surveying, and Building
- Electronics and Communications Engineering
- Energy Technology
- Engineering (General)
- Environmental Science, Engineering, and Technology
- History of Engineering and Technology
- Mechanical Engineering and Materials
- Technology of Industrial Chemistry
- Transport Technology and Trades
- Browse content in Environmental Science
- Applied Ecology (Environmental Science)
- Conservation of the Environment (Environmental Science)
- Environmental Sustainability
- Environmentalist Thought and Ideology (Environmental Science)
- Management of Land and Natural Resources (Environmental Science)
- Natural Disasters (Environmental Science)
- Nuclear Issues (Environmental Science)
- Pollution and Threats to the Environment (Environmental Science)
- Social Impact of Environmental Issues (Environmental Science)
- History of Science and Technology
- Browse content in Materials Science
- Ceramics and Glasses
- Composite Materials
- Metals, Alloying, and Corrosion
- Nanotechnology
- Browse content in Mathematics
- Applied Mathematics
- Biomathematics and Statistics
- History of Mathematics
- Mathematical Education
- Mathematical Finance
- Mathematical Analysis
- Numerical and Computational Mathematics
- Probability and Statistics
- Pure Mathematics
- Browse content in Neuroscience
- Cognition and Behavioural Neuroscience
- Development of the Nervous System
- Disorders of the Nervous System
- History of Neuroscience
- Invertebrate Neurobiology
- Molecular and Cellular Systems
- Neuroendocrinology and Autonomic Nervous System
- Neuroscientific Techniques
- Sensory and Motor Systems
- Browse content in Physics
- Astronomy and Astrophysics
- Atomic, Molecular, and Optical Physics
- Biological and Medical Physics
- Classical Mechanics
- Computational Physics
- Condensed Matter Physics
- Electromagnetism, Optics, and Acoustics
- History of Physics
- Mathematical and Statistical Physics
- Measurement Science
- Nuclear Physics
- Particles and Fields
- Plasma Physics
- Quantum Physics
- Relativity and Gravitation
- Semiconductor and Mesoscopic Physics
- Browse content in Psychology
- Affective Sciences
- Clinical Psychology
- Cognitive Psychology
- Cognitive Neuroscience
- Criminal and Forensic Psychology
- Developmental Psychology
- Educational Psychology
- Evolutionary Psychology
- Health Psychology
- History and Systems in Psychology
- Music Psychology
- Neuropsychology
- Organizational Psychology
- Psychological Assessment and Testing
- Psychology of Human-Technology Interaction
- Psychology Professional Development and Training
- Research Methods in Psychology
- Social Psychology
- Browse content in Social Sciences
- Browse content in Anthropology
- Anthropology of Religion
- Human Evolution
- Medical Anthropology
- Physical Anthropology
- Regional Anthropology
- Social and Cultural Anthropology
- Theory and Practice of Anthropology
- Browse content in Business and Management
- Business Ethics
- Business History
- Business Strategy
- Business and Technology
- Business and Government
- Business and the Environment
- Comparative Management
- Corporate Governance
- Corporate Social Responsibility
- Entrepreneurship
- Health Management
- Human Resource Management
- Industrial and Employment Relations
- Industry Studies
- Information and Communication Technologies
- International Business
- Knowledge Management
- Management and Management Techniques
- Operations Management
- Organizational Theory and Behaviour
- Pensions and Pension Management
- Public and Nonprofit Management
- Strategic Management
- Supply Chain Management
- Browse content in Criminology and Criminal Justice
- Criminal Justice
- Criminology
- Forms of Crime
- International and Comparative Criminology
- Youth Violence and Juvenile Justice
- Development Studies
- Browse content in Economics
- Agricultural, Environmental, and Natural Resource Economics
- Asian Economics
- Behavioural Finance
- Behavioural Economics and Neuroeconomics
- Econometrics and Mathematical Economics
- Economic History
- Economic Methodology
- Economic Systems
- Economic Development and Growth
- Financial Markets
- Financial Institutions and Services
- General Economics and Teaching
- Health, Education, and Welfare
- History of Economic Thought
- International Economics
- Labour and Demographic Economics
- Law and Economics
- Macroeconomics and Monetary Economics
- Microeconomics
- Public Economics
- Urban, Rural, and Regional Economics
- Welfare Economics
- Browse content in Education
- Adult Education and Continuous Learning
- Care and Counselling of Students
- Early Childhood and Elementary Education
- Educational Equipment and Technology
- Educational Strategies and Policy
- Higher and Further Education
- Organization and Management of Education
- Philosophy and Theory of Education
- Schools Studies
- Secondary Education
- Teaching of a Specific Subject
- Teaching of Specific Groups and Special Educational Needs
- Teaching Skills and Techniques
- Browse content in Environment
- Applied Ecology (Social Science)
- Climate Change
- Conservation of the Environment (Social Science)
- Environmentalist Thought and Ideology (Social Science)
- Natural Disasters (Environment)
- Social Impact of Environmental Issues (Social Science)
- Browse content in Human Geography
- Cultural Geography
- Economic Geography
- Political Geography
- Browse content in Interdisciplinary Studies
- Communication Studies
- Museums, Libraries, and Information Sciences
- Browse content in Politics
- African Politics
- Asian Politics
- Chinese Politics
- Comparative Politics
- Conflict Politics
- Elections and Electoral Studies
- Environmental Politics
- European Union
- Foreign Policy
- Gender and Politics
- Human Rights and Politics
- Indian Politics
- International Relations
- International Organization (Politics)
- International Political Economy
- Irish Politics
- Latin American Politics
- Middle Eastern Politics
- Political Behaviour
- Political Economy
- Political Institutions
- Political Theory
- Political Methodology
- Political Communication
- Political Philosophy
- Political Sociology
- Politics and Law
- Public Policy
- Public Administration
- Quantitative Political Methodology
- Regional Political Studies
- Russian Politics
- Security Studies
- State and Local Government
- UK Politics
- US Politics
- Browse content in Regional and Area Studies
- African Studies
- Asian Studies
- East Asian Studies
- Japanese Studies
- Latin American Studies
- Middle Eastern Studies
- Native American Studies
- Scottish Studies
- Browse content in Research and Information
- Research Methods
- Browse content in Social Work
- Addictions and Substance Misuse
- Adoption and Fostering
- Care of the Elderly
- Child and Adolescent Social Work
- Couple and Family Social Work
- Developmental and Physical Disabilities Social Work
- Direct Practice and Clinical Social Work
- Emergency Services
- Human Behaviour and the Social Environment
- International and Global Issues in Social Work
- Mental and Behavioural Health
- Social Justice and Human Rights
- Social Policy and Advocacy
- Social Work and Crime and Justice
- Social Work Macro Practice
- Social Work Practice Settings
- Social Work Research and Evidence-based Practice
- Welfare and Benefit Systems
- Browse content in Sociology
- Childhood Studies
- Community Development
- Comparative and Historical Sociology
- Economic Sociology
- Gender and Sexuality
- Gerontology and Ageing
- Health, Illness, and Medicine
- Marriage and the Family
- Migration Studies
- Occupations, Professions, and Work
- Organizations
- Population and Demography
- Race and Ethnicity
- Social Theory
- Social Movements and Social Change
- Social Research and Statistics
- Social Stratification, Inequality, and Mobility
- Sociology of Religion
- Sociology of Education
- Sport and Leisure
- Urban and Rural Studies
- Browse content in Warfare and Defence
- Defence Strategy, Planning, and Research
- Land Forces and Warfare
- Military Administration
- Military Life and Institutions
- Naval Forces and Warfare
- Other Warfare and Defence Issues
- Peace Studies and Conflict Resolution
- Weapons and Equipment

- < Previous chapter
- Next chapter >

9 Arithmetic Word Problem Solving and Mental Representations
Catherine Thevenot, University of Geneva
Pierre Barrouillet, University of Geneva
- Published: 04 August 2014
- Cite Icon Cite
- Permissions Icon Permissions
Arithmetic word problem solving is considered as a testing ground of mathematical achievement, but remains the area of mathematics in which students experience the greatest difficulties. In this chapter, we review recent theoretical and empirical work that could shed light on these difficulties. We first describe the most frequently used classifications of word problems and assess their psychological relevance. Then, we present the main hypotheses concerning the nature of the representations involved in word problems. Some theories assume that problem solving relies on the instantiation of schemas abstracted from recurrently encountered problems of the same relational structure, whereas other theories propose that ad hoc transient mental representations are constructed for each problem encountered. A third part is devoted to the impact of individual differences in calculation, reading comprehension, and more general factors, such as working memory capacity. Finally, we address the issue of enhancing performance in word problem solving.
Introduction
Arithmetic word problems, also called verbal or story problems, are verbal descriptions of numerically quantified situations involving one or several explicit questions. It is tacitly assumed that the answer to these questions must correspond to a numerical value that has to be reached after one or a series of arithmetic operations has been carried out. Arithmetic word problems are of particular importance because they are often considered and used as a privileged pedagogical situation in which students are expected to show their understanding and mastery of previously learned mathematical procedures and concepts. In some sense, world problem solving is seen as the testing ground of mathematical achievement. However, as testified by several international surveys (see for example Fayol, Barrouillet, & Camos, 1997 ), this school exercise remains the area of mathematics in which students experience the greatest difficulties, word problem solving lagging far behind others domains such as the mastery of computational algorithms. In this chapter, we review recent theoretical and empirical studies that have been conducted to classify the different additive and multiplicative problems that can be constructed, to describe the representations and processes involved in their solution, to identify the characteristics of the problems, as well as the individual factors that modulate performance, and to design intervention tools for enhancing performance. As we will see, these studies allow researchers to better understand the difficulties encountered by children and provide practitioners with tools to help children in overcoming them.
To anticipate, a consensus has developed among researchers that a large part of the difficulties children encounter in solving word problems arise from an underlying difficulty in understanding the situation that the text describes. Indeed, solving a word problem does not simply consist in successfully performing arithmetic calculations, but anteriorly in understanding the relational structure in which the numerical values are embedded. In this respect, we will see that the way the text is formulated and presented has a strong impact on the ease with which children and adults grasp this structure and solve the problems. It is the reason why word problems have been traditionally classified according to their semantic characteristics, and more precisely to the relational structure of the situation they refer to. According to this view, even when they require the same arithmetic operation, solving problems that refer to quantities that undergo some transformation would not involve the same cognitive processes as solving problems that refer to comparisons between unchanged quantities. In the first part of this chapter, we describe the most frequently used classifications, and we report the empirical evidence that have been gathered in order to attest for their psychological relevance. The second part of the chapter is devoted to the question of the nature of the representations involved in word problem solving. Do problem solvers progressively extract through practice schematic abstract representations that match the most frequent relational structures and then instantiate these representations with the numerical values of the problem at hand, or do they construct in working memory ad hoc transient mental representations for each word problem encountered? This is of importance because the strategies used probably depend on the nature of the representation constructed. Empirical evidence from developmental and adult studies that favor these opposite conceptions will be reviewed. Of course, performance in arithmetic word problem solving does not entirely depend on the semantic characteristics of the problems, but also on the characteristics of the problem solvers themselves. In a third part, we focus on the impact on performance of individual differences in those abilities that are directly involved in word problem solving, such as calculation and text comprehension, but also in more general cognitive resources, such as working memory capacity. Finally, we address the studies that have focused on methods for enhancing performance in word problem solving, either by making clearer the semantic aspects of the text or by providing students with more appropriate knowledge and strategies. We conclude by pointing what we consider as the main pending questions and suggesting some leads for future research.
Semantic Characteristics of Word Problems
The most famous classification of addition problems has been proposed by Riley, Greeno and Heller (1983) . It is based on the type of semantic relations described in the text. Thus, according to the authors, many problems can be classified into three semantic types: Change, Combine, and Comparison problems. Note that, as acknowledge by Riley et al. (1983) , these categories have been used by several other investigators sometimes using different names ( Nesher, 1981 ; Vergnaud, 1982 ). Change problems describe actions that cause increases or decreases in quantities and the unknown is either the resulting amount (John has 6 marbles. Tom gives him 3 more marbles. How many marbles does John have now?), the amount of the change (John had 4 marbles. Tom gave him some more marbles. Now, John has 6 marbles. How many marbles did Tom give him?), or the starting amount (John had some marbles. Tom gave him 5 marbles. Now, John has 7 marbles. How many marbles did John have at the beginning?). In contrast to such dynamic problems, Combine and Comparison problems are relative to static situations. Combine problems describe two quantities that have to be considered in combination to produce the answer. The question can be relative to the total amount (John has 2 marbles. Peter has 4 marbles. How many marbles do John and Tom have altogether?) or to the amount in one of the subsets (Joe and Tom have 8 marbles altogether. Joe has 3 marbles. How many marbles does Tom have?). Finally, in Compare problems, a Comparison is made between two quantities using the expressions ‘more than’ and ‘less than’. The unknown is either the difference between the two quantities (John has 3 marbles. Tom has 5 marbles. How many marbles does John have less than Tom?), the compared quantity (John has 3 marbles. Tom has 5 more marbles than John. How many marbles does Tom have?), or the referent (John has 3 marbles. He has 5 more marbles less than Tom. How many marbles does Tom have?).
The ecological relevance of this classification is attested by the fact that different problem types are not of equal difficulty, even when they require the same arithmetic operation for solution ( Riley et al., 1983 ). Besides, an improvement in performance for each category of problem is observed through development and education. More concretely, Change problems are easier for Grade 2 and 3 children than for kindergarteners and Grade 1 children. When the question of the problem is relative to the resulting state, problems are quite easy, even for young children. In contrast, when the question is about the starting quantity, children experience more difficulties and successful performance for this category of problems only reach a satisfactory rate in Grade 2 (75%). Similarly, Combine problems are easy for young children when the unknown is the sum of the sets but, when the unknown is one of these sets, children experience great difficulties (only 30% of successful solving in kindergarten and Grade 1). Finally, Compare problems are more difficult than Combine and Change problems.
If Riley et al.’s classification is limited to addition problems, Vergnaud (1983) conducted semantic analyses of multiplication problems. Three different forms of relations between the problem elements are described by the author. The first form (or structure) is called ‘isomorphism of measures’ and consists in direct and simple proportions between two measures or quantities (e.g. Linda wants to share her 12 cakes with her two friends. How many cakes each of the three little girls have?). The second form called ‘product of measures’ describes situations where three measures are involved and the third measure is the product of the first two, as in calculating surfaces or volumes (e.g. What is the surface of a room that is 27 feet long and 12 feet large?). The third form of relations is called ‘multiple proportions’ and corresponds to situations that are essentially similar to the isomorphism of measures structure, but in which several variables are involved (e.g. One cow produces an average of 30 liters of milk per day, each liter of milk costs $1.05. How much will the farmer earn over a 2-week period?).
Greer (1992) also proposed a classification of problems wherein a distinction is made between situations that are psychologically commutative and non-commutative. In non-commutative situations, the multiplier and the multiplicand can be distinguished. In other words, one of the quantities involved in multiplication (i.e. multiplier) is conceptualized as operating on the other (i.e. the multiplicand) to produce the result. Concerning division problems, this implies that two types of divisions can be distinguished: division by multiplier (e.g. A college passed the top 3/5 of its students in an exam. If 48 passed, how many students sat the exam?) and division by multiplicand (e.g. A college passed the top 48 out of 80 students who sat the exam. What fraction of the students passed?). In commutative situations, it is impossible to distinguish between multiplier and multiplicand and, consequently, between the two types of division.
Contrary to addition problems, the ecological validity of multiplication problem classifications has not yet been systematically investigated. This is probably due to the dissimilarities in the classification schemata used by different researchers. However, Vergnaud (1983) showed that children master scalar problems, wherein there is a number of groups or objects having the same number in each group (e.g. 3 children have 4 cookies each. How many cookies do they have altogether?) before Cartesian-product problems (e.g. In the ballroom there are 5 boys and 4 girls. If all the boys dance with all the girls, how many couples will be constituted?) or problems involving conversion of measures (e.g. A foot is 0.3 meters. How many meters are 3 feet?). Therefore, as addition problems, the difficulty of multiplication problems does not simply depend on the nature of the operation that has to be performed. The nature and the structure of the semantic situation in which the problem is embedded play a crucial role in individuals’ performance. This is due to the fact that these factors determine the structure, nature, and complexity of the mental representation that has to be constructed to reach the solution.
The Nature of The Mental Representations in Word Problem Solving
It is generally admitted that children struggle with arithmetic word problems when they experience difficulties in constructing the adequate mental representation of the situation that the text describes ( De Corte & Verschaffel, 1985 ). For instance, Change problems are relatively straightforward because they describe dynamic situations that can be easily mentally simulated. What is represented by children is a sequence of events ordered in time in a unidirectional manner ( Nesher, Greeno, & Riley, 1982 ). In contrast, Compare problems are difficult for children because they are not familiar with the expressions ‘more than’ and ‘less than’ ( De Corte & Verschaffel, 1985 ). Interestingly, if the problem ‘There are 5 birds and 3 worms. How many more birds than worms are there?’ leads to only 25% of correct responses in kindergarten children, reformulating the question into ‘How many birds won’t get a worm?’ leads to 96% of correct responses in children at the same level ( Hudson, 1983 ). Again, compared with the first version, the second version of the problem is easier to represent because the birds and the worms can be concretely or mentally matched to form a correspondence between the two sets. However, as stated above, some non-semantic factors modulate these patterns of results. The situation is more difficult to represent when the amount of change is unknown ( Ibarra & Lindvall, 1982 ) and even more when the start set is unknown ( Garcia, Jimenez, & Hess, 2006 ; Riley et al., 1983 ). The lack of knowledge of the first or intermediate quantity would severely hinder the solving process because problems cannot be represented directly by models.
Interestingly, the semantic characteristics of the problems play a role on children’s performance before and after formal instruction. Indeed, it has been shown that some preschool children can solve arithmetic word problem before any explicit instruction by using informal strategies based on their understanding of the situation described in the text of the problem ( Carpenter & Moser, 1982 ; De Corte & Verschaffel, 1987 ; Ibarra & Lindvall, 1982 ). Children who are successful in solving verbal problems have this ability to develop physical models to serve as an aid to solution ( Lindvall & Ibarra, 1980 ). Carpenter and Moser (1984) showed that the semantic characteristics of subtraction problems strongly shape the way the models are constructed. More precisely, problems such as ‘John has 8 cookies. He gives 3 cookies to his friend Leo. How many cookies does John have left?’ is likely to be solved by young children by a counting down strategy, which consists, in our example, in counting backwards three times from the starting amount of 8. This strategy perfectly models the decrease Change situation described in the problem. In contrast, a problem such as ‘Trudy has 3 cookies. How many more cookies does she have to get so that she has 8 cookies altogether?’ is likely to be solved by a counting up strategy from 3 to 8, which is derived from a model that mimics the situation of joining the missing addend. The answer to the problem is the number of counting words in the sequence. Whereas Carpenter and Moser did not find evidence for a relationship between the semantic structure of addition problems and children’s strategies, it was done by De Corte and Verschaffel (1987) . The authors showed that Change problems such as ‘Lynn has 2 cinema tickets. Her friend Sara gives her 2 more tickets. How many tickets does Lynn have now?’ is likely to be solved through an adding procedure. In this case, the child constructs a set of blocks corresponding to the first number in the problem, then adds to this set a number of blocks corresponding to the second number, and finally counts the total number of blocks. In contrast the Combine problem ‘John has 3 bottles of soda. Tom has 4 bottles. How many bottles do they have altogether’ is more likely to be solved by a joining or a no move strategy, where the child constructs two sets corresponding to the two given numbers and either counts the total number of blocks without physically moving the sets (i.e. no move strategy) or moves the sets together with both hands and finally counts the total number of blocks (i.e. joining strategy).
These studies clearly showed that the semantic of the problems determines the strategy used by young children. Nonetheless, even after instruction and practice, semantic factors still determine strategies. Indeed, it is assumed that through practice, problem schemata would be progressively abstracted from and associated with different semantic categories and would determine the procedures used by individuals to solve the problems.
Schemata and Word Problems
Riley et al. (1983) suggest that schemata have the form of semantic network structures that consist of elements and relations between those elements. For example, a Change problem schema would contain an initial quantity that represents a start set, an event that changes the start set (increase or decrease), and an unknown final quantity or result set. The empty slots in the schema are filled in progressively as soon as the relevant information is read in the text of the problem. When all the empty slots are filled in, specific expressions or propositions triggers a second type of schema (i.e. higher order schemata) that contains the procedure required to solve the problem ( Kintsch & Greeno, 1985 ). For example, in Compare problems, ‘more than’ and ‘less than’ propositions would trigger the More-than and Less-than schemata, respectively, which include a large-set, a small-set and a difference variables to be instantiated by numerical values. Both schemata also include a Difference strategy triggered by the same propositions that allows the comparisons of the two sets by the appropriate arithmetic operation of subtraction.
Arguments in favor of the use of arithmetic word problem schemata have been provided by Lewis and Mayer (1987) . These authors focused on the study of two forms of Compare problems, namely consistent and inconsistent problems. In consistent problems, the unknown variable is the subject of the second sentence that contains a relational term consistent with the necessary arithmetic operation. For example, in the consistent problem ‘John has 3 marbles. Tom has 5 more marbles than Joe. How many marbles does Tom have?’, the expression ‘has more’ is consistent with the fact that an addition is required to solve the problem. In contrast, in inconsistent problems, the unknown variable is the object of the second sentence and the relational term conflicts with the necessary arithmetic operation. In the problem ‘Joe has 3 marbles. He has 5 marbles less than Tom. How many marbles does Tom have?’ the term ‘less’ is inconsistent with the addition that has to be performed to solve the problem. According to the authors, problem solvers come to the task with a set of schemata associated to Compare problems. When the form of the given relational sentence is not consistent with the problem solver’s schema, she or he must mentally rearrange the information. The fact that inconsistent problems are more error prone and take longer to solve than consistent problems is coherent with the author’s interpretation. These findings were extended by Fuson, Carroll, and Landis (1996) who showed that the capacity to solve inconsistent problems represents the fourth level of a progression in conceptualizing and solving Compare problems in first and second graders. In a first level, children can only identify who has more or less without being able to quantify the difference. In a second level, they strongly rely on language cues and solve consistent problems (‘John has 3 marbles. Tom has 5 more marbles than Joe. How many marbles does Tom have?’) better than inconsistent problems and even problems requiring to calculate the difference (‘John has 9 marbles. Tom has 7 marbles. How many marbles does John have more than Tom?’). In a third level, children solve these two kinds of problems, but not yet the tricky inconsistent problems. This slow progression indicates how problems inducing the wrong schemata from misleading language cues are difficult to solve.
This sketchy presentation of the schemata-based approach makes clear that schemas are abstract general frames in which the quantitative elements of the problem (numerical values and relations) constitute the only relevant information needed for successful problem solving. However, the abstract nature of these representational frames makes that the schema approach encounters difficulties in accounting for content and context effects ubiquitous in word problem solving. This limitation prompted the emergence of theories that postulate the construction of representations, such as situation models or mental models, which bring to bear a rich real-word experience that makes them more appropriate to grasp semantic content and context.
Situation Model and Word Problem
Reusser (1989 ; Staub & Reusser, 1995 ) postulates the existence of an Episodic Situation Model that includes functional and temporal features of the story problem. This qualitative model would be activated by the solver before a more logico-mathematical representation of the problem corresponding to the schemas described above. Evidence for the construction of such qualitative model during the solving process has been collected through several studies. Coquin-Viennot and Moreau (2003) showed that a problem such as ‘For a prize-giving the florist prepares for each of the 14 candidates 5 roses and 7 tulips. How many flowers does the florist use in total?’ is likely to be solved by children with a distributed strategy consisting in performing (14 × 5) + (14 × 7). However, when formulated as ‘For a prize-giving, the florist prepares for each of the 14 candidates a bouquet composed of 5 roses and 7 tulips. How many flowers does the florist use in total?’ the problem is more likely to be solved with a factorized strategy, by performing 14 × (5 + 7). In the second formulation of the problem, the term ‘bouquet’ plays a structuring role in the organization of the elements contained in the mental representation constructed by children to solve the problem. This result is difficult to interpret within the schema framework, and Coquin-Viennot and Moreau concluded that less formal representations based on real-world knowledge necessarily come into play during the resolution. Similar results were obtained by Bassok, Chase, and Martin (1998) who showed that, when college students construct mathematical problems, they align the mathematical relations between arguments of arithmetic operations with the semantic relations that are evoked by the pairs of objects they are asked to include in the problems. More concretely, division involves an asymmetric mathematical relation between dividend and divisor (a/b ≠ b/a). Bassok et al.’s participants applied this asymmetric operation to object sets that readily evoked functionally asymmetric semantic relations (e.g. a apples and b baskets), but refrained from dividing object sets that did not evoke functionally asymmetric relations (e.g. a apples and b oranges). Instead, they usually related such functionally symmetric sets with the mathematically symmetric operation of addition ( a + b = b + a ).
In addition to real-world knowledge, it has also been shown that characteristics, such as the size of numbers used in the problem can affect the strategies selected by individuals. Thevenot and Oakhill (2005) presented adults with segmented problems such as ‘How many marbles does John have more than Tom and Paul altogether?/John has 49 marbles./Tom has 24 marbles./Paul has 17 marbles./(the ‘/’s represent the boundaries of the segment)’. When a segment appeared, the previous one disappeared. The strategy preferred by adults to solve the problem was to calculate: 49 – (24 + 17), whereas an alternative sequential strategy that is far less demanding could have been possible to implement, namely (49 – 24) – 17. Nevertheless, when three-digit instead or two-digit numbers were used in the text (How many marbles does John have more than Tom and Paul altogether? / John has 649 marbles./Tom has 324 marbles./ Paul has 217 marbles./’), the alternative strategy consisting in two successive subtractions was preferred by participants. Obviously, the difficulty of the task pushed individuals to construct an alternative representation to the one directly induced by the wording of the problem. Again, these results are difficult to reconcile with a pure schema-based approach in which the use of different strategies for a specific type of problem is difficult to interpret. Still, as stated above, it is not to say that schemata did not play any role in the course of the solution process, but rather that a transitional representation between the text problem and a pure mathematical mental representation comes into play.
Mental Models and Word Problems
Nevertheless, some studies might more fundamentally question the systematic resort to problem schema in children and adults. It was shown that placing the question before a story problem rather than classically presenting it at the end facilitates problem solution in children. In the seminal studies, this finding was first attributed to mobilization by problem solvers of the appropriate schema ( Devidal, Fayol, & Barrouillet, 1997 ; Fayol, Abdi, & Gombert, 1987 ). In a standard word problem, the question often contains all the information needed to describe the relations between the different elements of the text as well as specific linguistic expressions. Therefore, according to Devidal et al. (1997) , the question placed at the beginning of the text, via the specific information it contains, directly triggers the appropriate schema (e.g. the expression ‘less than’ will trigger a Comparison schema). This early activation allows the numerical data to be integrated into the schema as soon as they are encountered, and the calculations can be achieved during reading. The resulting release of working memory resources would explain the improvement in performance. However, Thevenot, Barrouillet, and Fayol (2004) confirmed the facilitatory effect of the question at the beginning, but showed that this effect is observable whether or not the calculations are achieved during reading. Consequently, the increase in the number of successful resolutions when the question is placed before, rather than after, the text cannot be attributable only to the on-line integration of the numerical data into the activated schema. The authors proposed that the aid provided by the position of the question before the text would only be due to its facilitatory effect on the construction of the representation required to solve the problem. Just like a title before a narrative text ( Rawson & Kintsch, 2002 ), the question before an arithmetic problem would facilitate the subsequent encoding and integration of the information in the representation. This interpretation is alternative to the one formulated within the Schema framework and is compatible with an approach that considers the representation as a specific and temporary mental structure constructed in working memory, such as the Situation Model approach described above ( Reusser, 1989 ) or the Mental Model theoretical framework ( Johnson-Laird, 1983 ).
This interpretation was confirmed by Thevenot, Devidal, Barrouillet, and Fayol (2007) who showed that the facilitatory effect of the question before the text was more pronounced for children with poor abilities in mathematics. These results are not compatible with the schema framework. If the facilitatory effect of the question before the text was due to the early activation of the appropriate schema, high-skilled children would be those who are more likely to benefit from this effect. Indeed, these children are more often successful in solving problems and, consequently, are more likely to have extracted schemas. As stated above, the reverse result was obtained: it were the low-skilled children who benefited more from the position of the question before the problem. While the simple fact that high-skilled children are not those who benefit more from the position of the question before the text is sufficient to rule out the Schema interpretation, the fact that the inverse result is obtained supports the Mental Model theory. We know that low-skilled children are those children who experience the highest difficulty in the construction of the Mental Model of the situation ( Cain & Oakhill, 1999 ; Oakhill, 1996 ; Oakhill, Cain, & Yuill, 1998 ; Yuill, Oakhill, & Parkin, 1989 ). Therefore, it seems natural that they are the same children who benefit more from an aid to the construction of the representation. Moreover, Thevenot et al. (2007) showed that the facilitatory effect of the question before the text was more pronounced for difficult than easier problems. Again, this result was not compatible with the Schema theory. Difficult problems, which by definition are often failed, are less likely to be associated with the appropriate schema than easier problems, which are often correctly solved. Therefore, the higher facilitatory effect for difficult problems cannot be attributable to the early activation of a problem schema.
The relative relevance of Mental Model and Schemas in the domain of story problems was also directly tested by Thevenot (2010) . In her experiment, adults were asked to solve Compare problems wherein the question took a form such as ‘How many marbles does Louis have more than Jean?’ After the solving phase, an unexpected task of problem recognition was proposed to participants. They were presented either with the original problems, with inconsistent problems that had never been solved, or with paraphrases that respected the relational structure of the original problems, but not their exact wording. These paraphrases were constructed by inverting the terms and the linguistic expressions in the original problems (our example became ‘How many marbles does Jean have less than Louis?). Inconsistent problems were constructed by keeping the same relational terms as in the original problem, but by inverting the names of the protagonists (i.e. ‘How many marbles does Jean have more than Louis’). Whereas the literal form of paraphrastic problems bore the least resemblance to original problems, paraphrastic problems were associated to higher recognition rates than inconsistent problems. These results provided strong evidence that it is the structure of the arithmetic word problem and not its exact wording or its propositional level of representation that is mentally represented by individuals engaged in a resolution task. This is perfectly coherent with the mental model theory proposed by Johnson-Laird (1983 ; see Mani & Johnson-Laird, 1982 , for a similar demonstration in the domain of spatial reasoning), and at odds with the approach postulating that the relational expression (‘have more’ in our example) plays a crucial role in triggering the appropriate schema or procedure.
These results also fit nicely with the Situation Strategy First framework proposed by Brissiaud and Sander (2010) , which posits that the initial mental representation constructed from a problem text activates a situation-based strategy even after instruction (see also Brissiaud, 1994 ). It would be only when it is not efficient for providing the solution that the representation would be modified so that the relevant arithmetic knowledge might be used. For example, the situation-based strategy for a problem such as ‘Luc is playing with 42 marbles. During the game, he loses 39 marbles. How many marbles does Luc have now?’ would consist in counting backward from 42, which is cognitively demanding. In order to gain in efficiency, children would have to ‘re-represent’ the problem in order to apply a mental arithmetic strategy consisting in counting forward from 39 to 42. In accordance with their Situation Strategy First model, the authors show that such problems are indeed more difficult for 7 – and 8-year-old children than problems where the situation-based strategy directly corresponds to the most economic strategy (i.e. ‘Luc is playing with 42 marbles. During the game he loses 3 marbles. How many marbles does Luc have now?’).
This series of works, which provides evidence that schemata do not always constitute the core of the mental representation constructed by individuals, questions assumptions relative to the source of age-related increase in performance. According to Judd and Bilsky (1989) , young children experience difficulties with word problems because they do not yet have a repertoire of highly automatized schemata for representing the different problem types. However, it seems that schemata are not always necessarily convoked to solve familiar problems. Thus, other sources of difficulties have to be considered, such as limited working memory capacities, reading and text comprehension difficulties, or insufficient mastery of number facts and calculation procedures.
Individuals’ Factors Impacting Arithmetic Word Problem Performance
We have seen that arithmetic word problem solving is a complex activity that requires a series of cognitive processes involving the comprehension of a text, the instantiation of abstract representations with current information or the construction of ad hoc mental models, as well as reasoning activities to select appropriate arithmetic operations. Once these operations have been identified, calculation of the answers often requires selection, running, and control of algorithmic procedures that necessitate retrieval of relevant arithmetic facts from long-term memory and temporary storage of intermediary results. These activities thus involve manipulation and maintenance of verbal, but also visuospatial information, as well as the selection and control of procedures. Thus, arithmetic problem solving is a complex cognitive activity that would involve all the components of working memory. Accordingly, several studies have investigated the role of working memory in word problem solving and how individual differences in working memory capacity affect performance.
Working Memory
The relationship between mathematical cognition and working memory has been extensively studied, especially in the domain of arithmetic problem solving (see DeStefano & LeFevre, 2004 ; LeFevre, DeStefano, Coleman, & Shanahan, 2005 , for reviews). In the same way, several studies have provided support for the importance of working memory (WM) in word problem solving. Most of them used the theoretical framework provided by Baddeley’s (1986) multi-component model and aimed at identifying the specific contribution of the central executive, which is considered to be primarily responsible for coordinating activity within the cognitive system, the phonological loop that assumes the storage of verbal material, and the visuospatial sketchpad devoted to the maintenance of visual and spatial information. Among these components, the phonological loop can be expected to play a crucial role in solving arithmetic problems presented through text. Accordingly, Passolunghi and Siegel (2001) showed that 9-year-old children (Grade 4) who are poor at problem solving have difficulties with digit span, which is often used as a measure of the phonological loop. Rasmussen and Bisanz (2005) further supported the notion that, for school-age children, phonological WM is what differentiates between poor and good verbal arithmetic performers. However, Passolunghi and Siegel (2001) also identified difficulties with central executive tests of WM in children who are poor at problem solving. Several other studies by Passolunghi shed light on the role of the central executive. For example, Passolunghi, Cornoldi, and De Liberto (1999) found that Grade 4 children who present difficulties in arithmetic word problem solving also present difficulties in working memory tasks that require the inhibition of irrelevant information. Passolunghi and Pazzaglia (2004) investigated the role of memory-updating, a central process needed to active the relevant information while removing no longer relevant information from WM (this capacity is, for example, assessed by asking children to recall the five smallest animals at the end of the following list of items presented successively: hare , tiger, game, type, mole, fly , fox, return, mosquito , idea, answer, and spider ). They observed that a group of fourth-graders with high memory-updating ability performed better in problem solving compared with a group of low memory-updating ability. Andersson (2007) extended these results by demonstrating that three different measures related with the central executive contributed unique variance to word problem solving in children in grades 2, 3, and 4 – the capacity to coordinate processing and storage, to shift attention from one task to another, and to access knowledge from long-term memory. This relation holds even when the influence of reading, age, and IQ were controlled. The involvement of central executive does not seem to be restricted to arithmetic word problems and several studies have found that this component of WM also contributes significant variance in predicting algebraic word problem solving in older children ( Lee, Ng, & Ng, 2009 ; Lee, Ng, Ng, & Lim, 2004 ).
Studies that have addressed the possible implication of the visuospatial sketchpad are rarer. Nonetheless, Passolunghi and Mammarella (2010) observed that fourth graders who are poor problem solvers exhibited specific difficulties in spatial WM assessed through a Corsi block and a spatial matrix tasks, but not visual working memory. It is worth noting that, in this study, the authors did not found any relation with verbal memory. A more extensive investigation has recently been conducted by Zheng, Swanson, and Marcoulides (2011) who assessed the three components of WM along with reading and mathematical achievement in a large sample of 310 children in grades 2, 3, and 4. The results indicated that the three components predicted word-problem solving performance. Interestingly, reading and calculation proficiency mediated the effect of verbal WM and central executive, whereas academic achievement did not mediate the relationship between visuospatial WM and word problem solving, a result that echoes Passolunghi and Mammarella’s (2010) observation.
In summary, there is a strong consensus that WM plays a crucial role in word problem solving and that individual differences in WM capacity underlie at least a part of individual differences in performance. Importantly, the three components of WM have been identified as having a specific impact, though reading abilities could act as a moderating factor, a point that will be addressed below. Although the studies reported here do not leave many doubts about the involvement of WM in the complex activity that word problem solving is, they have also the common characteristic of assessing correlations between abilities and capacities measured at a given moment in development. As such, they provide only indirect evidence concerning the question of the role of WM in the development of problem solving abilities. Interestingly, Swanson (2011) addressed the question of whether the development in problem solving abilities is related to growth in WM capacity. The results showed that WM performance in Grade 1 contributes approximately 26% of the variance to problem-solving accuracy in Grade 3. The significant contribution of WM to word problem-solving accuracy was maintained when measures of attention and long-term memory capacities were entered into the regression analysis. Swanson’s results also showed that Grade 1 performance on measures of naming speed (see also Kail, 2007 , for similar results) and inhibition (see Passolunghi et al., 1999 ) contribute unique variance to Grade 3 problem-solving performance. Finally, the growth curve analysis showed that growth on the executive component of WM was related to growth in word problem solving. Thus, the executive system (controlled attention) and not measures of storage (phonological loop) were related to growth in word problem solving, suggesting that this skill and its development strongly solicits the central cognitive system in charge of coordinating cognitive activities.
Reading and Text Comprehension Abilities
When thinking about the capacities needed to solve arithmetic word problems, reading comprehension and calculation skills are among the first abilities that come to mind. Indeed, solving word problems requires, as a minimum, comprehending the text, storing facts during solution, and performing calculations on the stored numbers. Thus, along with WM, reading comprehension and calculation skills should be among the main determinants of word problem-solving performance. Accordingly, several studies have reported a role of these factors. For example, Kail and Hall (1999) measured reading skills, arithmetic knowledge, and short-term memory span in 8–12-year-old children who were asked to solve Change, Equalize, Combine, and Compare problems. Not surprisingly, knowledge of basic arithmetic facts had a strong impact on word problem performance, but reading abilities contributed also consistently and strongly, although these abilities were assessed through a simple word recognition task, whereas memory was a less consistent predictor of word-problem performance. This latter finding has been reported in several studies. For example Swanson, Cooney, and Brok (1993) observed that the correlation between WM and word-problem performance became non significant when reading comprehension was partialed out. This suggests that reading comprehension takes precedence over memory, a phenomenon also observed by Fuchs and colleagues ( Fuchs, Fuchs, Compton, Powell, Seethaler, Capizzi, et al., 2006 ) who found that, when word-reading proficiency is entered in the regression model, WM no longer plays a significant role in predicting children’s word problem accuracy (see Lee et al., 2004 , for similar findings in the domain of algebraic word problems).
A number of following studies confirmed the impact of reading comprehension skills on problem solving. We mentioned above the study by Andersson (2007) who assessed a variety of WM capacities in relation with word problem-solving performance, but also arithmetic calculation and reading skills. Although the main conclusion of the authors were related with the unique contribution of WM capacities, the best predictor of arithmetic word-problem solving was, as Kail and Hall (1999) observed, arithmetic calculation ( r = 0.69) immediately followed by reading ability ( r = 0.56). In the same way, Zheng et al., (2011) investigated the specific role of several components of WM as we reported above, but they also assessed calculation capacities and reading comprehension. Once more, arithmetic calculation was the best predictor of word problem solving, followed by reading comprehension. Interestingly, this study also revealed that all the components of WM predict word problem performance, but that reading abilities can compensate for some of the influence of WM in children. It is worth to note that comprehension per se is the key aspect of the relationship between reading abilities and word problem solving. Pinperton and Nation (2010) studied the mathematical profile of 7–8-year-old poor comprehenders, who are children who show significant deficits in reading comprehension despite average, or above-average, word-reading ability. When compared with controls showing age-appropriate reading comprehension abilities, poor comprehenders showed a deficit in mathematical reasoning assessed through verbally presented problems, whereas they did not differ from their controls in solving numerical operations.
Although the relationship between reading comprehension and word problem solving seems straightforward, it could be more complex than expected. A first interpretation could be that better reading comprehension facilitates the construction of a correct representation of the situation the problem describes, a correct representation which is necessary for solving the problem. However, as Kail and Hall (1999 , p. 667) noted, another interpretation could be that because reading and mathematics achievement are correlated ( Geary, 1993 ; Jordan, Kaplan, & Hanish, 2002 ), reading comprehension may simply be a proxy for general mathematical skills, including word problem solving. This latter interpretation is reinforced by Lerkkanen, Rasku-Puttonen, Aunola, and Nurmi (2005) . They observed in a longitudinal study that mathematics and reading comprehension are highly associated during the first and second years of primary school, but, surprisingly, it appeared that mathematical performance predicted reading comprehension rather than vice versa. Though Lerkkanen et al. (2005) did not assess word problem solving performance, their finding suggests that the correlation observed by Kail and Hall (1999) does not necessarily reveal a causal relationship from reading comprehension to word problem solving performance.
Surprisingly, some studies focusing on groups of children suffering from learning disabilities have reached the same temperate conclusions. Vukovic, Lesaux, and Siegel (2010) studied mathematical skills of third graders who present two types of reading difficulties, either a dyslexia or specific reading comprehension difficulties. When contrasted with a large control group, the two groups presenting reading difficulties performed lower than the control group on word problems. However, once mastery of arithmetic facts was controlled, children with reading difficulties performed in the average range, suggesting that reading difficulties have no effect per se in word problem-solving performance. Nonetheless, as the authors note, the problems were read to the children, eliminating the reading demand of the task and, despite this aid, children with reading difficulties performed lower than the control group. In fact, several studies have shown that children who have difficulties in both mathematics and reading perform worse in word problems than children who have difficulties in mathematics only ( Jordan & Hanish, 2000 ; Jordan & Montani, 1997 ).
To summarize, many studies have established that, after fluency in arithmetic fact and calculation abilities, reading comprehension is a strong predictor of word problem-solving performance. Even if the directionality of the causal link remains uncertain and the specific contribution of reading comprehension is difficult to isolate, reading difficulties contribute to a lower achievement in word problem solving. Alternatively, it seems that reading abilities can compensate for the influence of more general cognitive traits like WM capacity. In the next section, we address the studies that have explicitly focused on those factors susceptible to improve performance.
Enhancing Word Problem Performance
Schema-based instruction (sbi).
As noted by Jitendra, Griffin, Deatline-Buchman, and Sczesniak (2007) , traditional instruction teaches students to use keywords in order to solve verbal problems. For example, ‘all’ suggests addition, whereas ‘share’ suggests division. This approach is limited because many problems do not have keywords. Moreover, as already noted when commenting on inconsistent problems, basing the solving process on keywords can be misleading and does not help students to make sense of problem situations. In order to better promote elementary students’ mathematical problem solving skills, Jitendra and her colleagues (e.g. Jitendra et al., 2007 ; Jitendra & Hoff, 1996 ; Jitendra & Star, 2011 ) designed an intervention tool called the schema-based instruction (SBI). SBI promotes the explicit analysis of the problem schema (e.g. part-part-whole) and the relationships between its different elements (e.g. parts make up the whole). The rationale behind SBI is that understanding these relationships is crucial in selecting appropriate operations needed to solve the problems. In fact, ‘SBI allows students to approach the problem by focusing on the underlying problem structure, thus facilitating conceptual understanding and adequate word-problem-solving skills’ ( Jitendra et al., 2007 , p. 286). The instruction program consists of asking children to read and retell the problem to discover its type (Change, Combine, or Comparison, for example, for addition problems), to underline and map important information onto a schematic diagram, to decide which operation has to be performed, to write the mathematical sentence and the answer, and finally to check it. Such program conducted in low – and high-ability, as well as special education classrooms revealed performance improvement from pretest to posttest on word problem solving and computation fluency measures.
Using Manipulatives
As seen above, capacities in WM can be related to word problem performance. Therefore, decreasing the WM demand of a problem by using external aids such as manipulatives could help children to solve it. Manipulative materials, such as Cuisenaire rods or craft sticks, are concrete models that incorporate mathematical concepts, and that can be touched and moved around by students. They can be used by children in order to represent the objects and their relationships described in the text. Then, using manipulatives may be beneficial in constructing an adequate situation or mental model of the problem. Indeed, providing kindergarten children with manipulatives enable them to solve simple word problem ( Carpenter, Hiebert, & Moser, 1983 ). However, Stellingwerf and Van Lieshout (1999) showed that 11-year-old children from schools for special education do not improve their performance on word problem after a training in which only manipulatives were used. The authors conclude that, whereas young children are helped by the use of manipulatives ( Carpenter et al., 1983 ), older ones do not benefit any longer from such instructional tools. Stellingwerf and Van Lieshout also demonstrated the existence of a transitional stage (i.e. between the age of 6 and 11) in which manipulatives are helpful when used in combination with other educational tools such as the use of number sentences (e.g. a +? = c ).
Rewording The Problems
As already stated above, reformulating the problem can dramatically improve children performance. We saw that whereas the problem ‘There are 5 birds and 3 worms. How many more birds than worms are there?’ leads to only 25% of correct response in kindergarten children, a reformulation into ‘How many birds won’t get a worm?’ leads to 96% of correct response in children at the same level ( Hudson, 1983 ). According to Staub and Reusser (1995) , the two versions of the problem refer to different episodic situation models. Whereas the first version describes a static and abstract situation, the second version describes a dynamic and more concrete situation. As a consequence, everyday and real-world knowledge can easily be applied during the course of the comprehension and solving processes, hence the improvement in performance. Likewise, Stern and Lehrndofer (1992) recorded better results on Compare problems when they added qualitative information to the text. For example, in a problem such as ‘How many fewer pencils does Laura have than Peter?’, adding that Peter is the older brother and, as such, enjoys a number of advantage, helps children in their understanding of the problem. However, other studies failed to obtain such effects. Cummins, Kintsch, Reusser, & Weimer (1988) compared second graders’ comprehension and solution of standard word problems with enriched problems embedded into little stories showing plausible and realistic situations. For example, the problem ‘Joe has 8 dollars. He has 5 more dollars than Tom. How many dollars does Tom have?’ was reformulated into ‘Joe and Tom play tennis together twice a week. They both always try hard to beat each other. Both of them decided to buy new tennis balls. So far Joe has saved 8 dollars for his balls. He has saved 5 dollars more than Tom. How many dollars has Tom saved?’ Contrary to the author’s expectations, the reworded problems did not elicit significantly higher solution performance than the standard ones.
As a matter of fact, Vicente, Orrantia, and Verschaffel (2007) have shown recently that such situational rewordings are, indeed, not always very efficient. In contrast, conceptual rewording, which consists of the enhancement of the underlying semantic structure of the problem, more systematically elicits positive effects. It is the case in De Corte, Verschaffel, and de Win’s study (1985) , where the semantic relationship between the sets implied in the problem are stated more transparently. First and second graders were asked to solve Change, Combine, and Compare problems, either in their classical form, or in a reworded way. The problems were reformulated in such a way that the relations between the given and the unknown sets were made more explicit. However, the underlying mathematical structure of the problems was not affected. For example, a sentence relative to the initial situation was added at the beginning of Change problems (‘Joe had some marbles. He won 3 more marbles. Now he has 5 marbles. How many marbles did Joe have at the beginning?’) or the part-whole relation was made explicit in Combine problems (‘Tom and Ann have 9 nuts altogether. Three nuts belong to Tom. The rest belong to Ann. How many marbles does Ann have?’). Such conceptual rewordings facilitated the solving process in both age groups, but especially in younger children. The interpretation of Verschaffel, De Corte, and De Win (1985) was that their reformulations helped children in constructing the adequate mental representation of the problem, especially in children whose canonical schemata are not fully developed and, therefore, depend more on text-driven (bottom-up) processing to construct their mental representation.
Using Pictures, Graphs, and Figures
Using pictures or self-generated drawings tends to facilitate the solution of word problems. Willis and Fuson (1988) showed that asking children to choose a schematic drawing that matches the situational structure of a given problem, then to fill the problem numbers into appropriate locations in the drawing and, finally, to use the drawing in order to decide the solution procedure is an efficient mean to improve performance in second-graders. Children from high and average-achieving classrooms were generally accurate in choosing the correct drawing for Combine, Change, and Compare problems. According to the authors, this technique is useful because it helps children to grasp the semantic structure of the problem situation. Sprinthall and Nolan (1991) added that the structure of Combine problems is made even more salient when different objects are introduced within the pictures. For example, it is more efficient to present first-grade children with pictures representing 5 tulips and 2 roses than representing the same objects: 5 tulips and 2 tulips. The pictures or drawing representing different objects would present clearer visual arrays, which would allow students to form contrasting perceptions of the quantities in each set and, consequently, would help them to grasp more easily the semantic structure of the problem.
However, as for rewording, Vicente, Orrantia, and Verschaffel (2008) showed that only conceptual (mathematical) drawings and not situational ones are efficient. Conceptual drawings make salient the mathematical organization, whereas situational drawings highlight the components of the situation such as the temporal sequence of events or the context in which the story is embedded. Vicente et al. (2008) asked children with high and low abilities in arithmetic to solve easy and difficult problems. The former could be solved following the time sequence described in the text, whereas for the latter it was necessary to solve the second part of the problem before its first part in order to reach the solution (e.g. ‘Laura had 47 beads. She bought some more beads. She used 126 beads and she was left with 11 beads. How many beads did she buy?’). The problems could be presented in their standard form (as in the example), with extra mathematical information that highlighted the part-whole relation described in the problem (‘Laura had 47 beads. She bought some more beads and put them together with the beads she already had. From the total amount of beads she had after buying some , she used 126 beads and she was left with 11 beads. How many beads did she buy?’), or with extra situational information that emphasized the temporal sequence of events described in the problem (‘ Two days ago , Laura had 47 beads. Yesterday , she bought some more beads. This morning she decided to make a collar for her mother. To do so , she used 126 beads and, when she finished , she was left with 11 beads. How many beads did she buy?’). The problems could be presented by themselves or along with a drawing. Drawings that were presented with standard problems did not contribute to the understanding of the conceptual or mathematical situation of the problem but merely represented the protagonist(s) and the objects described in the problem. The mathematical drawing represented the part-whole relation described in the problem. It was constituted of two pictures, one for each part of the Change situation. The first picture depicted the reunion of the two parts into the whole, for example, Figure 9.1 .
Each part of the Change situation.
The second picture showed that the initial total amount of objects can be portioned into two parts, for example, Figure 9.2 .
Finally, situational drawings corresponded to a kind of comic strip in which each of the frames represented a temporal window described in the problem. In line with previous research (see the above section about rewording), the authors showed that when no drawing was presented with the problems, the situational and the mathematical versions of the problems elicited more correct answers than the standard versions. This was especially true for difficult problems. More importantly for our purpose, when the problem was accompanied with a mathematical drawing, children’s rate of correct answers was greater compared with a situation in which a standard or situational drawing was presented. However, and interestingly, only children with higher abilities in mathematics benefited from the presentation of mathematical drawings. The interpretation of the authors concerning this last finding was that only children with high abilities have the necessary skills and cognitive resources to process the mathematical drawing efficiently.
Initial total amount of objects portioned into two parts.
Conclusions and Directions
The main conclusion that could be drawn from this rapid survey of the literature on arithmetic word problem solving during the last decades is that this research domain has progressively moved towards an increasing emphasis of the role of semantically rich and complex representations. If the seminal works on this domain and the first semantic classifications could lend credence to a word problem-solving activity relying on a restricted and finite number of definite schemas progressively abstracted through repeated exposition with problems, the difficulties that this view encountered in accounting for ubiquitous effects of content and context brought about the necessity of envision more complex representations and associated cognitive processes. Instead of retrieving abstract schemata from long-term memory that would be readily instantiated with the available numerical values before running the associated calculation, problems solvers seem to construct ad hoc transient representations (mental models or situation models) that involve a fairly rich real-world knowledge. However, the level of abstractness of representations and procedures remains a controversial issue in research on numerical and arithmetic processes (see Cohen Kadosh & Walsh, 2009 ; Landy & Goldstone, 2007 ), and the widespread use of context-bound representations does not mean that more abstract mathematical representations cannot emerge from these initial representations and direct further calculation. Nonetheless, empirical evidence indicates that semantic and pragmatic aspects influence the entire process of problem solving. The difficulty of constructing these complex representations from text reading, and the necessity to maintain them in an active state, while selecting and performing appropriate calculations, probably explains why, along with trivial factors, such as calculation proficiency and reading comprehension, working memory capacities are so good predictors of performance. This is also probably why, among the variety of interventions and tutorials that have been imagined and tested, such as rewordings or use of drawings, those that emphasize the conceptual characteristics of the problems are the most efficient. Arithmetic word problem solving thus appears as a highly complex cognitive activity that mobilizes a variety of conceptual knowledge and procedural skills, and it does not come as a surprise that it constitutes the domain in which pupils experience their main difficulties.
As other high-level cognitive activities, such as reasoning, decision making, or expertise acquisition, the complexity of the activity of arithmetic word problem solving makes its study especially arduous for cognitive and educational psychologists. However, we would like to conclude by suggesting some leads for future research. The present review of the literature made probably clear that developmental studies have up to now focused on relatively restricted ranges of ages and degrees. Nonetheless, studies that would encompass the complete developmental era or the educational curriculum, say from kindergarten age to adulthood, would be of interest. Indeed, very little is known about how strategies and their underlying representations evolve in the long run. It is possible that the use of manipulatives to model problem situations in young children is progressively interiorized into some mental schemas that would coexist with the use of semantic representations like mental or situation models, enriching children’s toolbox. It is also possible that these semantic representations evolve themselves into more abstract representations that could direct problem solving, in the same way as experts in several domains have memorized an impressive list of strategies in response to a variety of recurrent situations. It cannot be excluded that these developmental changes interact with content and contexts, rendering developmental trends even more complex. For example, contents that facilitate the construction of mental images, such as problems involving count nouns, could more easily elicit mental models than problems involving mass nouns that could more efficiently trigger schema retrieval due to their low level of imageability. In other words, it might be that problem solvers mobilize at each developmental level a variety of strategies varying in representational format and level of abstraction, in the same way as children and adults use a variety of strategies, even for solving very simple arithmetic problems such as elementary additions or subtractions ( Siegler, 1996 ). Another necessary extension of the current research concerns the variety of the problems studied. As the present review made clear, and apart from rare attempts, the range of the problems studied has up to now remained restricted to the simplest forms of the additive problems. The ecological and psychological validity of more extended and complex classifications requires to be established. More complex problems might reveal even more elaborated strategies involving an increased variety of representational formats. Finally, psychologists should not forget that arithmetic word problem solving is not only a complex cognitive activity that constitutes a privileged tool for exploring high-level cognitive processes, but also a school activity and one of the main pedagogical tools for mathematics teaching. In this respect, a better understanding of the underlying factors that make this activity so difficult, and of the determinants of individual difficulties is needed that could help to promote more rational teaching curricula and more efficient intervention programs.
Andersson, U. ( 2007 ). The contribution of working memory to children’s mathematical word problem solving. Applied Cognitive Psychology, 21, 1201–1216.
Google Scholar
Baddeley, A. ( 1986 ). Working Memory. New York: Oxford University Press.
Google Preview
Bassok, M. , Chase, V.M. , & Martin, S.A. ( 1998 ). Adding apples and oranges: alignment of semantic and formal knowledge. Cognitive Psychology, 35, 99–134.
Brissiaud, R. ( 1994 ). Teaching and development: solving ‘missing addend’ problems using subtraction. European Journal of Psychology of Education, 4, 343–365.
Brissiaud, R. , & Sander, E. ( 2010 ). Arithmetic word problem solving: a Situation Strategy First Framework. Developmental Science, 13(1), 92–107.
Cain, K. , & Oakhill, J. ( 1999 ). Inference making ability and its relation to comprehension failure in young children. Reading and Writing, 11, 489–503.
Carpenter, T.P. , Hiebert, J. , & Moser, J.M. ( 1983 ). Problem structure and first-grade-children’s initial solution processes for simple addition and subtraction problems. Journal for Research in Mathematics Education, 12, 27–39.
Carpenter, T.P. , & Moser, J.M. ( 1982 ). The development of addition and subtraction problem-solving skills. In T. P Carpenter , J. M. Moser , & T. A. Romberg (Eds), Addition and Subtraction: A Cognitive Perspective (pp. 9–25). Hillsdale: Erlbaum.
Carpenter, T.P. , & Moser, J.M. ( 1984 ). The acquisition of addition and subtraction concepts in grades one through three. Journal for Research in Mathematics Education, 15, 179–202.
Cohen Kadosh, R. , & Walsh, V ( 2009 ). Numerical representation in the parietal lobes: abstract or not abstract? Behavioral and Brain Sciences, 32(3–4): 313–28.
Coquin-Viennot, D. , & Moreau, S. ( 2003 ). Highlighting the role of the episodic situation model in the solving of arithmetical problems. European Journal of Psychology of Education, 18, 267–279.
Cummins, D. , Kintsch, W. , Reusser, K. , & Weimer, R. ( 1988 ). The role of understanding in solving word problems. Cognitive Psychology, 20, 405–438.
De Corte, E. , & Verschaffel, L. ( 1985 ). Beginning first graders’ initial representation of arithmetic word problems. Journal of Mathematical Behavior, 4, 3–21.
De Corte, E. , & Verschaffel, L. ( 1987 ). The effect of semantic structure on first grader’s strategies for solving addition and subtraction word problems. Journal for Research in Mathematics Education, 18, 363–381.
De Corte, E. , Verschaffel, L. , & De Win, L. ( 1985 ). Influence of rewording verbal problems on children’s problem representations and solutions. Journal of Educational Psychology, 77, 460–470.
DeStefano, D. , & LeFevre, J. ( 2004 ). The role of working memory in mental arithmetic. European Journal of Cognitive Psychology, 16, 353–386.
Devidal, M. , Fayol, M. & Barrouillet, P. ( 1997 ). Stratégies de lecture et résolution de problèmes arithmétiques. L’Année Psychologique, 97, 9–31.
Fayol, M. , Abdi, H. , & Gombert, J.E. ( 1987 ). Arithmetic problem formulation and working memory load. Cognition and Instruction, 4, 183–202.
Fayol, M. , Barrouillet, P. , & Camos, V. ( 1997 ). Early mathematics learning: what can research tell us, Report for the DG 22 of the European Community, Brussels. Brussels: EU.
Fuchs, L.S. , Fuchs, D. , Compton, D.L. , Powell, S.R. , Seethaler, P.M. , Capizzi, A.M. , Schatschneider, C. , & Fletcher, J.M. ( 2006 ). The cognitive correlates of third-grade skill in arithmetic, algorithmic computation, and arithmetic word problems. Journal of Educational Psychology, 98, 29–43.
Fuson, K.C. , Carroll, W.M. , & Landis, J. ( 1996 ). Levels in conceptualizing and solving addition and subtraction compare word problems. Cognition and Instruction, 14, 345–371.
Garcia, A.I. , Jimenez, J.E. , & Hess, S. ( 2006 ). Solving arithmetic word problems: an analysis of classification as a function of difficulty in children with and without arithmetic LD. Journal of Learning Disabilities, 39, 270–281.
Geary, D.C. ( 1993 ). Mathematical disabilities: cognitive, neuropsychological, and genetic components. Psychological Bulletin, 114, 345–362.
Greer, B. ( 1992 ). Multiplication and division as models of situations. In D. A. Grouws (Ed.) Handbook of Research in Mathematics Teaching and Learning (pp. 276–295). New York: Macmillan.
Hudson, T. ( 1983 ). Correspondences and numerical differences between disjoint set. Child Development, 54, 84–90.
Ibarra, C. , & Lindvall, C. ( 1982 ). Factors associated with the ability of kindergarten children to solve simple arithmetic story problems. Journal of Educational Research, 75, 149–155.
Jitendra, A, K. , Griffin, C. C. , Deatline-Buchman, A. , & Sczesniak, E. ( 2007 ). Mathematical word problem solving in third-grade classrooms. Journal of Educational Research, 100, 283–302.
Jitendra, A.K. , & Hoff, K. ( 1996 ). The effects of schema-based instruction on the mathematical word-problem solving performance of students with learning disabilities. Journal of Learning Disabilities, 29, 422–431.
Jitendra, A.K. , & Star, J.R. ( 2011 ). Meeting the needs of students with learning disabilities in inclusive mathematics classrooms: the role of schema-based instruction on mathematical problem-solving. Theory into Practice, 50, 12–19.
Johnson-Laird, P.N. ( 1983 ). Mental Models: Towards a Cognitive Science of Language, Inference, and Consciousness. Cambridge, MA: Harvard University Press/Cambridge: Harvard University Press.
Jordan, N.C. , & Hanish, L.B. ( 2000 ). Mathematical thinking in second-grade children with different forms of LD. Journal of Learning Disabilities, 33, 567–578.
Jordan, N.C. , Kaplan, D. , & Hanish, L.B. ( 2002 ). Achievement growth in children with learning difficulties in mathematics: findings of a two-year longitudinal study. Journal of Educational Psychology, 94, 586–597.
Jordan, N.C. , & Montani, T.O. ( 1997 ). Cognitive arithmetic and problem solving: a comparison of children with specific and general mathematics difficulties. Journal of Learning Disabilities, 30, 624–634.
Judd, T.P. , & Bilsky, L.H. ( 1989 ). Comprehension and memory in the solution of verbal arithmetic problems by mentally retarded and nonretarded individuals. Journal of Educational Psychology, 81, 541–546.
Kail, R.V. ( 2007 ). Longitudinal evidence that increases in processing speed and working memory enhance children’s reasoning. Psychological Science, 18, 312–313.
Kail, R. , & Hall, L.K. ( 1999 ). Sources of developmental change in children’s word problem performance. Journal of Educational Psychology, 91, 660–668.
Kintsch, W. , & Greeno, J.G. ( 1985 ). Understanding and solving word arithmetic problems. Psychological Review, 92, 109–129.
Landy, D. , & Goldstone, R.L. ( 2007 ). How abstract is symbolic thought? Journal of Experimental Psychology: Learning, Memory and Cognition, 33(4), 720–733.
Lee, K. , Ng, S.F. , & Ng, E.L. ( 2009 ). The contributions of working memory and executive functioning to problem representation and solution generation in algebraic word problems. Journal of Educational Psychology, 101, 373–387.
Lee, K. , Ng, S.F. , Ng, E.L. , & Lim, Z.Y. ( 2004 ). Working memory and literacy as predictors of performance on algebraic word problem. Journal of Experimental Child Psychology, 89, 140–158.
LeFevre, J. , DeStefano, D. , Coleman, B. , & Shanahan, T. ( 2005 ). Mathematical cognition and working memory. In J. I. D. Campbell (Ed.), Handbook of Mathematical Cognition (pp. 361–377). New York: Psychology Press.
Lerkkanen, M.-K. , Rasku-Puttonen, H. , Aunola, K. , & Nurmi, J-E. ( 2005 ). Mathematical performance predicts progress in reading comprehension among 7-year olds. European Journal of the Psychology of Education, 20, 121–137.
Lewis, A. , & Mayer, R.E. ( 1987 ). Students’ miscomprehension of relational statements in arithmetic word problems. Journal of Educational Psychology, 79, 363–371.
Lindvall, C.M. , & Ibarra, C.G. ( 1980 ). Incorrect procedure used by primary grade pupils in solving open addition and subtraction sentences. Journal for Research in Mathematics Education, 11, 50–62.
Mani, K. , & Johnson-Laird, P.N. ( 1982 ). The mental representation of spatial description. Memory & Cognition, 10, 181–187.
Nesher, P. ( 1981 ). Levels of description in the analysis of addition and subtraction. In T. P. Carpenter , J. M. Moser , & T. A. Romberg (Eds), Addition and Subtraction: Developmental Perspective (pp. 25–38). Hillsdale, NJ: Lawrence Erlbaum.
Nesher, P. , Greeno, J.G. , & Riley, M.S. ( 1982 ). The development of semantics categories for addition and subtraction. Educational Studies in Mathematics, 13, 373–394.
Oakhill, J. ( 1996 ). Mental models in children’s text comprehension. In J. Oakhill & A. Garnham (Eds), Mental Models in Cognitive Science (pp. 77–94). Hove: Psychology Press.
Oakhill, J. , Cain, K. , & Yuill, N. ( 1998 ). Individual differences in children’s comprehension skill: Toward an integrated model. In C. Hulme & R. M. Joshi (Eds), Reading and Spelling: Development and Disorders (pp. 343–367). Mahwah, NJ: Lawrence Erlbaum Associates, Publishers.
Passolunghi, M.C. , Cornoldi, C. , & De Liberto, S. ( 1999 ). Working memory and intrusions of irrelevant information in a group of specific poor problem solvers. Memory & Cognition, 27, 779–790.
Passolunghi, M.C. , & Mammarella, I.C. ( 2010 ). Spatial working memory impairments in children with difficulties in arithmetic word problem-solving. European Journal of Cognitive Psychology, 22, 944–963.
Passolunghi, M.C. , & Pazzaglia, F. ( 2004 ). Individual differences in memory updating in relation to arithmetic problem solving. Learning and Individual Differences, 14, 219–230.
Passolunghi, M.C. , & Siegel, L.S. ( 2001 ). Short-term memory, working memory, and inhibitory control in children with difficulties in arithmetic problem solving. Journal of Experimental Child Psychology, 80, 44–57.
Pinperton, H. , & Nation, K. ( 2010 ). Understanding words, understanding numbers: an exploration of the mathematical profiles of poor comprehenders. British Journal of Educational Psychology, 80, 255–268.
Rasmussen, C. , & Bisanz, J. ( 2005 ). Representation and working memory in early arithmetic. Journal of Experimental Child Psychology, 91, 137–157.
Rawson, K.A. , & Kintsch, W. ( 2002 ). How does background information improve memory for test content? Memory & Cognition, 30 (5), 768–778.
Reusser, K. ( 1989 ). Textual and Situational Factors in Solving Mathematical Word Problems. Bern: University of Bern.
Riley, M.S. , Greeno, J.G. , & Heller, J.I. ( 1983 ). Development of children’s problem solving ability in arithmetic. In H. P. Ginsburg (Ed.), The Development of Mathematical Thinking. New York: Academic Press.
Siegler, R.S. ( 1996 ). Emerging Minds: The Process of Change in Children’s Thinking. Oxford: Oxford University Press.
Sprinthall, R.C. , & Nolan, T.E. ( 1991 ). Efficacy of representing quantities with different pictures in solving arithmetic word problems. Perceptual and Motor Skills, 72, 274–274.
Staub, F.C. , & Reusser, K. ( 1995 ). The role of presentational structures in understanding and solving mathematical word problems. In C. A. Weaver , S. Mannes , & C. R. Fletcher (Eds), Discourse Comprehension. Essays in Honor of Walter Kintsch (pp. 285–305). Hillsdale, NJ: Lawrence Erlbaum.
Stellingwerf, B.P. , & Van Lieshout, E.C.D.M. ( 1999 ). Manipulatives and number sentences in computer aided arithmetic word problem solving. Instructional Science, 27, 459–476.
Stern, E. , & Lehrndorfer, A. ( 1992 ). The role of situational context in solving word problems. Cognitive Development, 2, 259–268.
Swanson, H.L. ( 2011 ). Working memory, attention, and mathematical problem solving: a longitudinal study of elementary school children. Journal of Educational Psychology, 103, 821–837.
Swanson, H.L. , Cooney, J.B. , & Brock, S. ( 1993 ). The influence of working memory and classification ability on children’s word problem solution. Journal of Experimental Child Psychology, 55, 374–395.
Thevenot, C. ( 2010 ). Arithmetic word problem solving: evidence for the construction of a mental model. Acta Psychologica, 133, 90–95.
Thevenot, C. , Barrouillet, P. , & Fayol, M. ( 2004 ). Mental representation and procedures in arithmetic word problems: The effect of the position of the question. L’Année Psychologique, 104 (4), 683–699.
Thevenot, C. , Devidal, M. , Barrouillet, P. , & Fayol, M. ( 2007 ). Why does placing the question before an arithmetic word problem improve performance? A situation model account. Quarterly Journal of Experimental Psychology, 60, 43–56.
Thevenot, C. , & Oakhill, J. ( 2005 ). The strategic use of alternative representation in arithmetic word problem solving. Quarterly Journal of Experimental Psychology – A, 58, 1311–1323.
Verschaffel, L. , De Corte, E. , & De Win, L. ( 1985 ). Influence of rewording verbal problems on children’s problem representations and solutions. Journal of Educational Psychology, 77, 460–470.
Vergnaud, G. ( 1982 ). A classification of cognitive tasks and operations of thought involved in addition and subtraction problems. In T. P. Carpenter , J. M. Moser , & T. A Romberg (Eds), Addition and Subtraction: A Cognitive Perspective (pp. 39–59). Hillsdale: Erlbaum.
Vergnaud, G. ( 1983 ). Multiplicative structures. In R. Lesh , & M. Landau (Eds), Acquisition of Mathematic Concepts and Processes. New York: Academic Press.
Vicente, S. , Orrantia, J. , & Verschaffel, L. ( 2007 ). Influence of situational and conceptual rewording on word problem solving. British Journal of Educational Psychology, 77, 829–848.
Vicente, S. , Orrantia, J. , & Verschaffel, L. ( 2008 ). Influence of mathematical and situational knowledge on arithmetic word problem solving: textual and graphical aids. Infancia y Aprendizare, 31, 463–483.
Vukovic, R.K. , Lesaux, N.K. , & Siegel, L.S. ( 2010 ). The mathematics skills of children with reading difficulties. Learning and Individual Differences, 20, 639–643.
Willis, G.B. , & Fuson, K.C. ( 1988 ). Teaching children to use schematic drawings to solve addition and subtraction word problems. Journal of Educational Psychology, 80, 192–201.
Yuill, N. , Oakhill, J. , & Parkin, A. ( 1989 ). Working memory, comprehension ability and the resolution of text anomaly. British Journal of Psychology, 80, 351–61.
Zheng, X. , Swanson, H.L. , & Marcoulides, G.A. ( 2011 ). Working memory components as predictors of children’s mathematical word problem solving. Journal of Experimental Child Psychology, 110, 481–498.
- About Oxford Academic
- Publish journals with us
- University press partners
- What we publish
- New features
- Open access
- Institutional account management
- Rights and permissions
- Get help with access
- Accessibility
- Advertising
- Media enquiries
- Oxford University Press
- Oxford Languages
- University of Oxford
Oxford University Press is a department of the University of Oxford. It furthers the University's objective of excellence in research, scholarship, and education by publishing worldwide
- Copyright © 2024 Oxford University Press
- Cookie settings
- Cookie policy
- Privacy policy
- Legal notice
This Feature Is Available To Subscribers Only
Sign In or Create an Account
This PDF is available to Subscribers Only
For full access to this pdf, sign in to an existing account, or purchase an annual subscription.

Drawings of mathematical problems predict their resolution
Solving arithmetic problems, even simple subtractions, involves mental representations whose influence remains to be clarified. Visualizing these representations would enable us to better understand our reasoning and adapt our teaching methods. A team from the University of Geneva (UNIGE), in collaboration with CY Cergy Paris University (CYU) and University of Burgundy (uB), analyzed drawings made by children and adults when solving simple problems. The scientists found that, whatever the age of the participant, the most effective calculation strategies were associated with certain drawing typologies. These results, published in the journal Memory & Cognition , open up new perspectives for the teaching of mathematics.
Learning mathematics often involves small problems, linked to concrete everyday situations. For example, pupils have to add up quantities of flour to make a recipe or subtract sums of money to find out what's left in their wallets after shopping. They are thus led to translate statements into algorithmic procedures to find the solution. This translation of words into solving strategies involves a stage of mental representation of mathematical information, such as numbers or the arithmetic operation to be performed, and non-mathematical information, such as the context of the problem.
The cardinal or ordinal dimensions of problems
Having a clearer idea of these mental representations would enable a better understanding of the choice of calculation strategies. Scientists from UNIGE, CYU and uB conducted a study with 10-year-old children and adults, asking them to solve simple problems with the instruction to use as few calculation steps as possible. The participants were then asked to produce a drawing or diagram explaining their problem-solving strategy for each statement. The contexts of some problems called on the cardinal properties of numbers -- the quantity of elements in a set -- others on their ordinal properties -- their position in an ordered list.
The former involved marbles, fishes, or books, for example: ''Paul has 8 red marbles. He also has blue marbles. In total, Paul has 11 marbles. Jolene has as many blue marbles as Paul, and some green marbles. She has 2 green marbles less than Paul has red marbles. In total, how many marbles does Jolene have?''. The latter involved lengths or durations, for example: ''Sofia traveled for 8 hours. Her trip started during the day. Sofia arrived at 11. Fred leaves at the same time as Sofia. Fred's trip lasted 2 hours less than Sofia's. What time was it when Fred arrived?"
Both of the above problems share the same mathematical structure, and both can be solved by a long strategy in 3 steps: 11 -- 8 = 3; 8 -- 2 = 6; 6 + 3 = 9, but also in a single calculation: 11 -- 2 = 9, using a simple subtraction. However, the mental representations of these problems are very different, and the researchers wanted to determine whether the type of representations could predict the calculation strategy, in 1 or 3 steps, of those who solve them.
''Our hypothesis was that cardinal problems -- such as the one involving marbles -- would inspire cardinal drawings, i.e. diagrams with identical individual elements, such as crosses or circles, or with overlaps of elements in sets or subsets. Similarly, we assumed that ordinal problems -- such as the one mentioning travel times -- would lead to ordinal representations, i.e. diagrams with axes, graduations or intervals -- and that these ordinal drawings would reflect participants' representations and indicate that they would be more successful in identifying the one-step solution strategy,'' explains Hippolyte Gros, former post-doctoral fellow at UNIGE's Faculty of Psychology and Educational Sciences, associate professor at CYU, and first author of the study.
Identifying mental representations through drawings
These hypotheses were validated by analyzing the drawings of 52 adults and 59 children. ''We have shown that, irrespective of their experience -- since the same results were obtained in both children and adults -- the use of strategies by the participants depends on their representation of the problem, and that this is influenced by the non-mathematical information contained in the problem statement, as revealed by their drawings,'' says Emmanuel Sander, full professor at the UNIGE's Faculty of Psychology and Educational Sciences. ''Our study also shows that, even after years of experience in solving addition and subtraction, the difference between cardinal and ordinal problems remains very marked. The majority of participants were only able to solve problems of the second type in a single step''.
Improving mathematical learning through drawing analysis
The team also noted that drawings showing ordinal representations were more frequently associated with a one-step solution, even if the problem was cardinal. In other words, drawing with a scale or an axis is linked to the choice of the fastest calculation. "From a pedagogical point of view, this suggests that the presence of specific features in a student's drawing may or may not indicate that his or her representation of the problem is the most efficient one for meeting the instructions -- in this case, solving with the fewest calculations possible," observes Jean-Pierre Thibaut, full professor at the uB Laboratory for Research on Learning and Development.
''Thus, when it comes to subtracting individual elements, a representation via an axis -- rather than via subsets -- is more effective in finding the fastest method. Analysis of students' drawings in arithmetic can therefore enable targeted intervention to help them translate problems into more optimal representations. One way of doing this is to work on the graphical representation of statements in class, to help students understand the most direct strategies,'' concludes Hippolyte Gros.
- Educational Technology
- Mathematics
- Quantum Computers
- Mathematical Modeling
- Computer Modeling
- Computers and Internet
- Math Puzzles
- Algebraic geometry
- Graph drawing
- Scientific visualization
- Computer and video game genres
- Supercomputer
- Chaos theory
- Knot theory
Story Source:
Materials provided by Université de Genève . Note: Content may be edited for style and length.
Journal Reference :
- Hippolyte Gros, Jean-Pierre Thibaut, Emmanuel Sander. Uncovering the interplay between drawings, mental representations, and arithmetic problem-solving strategies in children and adults . Memory & Cognition , 2024; DOI: 10.3758/s13421-024-01523-w
Cite This Page :
Explore More
- Climate Change and Mercury Through the Eons
- Iconic Horsehead Nebula
- Sustainable Jet Fuel from Landfill Emissions
- Bacterial Spores in Bioplastic Make It 'Green'
- Genetic Signals Linked to Blood Pressure
- Double-Fangs of Adolescence Saber-Toothed Cats
- Microarray Patches for Vaccinating Children
- Virus to Save Billions of Gallons of Wastewater
- Weather Report On Planet 280 Light-Years Away
- Trotting Robots and Animal Gait Transitions
Trending Topics
Strange & offbeat.

- The Publisher
- Conferences
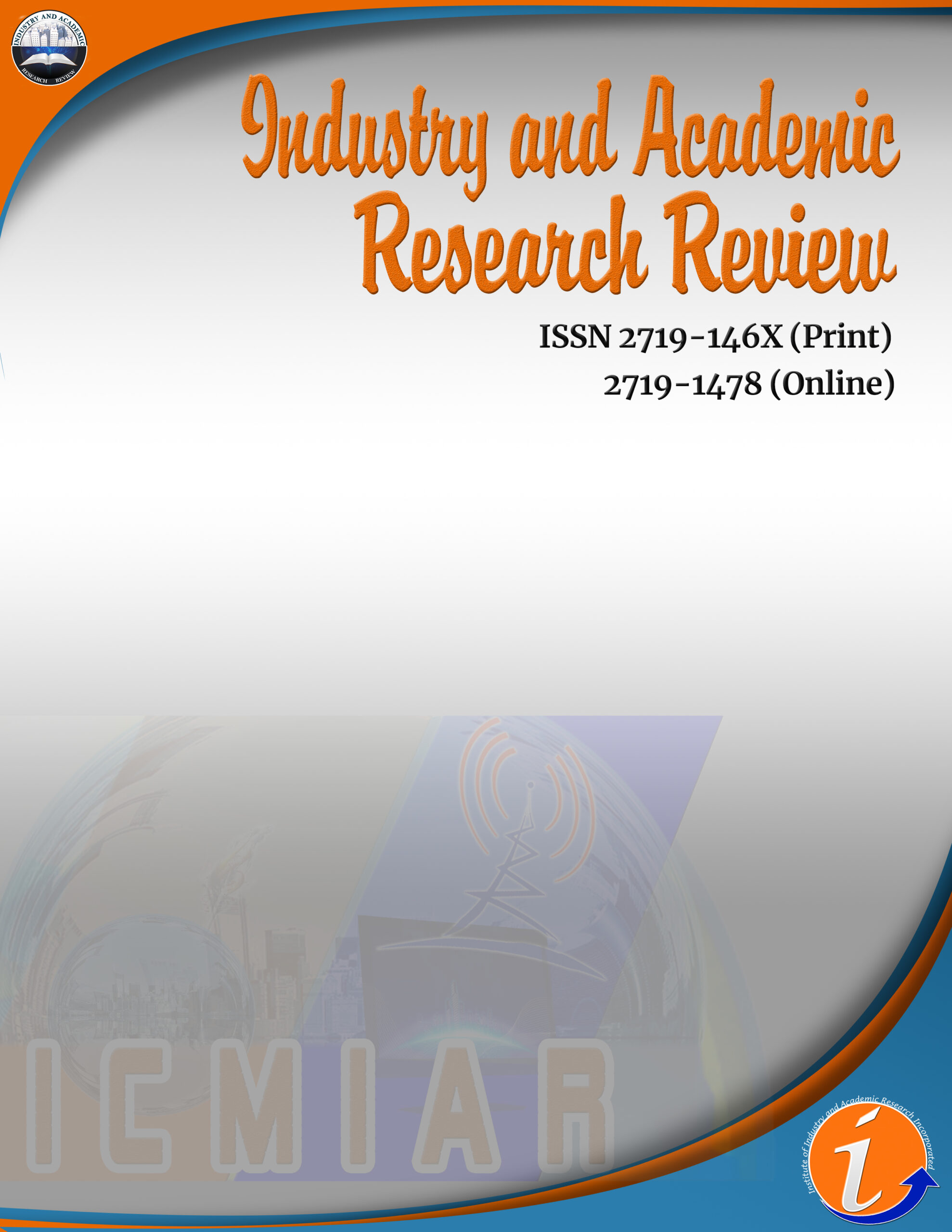
Industry & Academic Research Review
ISSN 2719-146X (Print) 2719-1478 (Online)
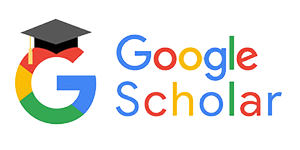
Mental Representation and Critical Thinking in Problem Solving
Monica o. eroles & delon a. ching, december 2021 special issue, presented in first iiari research competitions, december 4, 2021.
- Aims and Scope
- Editorial Board
- Current Issue
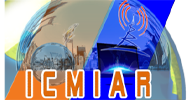
Small Research Grant
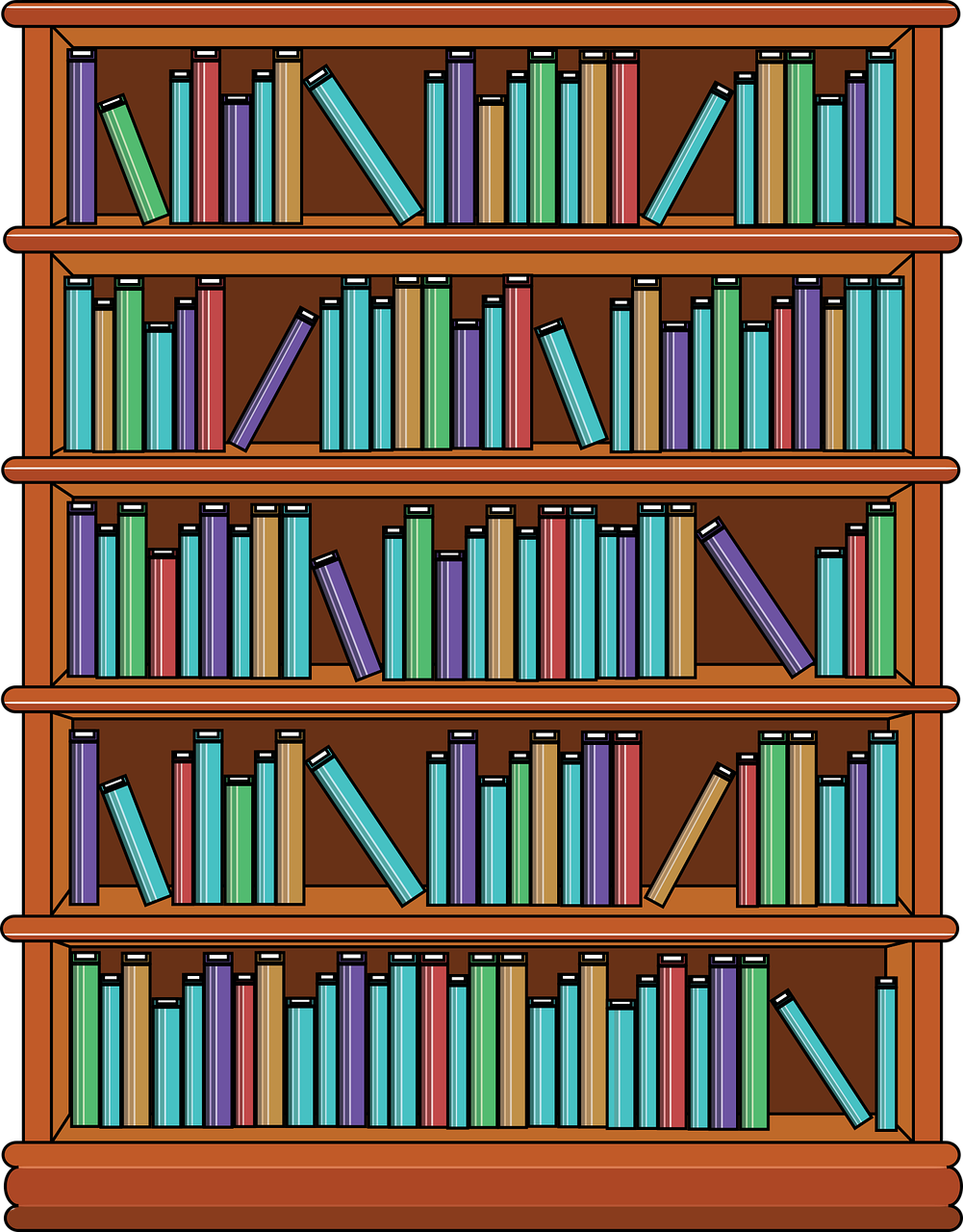
Book Project
Problem solving is the act of defining a problem, identifying the cause of the problem, determining, categorizing, and choosing options for a solution, and applying a solution. Word problems tend to be complex in part due to their illustrative language. Students usually do not recognize what exactly they are being asked, mostly when the problem includes abstract ideas. As a response, this investigation aims to seek the relationship between mental representation and critical thinking in problem solving. The study utilized descriptive and correlational design and focused on describing the assessed extent of Grade VIII students in critical thinking and the perceived extent of students in mental representation. In determining the relationship of mental representation to critical thinking skills of 40 grade VIII students of Cristobal S. Conducto Memorial Integrated National High School in solving word problems. The study applied survey questionnaires and examinations as instruments. The ramification of the study shows that there is a significant relationship among text-based mental representation and critical thinking skills in problem solving, surface components and the two components in critical thinking which is evaluation and inference, and lastly only inference has a significant relationship in situation model. Thus, this study recommends to seek on how mental representation affects critical thinking of one’s student in solving mathematical word problem.
Keywords: Mental Model, Reading Comprehension, Numerical Word Problems, Grade 8 students
Cite this article:
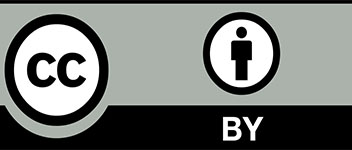
The Research Probe
Proceedings journal for institutional researches.
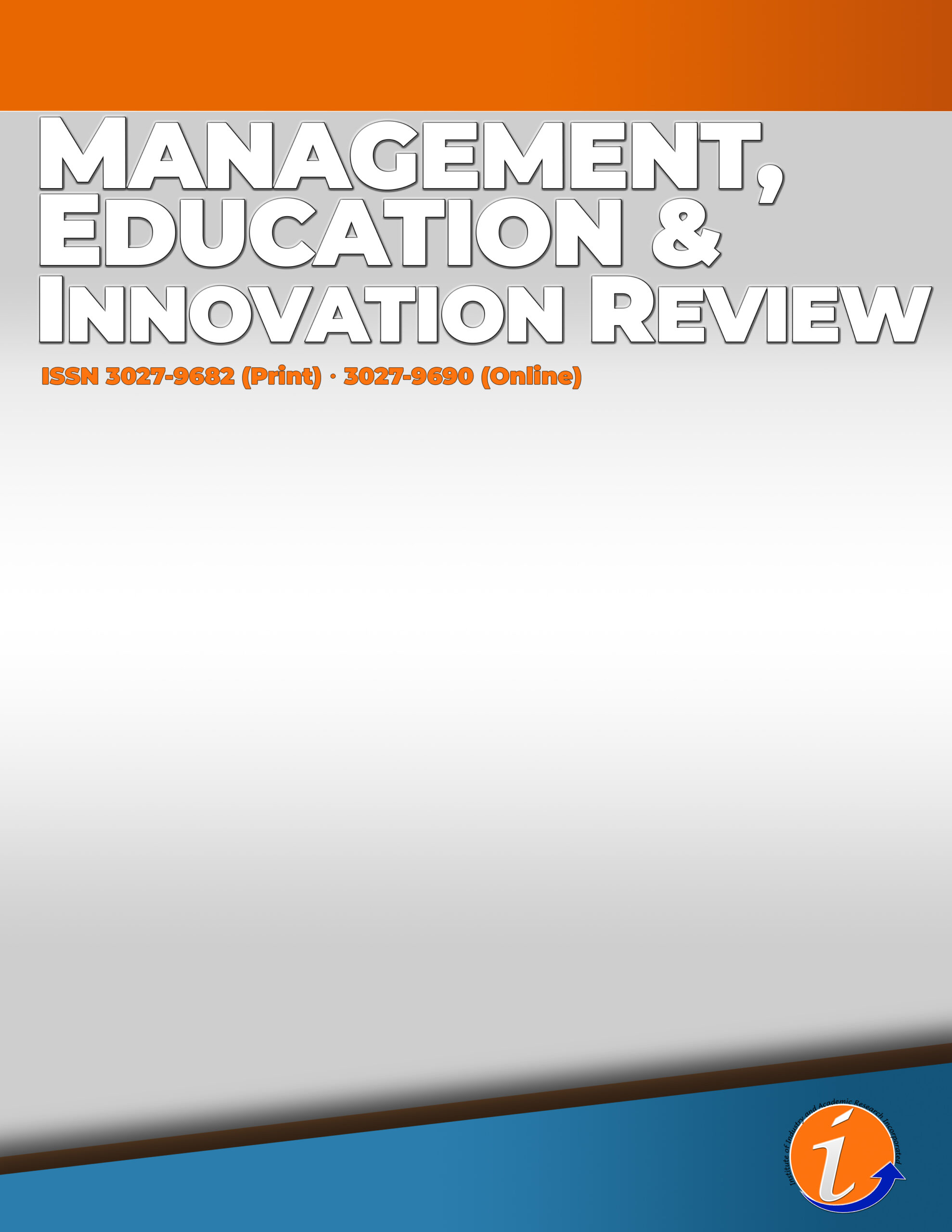
Management, Education & Innovation Review
Proceedings journal for ICMEI.
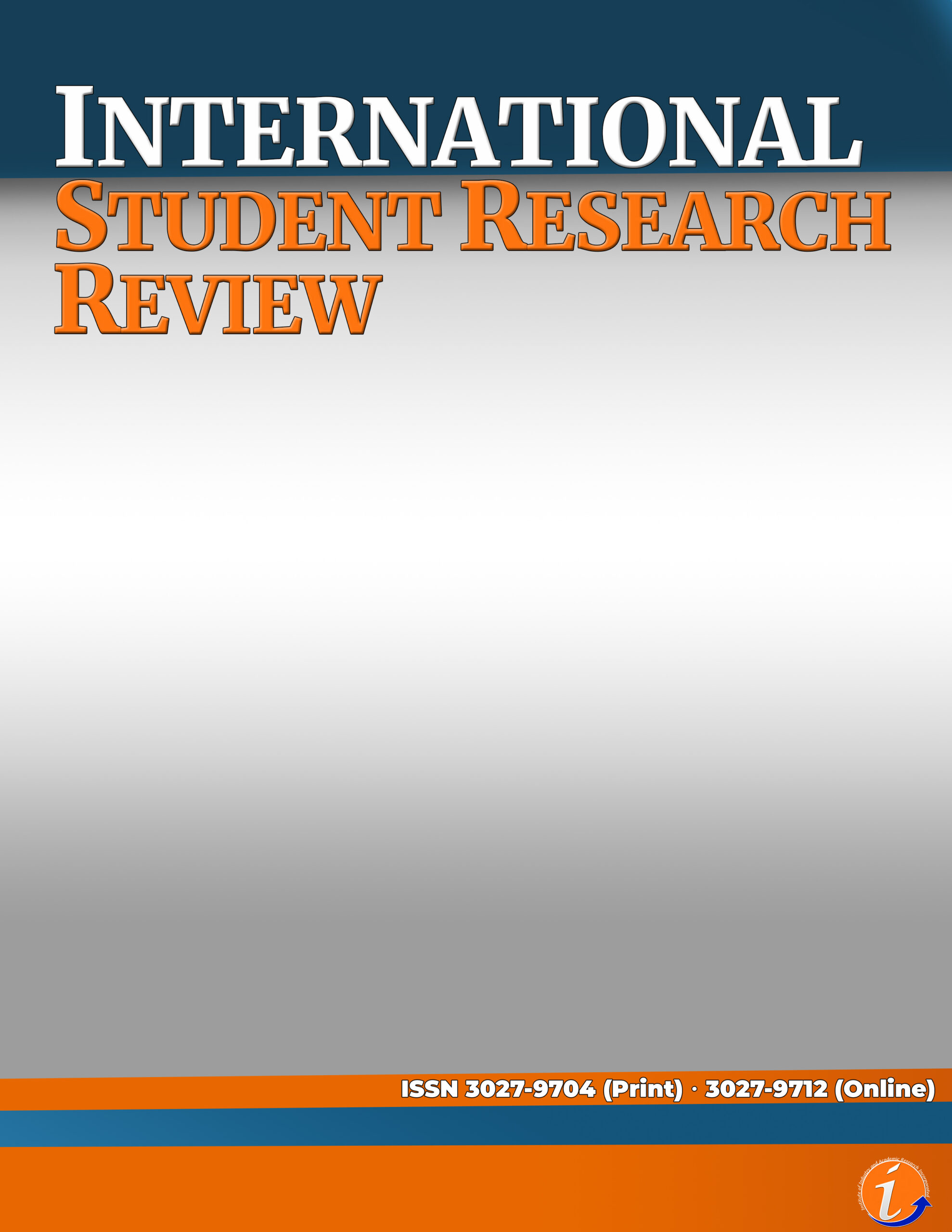
International Student Research Review
Proceedings journal for ISRC.
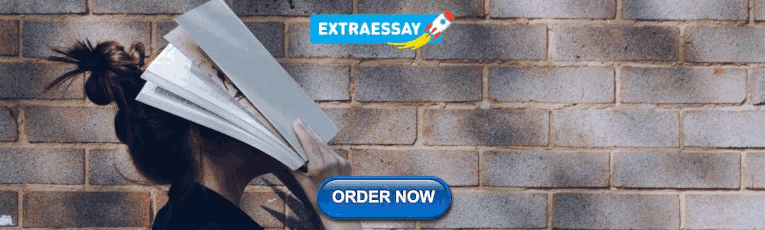
IMAGES
VIDEO
COMMENTS
This study examined the development of mental representations in problem solving situations. The study incorporated a constructivist perspective on learning, including the view that mental ...
Problem solving uses mental models, forms a basis for learning, and can be supported in a variety of ways. Decision making is a more punctuated form of problem solving, made about and with systems. It is not always as clear or accurate as one would like (or expect), and there are ways to support and improve it.
This mental representation of the problem is also known as problem space (Newell and Simon 1972). Second, problem solving often involves retrieving problem schemas from the problem solver's memory to assist the process. A person's problem-solving experiences are accumulated and stored in his or her memories.
solving the problems are a form of external manifestations which are used and related to the Johnson-Laird [41] cognitive framework (shown in Fig. 1) and the theoretical underpinning of the study to infer the kinds of mental representations. Problem solving is a crucial element and is integral in the physics domain or any scientific discipline ...
Abstract. It is widely accepted that mental representations (e.g., mental models and other internal cognitive structures) play a key role in the development of knowledge and expertise. This is especially true in problem-solving domains that involve many interacting components (e.g., complex dynamic systems). However, mental representations are ...
Problem solving does not usually begin with a clear statement of the problem; rather, most problems must be identified in the environment; then they must be defined and represented mentally. The focus of this chapter is on these early stages of problem solving: problem recognition, problem definition, and problem representation. The authors describe the steps and mental processes that ...
The notion of a "mental representation" is, arguably, in the first instance a theoretical construct of cognitive science. As such, it is a basic concept of the Computational Theory of Mind, according to which cognitive states and processes are constituted by the occurrence, transformation and storage (in the mind/brain) of information-bearing structures (representations) of one kind or ...
The study considered representations as conceptual organizations of actions, built up by solvers in problem solving situations, and serving as interpretive tools of understanding to aid their solution activity. Hence, the study focused on the cognitive activity of the learner with particular emphasis on the ways that they elaborate, re-organize ...
Summary. This chapter elaborates what you learned in Chapter 7. It points out that despite the fact that many problems have an obvious visual representation, we need to be able to incorporate more abstract cognitive representations in our theory of problem solving. Traditionally, multidimensional scaling (MDS) has been used to infer Euclidean ...
In this paper, we report on a project concerned with the role of cognition during problem solving. We specifically explore the categories of mental representations that students work with during problem solving of different representational task formats. The sample, consisting of 19 engineering students taking a calculus-based physics course, attempted tasks from the topics of kinematics and ...
A schema can be defined as a set of linked mental representations of the world, which we use both to understand and to respond to situations. The assumption is that we store these mental representations and apply them when needed. ... Problem Solving: Provide complex problems and have students work on solutions, integrating various subjects and ...
Observing changes in solvers' mental problem representation is a methodological and statistical challenge, which is addressed in the present work. Among the reasons for this lack of clarity is that many measures of insight rely solely on the solvers' subjective experience of the solution process. ... Investigating the effect of mental set ...
The proposed models for how word problems are solved disagree on the origin of the internal problem model, i.e., whether the internal problem representation is a result of a schema or a situation ...
Every cognitive enterprise involves some form of knowledge representation. Humans represent information about the external world and internal mental states, like beliefs and desires, and use this information to meet goals (e.g., classification or problem solving). Unfortunately, researchers do not have direct access to mental representations.
Some theories assume that problem solving relies on the instantiation of schemas abstracted from recurrently encountered problems of the same relational structure, whereas other theories propose that ad hoc transient mental representations are constructed for each problem encountered.
problem solvers is the number and kinds of representations they bring to the problem. Introduction to Research on Problem Solving in Chemistry For over 15 years, we have been interested in bridging the gap between theory and practice within the domain of problem solving in chemistry; a gap that results from
Uncovering the interplay between drawings, mental representations, and arithmetic problem-solving strategies in children and adults. Memory & Cognition , 2024; DOI: 10.3758/s13421-024-01523-w Cite ...
process because the mental model is an internal representation involving the recall and processing of memory-owned information that aims at solving the problem. Educators must be able to know the student's mental model for learning to be more optimal. Keywords: Mental Model, Problem-Solving, Internal Representation, Mathematics learning
The ramification of the study shows that there is a significant relationship among text-based mental representation and critical thinking skills in problem solving, surface components and the two components in critical thinking which is evaluation and inference, and lastly only inference has a significant relationship in situation model. Thus ...
Abstract. Uncovering the interplay between drawings, mental representations, and arithmetic problem-solving strategies in children and adults. There is an ongoing debate in the scientific community regarding the nature and role of the mental representations involved in solving arithmetic word problems. In this study, we took a closer look at ...
There is an ongoing debate in the scientific community regarding the nature and role of the mental representations involved in solving arithmetic word problems.
Problem solving is the act of defining a problem, identifying the cause of the problem, determining, categorizing, and choosing options for a solution, and applying a solution. ... In determining the relationship of mental representation to critical thinking skills of 40 grade VIII students of Cristobal S. Conducto Memorial Integrated National ...
In insight problem-solving, the cognitive processes that help you solve a problem happen outside your conscious awareness. 4. Working backward. Working backward is a problem-solving approach often ...